A potential role of homeobox transcription factors in osteoarthritis
Hox genes in adult tissues
The highly conserved Homeobox (abbreviations: Hox for general reference; HOX specifically for the human species) transcription factors play an unquestioned crucial role as master regulators during embryonic vertebrate development and morphogenesis by controlling the three dimensional body plan organisation (1,2). In addition to these known functions in embryonic models, more recently an important role has been ascribed to these transcription factors during remodelling and homeostasis of adult tissues (3,4). By regulating their downstream targets (e.g., matrix-degrading proteinases, extracellular matrix proteins or adhesion molecules), Hox gene products were shown to influence physiological tissue remodelling processes e.g., during wound healing, mainly by coordinating angiogenesis (5). Due to their regulatory functions on tissue synthesis and degradation, it is not surprising that Hox genes have also been identified to be differentially expressed in several diseases, such as atherosclerosis (6), diabetes (7) or cancer (8,9), reflecting pathophysiological conditions characterized by an aberrant metabolism and concomitant with locally atypical vascularization.
Osteoarthritis (OA) pathogenesis and need of early markers
Disrupted metabolism and enhanced vascularisation have been described to be hallmarks of OA. Being the most prevalent degenerative joint disorder leading to pain and disability of millions of people worldwide, OA has become a major burden for patients and healthcare institutions. In the etiology of OA, several key factors have been identified, including mechanical stress (caused mainly by overload, malalignment or obesity), previous joint injuries and aging (10). Being in the focus of research since decades, substantial efforts have been undertaken to unravel mechanisms leading to degradation of the cartilage matrix and ultimately resulting in abnormal remodelling of the affected joint (11). It seems that complex events resembling endochondral bone formation as seen during embryonic development are initiated in OA, leading to cartilage/joint degeneration (12). But still the mechanisms and factors priming these events, as well as markers associated with the onset of early stages of OA, are far from being comprehensively defined.
Early stages of OA are often not accompanied by pain and thus onset of the disease regularly appears unnoticed. Only when degeneration has progressed to a non-revertible, painful stage and structural changes of the joint are visible by MRI, patients are diagnosed for OA. At this stage, treatment actions are not likely to succeed due to the largely damaged state of the joint. As no disease modifying drugs are available, actions are limited to palliation of symptoms—and in many cases terminated by replacement of the joint with a prosthetic implant. Identification of markers to define a stage prior to the onset of irreversible joint degeneration would thus be crucial to anticipate the window for potential clinical treatment, possibly increasing the likelihood of successful repair of the cartilage.
Hox genes in chondrogenesis and OA
Among the factors known to be important during skeletal development, Hox11 genes (such as Hoxa11 and Hoxd11) are known to regulate chondrocyte differentiation (13,14), whereas genes of the Hox8 group are essential during bone development (15,16). Dysregulation of such Hox genes that are essential during joint formation are consequently believed to be involved in several cartilage/skeletal disorders in adults and might thus lead to OA development with time (17).
The onset of another pathology leading to cartilage degradation, namely rheumatoid arthritis, has also been connected with Hox gene regulation. In particular, the inflammation-induced overexpression of Ezh2 (histone methyl transferase, functioning as the catabolic subunit of polycomb repressor and whose targets include Hox genes), correlating with rheumatoid arthritis, was shown to repress the expression of a number of Hox genes (18,19).
Unpublished data from our research group indicate differential expression of selected HOX genes in articular chondrocytes (AC; n=9) isolated from healthy cartilage as compared to chondrocytes retrieved from osteoarthritic (OA, n=5) joints. Whereas HOXA10 and HOXA13 tended to be lower expressed in OA chondrocytes, HOXC8 and HOXD10 were significantly up-regulated in the pathological tissues (Figure 1) and correlated with an upregulation of more than 100-fold of VEGF in OA chondrocytes (data not shown), as previously also described by others (20). In tumors and wound healing, HOXD10 was shown to have an anti-angiogenic effect via reduction of VEGF expression (21,22). In an osteoarthritic environment, that is characterized by an atypically increased vascularization, unexpected upregulation of HOXD10 could reflect an attempt of cells to react and attenuate blood vessel ingrowth, thus underlining the tissue-specific and context-dependent function of Hox genes (5).
