Crocin induces autophagic cell death and inhibits cell invasion of cervical cancer SiHa cells through activation of PI3K/AKT
Introduction
Cervical cancer, the most common human papillomavirus (HPV)-associated cancer, is one of the most prevalent tumors among women in the developing world (1). As vaccination is still largely economically unavailable, cervical cancer continues to be a growing major public health issue (2). Once diagnosed, the prognosis of cervical cancer is poor, especially in women with metastatic or recurrent disease. Novel therapeutic approaches including immunotherapy and targeted therapy, for example platinum combined with bevacizumab or pembrolizumab, have been able to extend overall survival (3). However, these treatment approaches remain insufficient and expensive.
Autophagy have critical effects on HPV infection and development of cervical cancer [reviewed in (4)]. HPV inhibited autophagy via inhibiting the fusion of autophagosome-lysosome with multiple ways. Autophagy was inhibited upon infection to avoid digestion, and in later processes to promote proliferation and carcinogenesis of infected cells. Inhibited autophagy could also avoid apoptotic cell death induced with violent autophagy. It is thus very important to restore the autophagic processes in treating cervical cancer.
Saffron, a traditional Chinese medicine, is the dried dark-red stigma of the plant Crocus sativus L. Saffron has been used traditionally to treat some conditions (5), such as certain cancers, neurological defects, cognitive problems and inflammatory diseases. One of the main bioactive components of Saffron is crocin, a soluble carotenoid with two D-gentiobioside glycosyl esters. The mechanism of action of crocin is complex (5), and includes the inhibition of DNA and RNA synthesis, reversion of epithelial-mesenchymal transition (EMT), suppression of cell invasion and metastasis, targeting of cellular topoisomerase and microtubules, induction of apoptosis, suppression of telomerase activity, reduction of oxidative stress, and epigenetic effects. These mechanisms suggest that crocin may have potential as an antitumor agent. Furthermore, emerging evidence suggests that saffron is selectively toxic to tumor cells but safe to normal cells (6,7). Crocin was implied to induce autophagy and promote apoptosis in hepatocellular carcinoma by inhibiting Akt/mTOR activity (8). Crocin has also demonstrated time and dose dependent inhibition of cervical cancer cell viability, and induced apoptosis and cell cycle arrest (7). However, whether or not autophagy and apoptosis could be induced with crocin in cervical cancer still require further investigation.
To this end, the current study tested the effects of crocin on SiHa cells. Viability, apoptosis, autophagy and potential signaling molecules were investigated, and also validated in female BALB/c nude mice. We present the following article in accordance with the ARRIVE reporting checklist (available at http://dx.doi.org/10.21037/atm-20-5882).
Methods
Cell culture and viability
SiHa cells were purchased from the American Type Culture Collection (Rockville, MD, USA), and were maintained at 37 °C with 5% CO2. Cells were cultured with Dulbecco’s modified eagle medium (DMEM) supplemented with 10% fetal bovine serum and 1% penicillin/streptomycin solution. For testing cell viability, the SiHa cells were seeded onto 96-well plates with 3×103 cells/well in 100 µL of DMEM medium supplemented with 0.06, 0.13, 0.25, 0.5, 1, 2, 4, 8 and 16 mM of crocin (#17304, Sigma-Aldrich, Missouri, USA). After 22 hours, 10 µL CCK-8 solution was dissolved in 90 µL of DMEM medium and subsequently added to each well. The plates were incubated for 2 hours, and the absorbance value was measured at 450 nm.
Transwell migration assay
A transwell migration assay was conducted to measure cell invasion. The Matrigel (Becton, Dickinson and Company, Bioscience, San Jose, CA, USA) was pre-cooled at 4 °C overnight for liquidation, and diluted with serum-free medium on ice for a 1:1 dilution. The Matrigel mixture (15 µL) was then added to the upper chamber (Costar, Cambridge, MA, USA) in each well and cultured at 37 °C for 1 hour, then rinsed three times using serum-free medium. SiHa cells (1×105 cells) suspended in DMEM were added to the upper chamber, and 600 µL DMEM complete medium containing 15% FBS was added to the basolateral chamber. To both chambers, 0, 1, 2 or 4 mM of crocin was added, then cultured for 24 hours. The chambers were then fixed with 50% methyl alcohol for 15 minutes. Crystal violet staining was performed, and 6 visual fields from each well were randomly observed and imaged under an inverted microscope (200×, Olympus Optical Co., Ltd.). The average number of cells was calculated in each field.
Flow cytometry
To detect cell apoptosis, SiHa cells were treated with crocin (0, 1, 2 and 4 mM) for 24 hours then digested with trypsin. The trypsin digested cells (1×106) were subjected to flow cytometry using an Annexin V-FITC/PI Apoptosis Detection kit (Beyotime, Shanghai, Chain) following the manufacturer’s protocol. Cells with Annexin V-positive values were considered early apoptotic cells, those with PI-positive values were considered necrotic, and those with double positive values were considered late apoptotic.
Western blotting analysis
Western blotting was performed to measure protein expression. SiHa cells were treated with crocin (0, 1, 2 and 4 mM) for 24 hours then washed twice with cold PBS, and RIPA lysis buffer (Beyotime) was added to collect protein lysates. Total protein concentration was measured using the BCA Protein Assay Reagent (Pierce, Rockford, IL, USA), and the sample concentrations were diluted with PBS. Equal amounts of protein from experimental groups were subjected to SDS-PAGE and transferred to PVDF membranes. The membranes were blocked with 5% fat-free milk, probed with the primary and secondary antibodies, then enhanced with a chemiluminescence (ECL) reagent (Amersham Pharmacia Biotech). The following antibodies (Abcam, Cambridge, UK) were used: anti-Caspase-3 (ab32351), anti-Caspase-9 (ab202068), anti-LC3B (ab48394), anti-Beclin1 (ab210498), anti-ATG-7 (ab52472), anti-AMPK (ab80039), anti-p-AMPK (ab133448), anti-AKT (ab8805), anti-p-AKT (phospho T308, ab38449), anti-mTOR (ab2732), anti-p-mTOR (ab109268), anti-VEGF (ab150375), anti-Actin (ab8227) and goat-anti-rabbit IgG (ab205718).
Immunofluorescence
Immunofluorescence of SiHa cells was performed as previously described (9). After fixation with 4% paraformaldehyde for 15 min at 37 °C, SiHa cells were permeabilized with 0.1% Triton X-100 (Beyotime, China) for 30 min, and blocked with goat serum for 15 min. Cells were incubated with primary LC3 antibody (1 µg/m, Abcam) overnight at 4 °C, and then with AlexaFluor® 647 conjugated secondary antibody (#4414, 1:2,000, CST, USA) at room temperature for 60 min. DAPI (Beyotime) was used to counterstain the cell nuclei. Immunofluorescence images were acquired with confocal microscopy (LSM 510 Meta, Zeiss, Oberkochen, Germany). LC3 puncta were counted from 10 random fields per slide.
Animal experiments
The study was approved by the Medical Ethics Committee of West China Second Hospital, Sichuan University (No. 2014-2020). All animal experiments were approved by the Institutional Animal Care and Use Committee of Sichuan Academy of Medical Sciences & Sichuan Provincial People’s Hospital, and were performed according to institutional guidelines. Twelve 4-week-old female BALB/c nude mice were purchased from Shanghai SLAC Laboratory Animal Co., Ltd. The mice were housed in 2 polypropylene cages at room temperature with a relative humidity of 60 ± 5% and a photoperiod of 12 h/day. Approximately 1×107 SiHa cells suspended in 100 µL of PBS were injected into the right