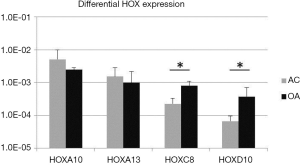
Potential regulation of Hox gene expression in OA by microRNAs (miRNA)
In the search of applicable and predictive markers of early OA, miRNAs have been suggested (23) as they are differentially expressed in OA as compared to healthy joints (24,25). MiRNAs are small non-coding RNAs that function as translational and post-transcriptional regulators of gene expression (26). Interestingly, at least two miRNA families are located within the HOX clusters regulating their expression (27), further indicating a potential connection between OA and HOX gene dysregulation. Those miRNAs located within the HOX gene cluster were also shown to be implicated in Huntington’s disease pathogenesis (28). Moreover, dysregulation of miRNAs has recently been linked to age-related human neurodegenerative diseases (29). Together, these findings indicate a potential role of differentially expressed specific miRNAs in the regulation of HOX genes in OA.
Perspective
Involvement of HOX gene regulation in a wide variety of pathologies and our preliminary results indicate a potential link between OA and HOX transcription factors. Unravelling the mechanisms modulating their differential expression in the development of OA could provide relevant information to better understand this pathology and its initiation. Furthermore HOX genes and/or their upstream regulators should be considered to possibly identify markers of early stages of OA. Defining those early stages and thereby a time window for potential clinical treatment at the beginning of the disease (Figure 2) would open new possibilities in the development of new strategies for treatment of OA before irreversible destruction of the knee joint, and thus diminish the need for total joint replacement by artificial prostheses.
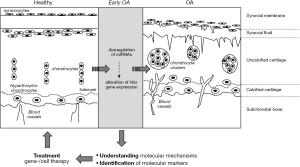
Comprehension of the role of HOX genes in OA could also enhance successful implementation of cell-therapies for its treatment. Some reports have described the use of autologous AC as cell suspension (ACT; autologous chondrocyte transplantation) (30) or assisted with a collagen-based matrix (31) not only for repair of fresh and confined cartilage injuries, but also for the repair of early stage osteoarthritic lesions. The clinical outcome in this more complex setting was inferior in quality and accompanied by a higher failure rate as compared to non-osteoarthritic cartilage defects (30,31). Still, the success rate was acceptable in the reported cases, justifying the treatment of patients with early signs of OA using conventional/matrix assisted ACT in order to avoid or delay prosthetic knee replacement. To improve the quality and success rate of cartilage repair in early osteoarthritic conditions, different cell sources with improved cartilage forming capacities should be considered. Several progenitor-like cell types including mesenchymal stromal cells from different sources (32) and (inducible) pluripotent stem cells (33,34), but also more restricted/differentiated cells originating from other sources of cartilage, such as nasal chondrocytes (35), have been proposed and investigated for their compatibility in cartilage repair. Whether these alternative cell types will also be molecularly and physiologically compatible in a more complex pre-osteoarthritic environment is yet to be investigated and defined. In this context, Hox gene expression could be used to investigate such compatibility and to predict interaction of the implanted cells/tissue with resident cells (35).
Hox genes may also represent the target of a gene therapy approach to OA. Hox gene therapy has already been shown to reverse dysfunctional mesenchymal progenitor cells in diabetic mice by gene transfer of Hoxd3 (36) or Hoxa3 (7). This approach of restoring a “healthy” expression level of Hox as master transcription factor(s) should also be considered in the search for potential means to treat OA. Importantly, given that Hox genes typically regulate cell function as a constructed circuit, activation (or de-activation) of different Hox genes in defined patterns may be necessary to instruct a specific cell behaviour, also depending on the targeted cell type and site of repair.
Modulation of HOX genes was identified during aging in mesenchymal stromal/stem cells (37) and in particular HOXB7 was indicated as a master regulator of age related behavioural changes in progenitor cells (38). In fact, elderly people are more susceptible to diseases with known dis-regulation of HOX, such as cancer, diabetes or atherosclerosis—and also OA is typically associated with aging. Future studies investigating HOX gene modulation could therefore generate more fundamental understanding of the onset and development of a variety of age-related, degenerative diseases, and potentially lead to improved therapeutic strategies applicable in different pathological settings.
Acknowledgements
The presented data were generated and financed within a Swiss National Science Foundation granted Marie-Heim-Vögtlin project PMPDP3_151396/1.
Footnote
Conflicts of Interest: The authors have no conflicts of interest to declare.
References
- Kmita M, Duboule D. Organizing axes in time and space; 25 years of colinear tinkering. Science 2003;301:331-3. [PubMed]
- Krumlauf R. Hox genes in vertebrate development. Cell 1994;78:191-201. [PubMed]
- Rinn JL, Wang JK, Allen N, et al. A dermal HOX transcriptional program regulates site-specific epidermal fate. Genes Dev 2008;22:303-7. [PubMed]
- Mahdipour E, Mace KA. Hox transcription factor regulation of adult bone-marrow-derived cell behaviour during tissue repair and regeneration. Expert Opin Biol Ther 2011;11:1079-90. [PubMed]
- Kachgal S, Mace KA, Boudreau NJ. The dual roles of homeobox genes in vascularization and wound healing. Cell Adh Migr 2012;6:457-70. [PubMed]
- Cantile M, Schiavo G, Terracciano L, et al. Homeobox genes in normal and abnormal vasculogenesis. Nutr Metab Cardiovasc Dis 2008;18:651-8. [PubMed]
- Mace KA, Hansen SL, Myers C, et al. HOXA3 induces cell migration in endothelial and epithelial cells promoting angiogenesis and wound repair. J Cell Sci 2005;118:2567-77. [PubMed]
- Bhatlekar S, Fields JZ, Boman BM. HOX genes and their role in the development of human cancers. J Mol Med (Berl) 2014;92:811-23. [PubMed]
- Caré A, Silvani A, Meccia E, et al. HOXB7 constitutively activates basic fibroblast growth factor in melanomas. Mol Cell Biol 1996;16:4842-51. [PubMed]
- Buckwalter JA, Martin JA. Osteoarthritis. Adv Drug Deliv Rev 2006;58:150-67. [PubMed]
- Pap T, Korb-Pap A. Cartilage damage in osteoarthritis and rheumatoid arthritis-two unequal siblings. Nat Rev Rheumatol 2015. [Epub ahead of print]. [PubMed]
- Wang M, Shen J, Jin H, et al. Recent progress in understanding molecular mechanisms of cartilage degeneration during osteoarthritis. Ann N Y Acad Sci 2011;1240:61-9. [PubMed]
- González-Martín MC, Mallo M, Ros MA. Long bone development requires a threshold of Hox function. Dev Biol 2014;392:454-65. [PubMed]
- Gross S, Krause Y, Wuelling M, et al. Hoxa11 and Hoxd11 regulate chondrocyte differentiation upstream of Runx2 and Shox2 in mice. PLoS One 2012;7:e43553. [PubMed]
- van den Akker E, Fromental-Ramain C, de Graaff W, et al. Axial skeletal patterning in mice lacking all paralogous group 8 Hox genes. Development 2001;128:1911-21. [PubMed]
- Zheng YJ, Chung HJ, Min H, et al. In vitro osteoblast differentiation is negatively regulated by Hoxc8. Appl Biochem Biotechnol 2010;160:891-900. [PubMed]
- van der Kraan PM. Understanding developmental mechanisms in the context of osteoarthritis. Curr Rheumatol Rep 2013;15:333. [PubMed]
- Schwarz D, Varum S, Zemke M, et al. Ezh2 is required for neural crest-derived cartilage and bone formation. Development 2014;141:867-77. [PubMed]
- Trenkmann M, Brock M, Gay RE, et al. Expression and function of EZH2 in synovial fibroblasts: epigenetic repression of the Wnt inhibitor SFRP1 in rheumatoid arthritis. Ann Rheum Dis 2011;70:1482-8. [PubMed]
- Pufe T, Petersen W, Tillmann B, et al. The splice variants VEGF121 and VEGF189 of the angiogenic peptide vascular endothelial growth factor are expressed in osteoarthritic cartilage. Arthritis Rheum 2001;44:1082-8. [PubMed]
- Chen A, Cuevas I, Kenny PA, et al. Endothelial cell migration and vascular endothelial growth factor expression are the result of loss of breast tissue polarity. Cancer Res 2009;69:6721-9. [PubMed]
- Myers C, Charboneau A, Cheung I, et al. Sustained expression of homeobox D10 inhibits angiogenesis. Am J Pathol 2002;161:2099-109. [PubMed]
- Bernard NJ. Osteoarthritis: circulating miRNAs-early osteoarthritis biomarkers? Nat Rev Rheumatol 2014;10:197. [PubMed]
- Jones SW, Watkins G, Le Good N, et al. The identification of differentially expressed microRNA in osteoarthritic tissue that modulate the production of TNF-alpha and MMP13. Osteoarthritis Cartilage 2009;17:464-72. [PubMed]
- Le LT, Swingler TE, Clark IM. Review: the role of microRNAs in osteoarthritis and chondrogenesis. Arthritis Rheum 2013;65:1963-74. [PubMed]
- Bartel DP. MicroRNAs: genomics, biogenesis, mechanism, and function. Cell 2004;116:281-97. [PubMed]
- Yekta S, Tabin CJ, Bartel DP. MicroRNAs in the Hox network: an apparent link to posterior prevalence. Nat Rev Genet 2008;9:789-96. [PubMed]
- Hoss AG, Kartha VK, Dong X, et al. MicroRNAs located in the Hox gene clusters are implicated in huntington's disease pathogenesis. PLoS Genet 2014;10:e1004188. [PubMed]
- Junn E, Mouradian MM. MicroRNAs in neurodegenerative diseases and their therapeutic potential. Pharmacol Ther 2012;133:142-50. [PubMed]
- Minas T, Gomoll AH, Solhpour S, et al. Autologous chondrocyte implantation for joint preservation in patients with early osteoarthritis. Clin Orthop Relat Res 2010;468:147-57. [PubMed]
- Niemeyer P, Lenz P, Kreuz PC, et al. Chondrocyte-seeded type I/III collagen membrane for autologous chondrocyte transplantation: prospective 2-year results in patients with cartilage defects of the knee joint. Arthroscopy 2010;26:1074-82. [PubMed]
- Bornes TD, Adesida AB, Jomha NM. Mesenchymal stem cells in the treatment of traumatic articular cartilage defects: a comprehensive review. Arthritis Res Ther 2014;16:432. [PubMed]
- Sun N, Panetta NJ, Gupta DM, et al. Feeder-free derivation of induced pluripotent stem cells from adult human adipose stem cells. Proc Natl Acad Sci U S A 2009;106:15720-5. [PubMed]
- Takahashi K, Tanabe K, Ohnuki M, et al. Induction of pluripotent stem cells from adult human fibroblasts by defined factors. Cell 2007;131:861-72. [PubMed]
- Pelttari K, Pippenger B, Mumme M, et al. Adult human neural crest-derived cells for articular cartilage repair. Sci Transl Med 2014;6:251ra119.
- Hansen SL, Myers CA, Charboneau A, et al. HoxD3 accelerates wound healing in diabetic mice. Am J Pathol 2003;163:2421-31. [PubMed]
- Bork S, Pfister S, Witt H, et al. DNA methylation pattern changes upon long-term culture and aging of human mesenchymal stromal cells. Aging Cell 2010;9:54-63. [PubMed]
- Candini O, Spano C, Murgia A, et al. Mesenchymal progenitors aging highlights a miR-196 switch targeting HOXB7 as master regulator of proliferation and osteogenesis. Stem Cells 2015;33:939-50. [PubMed]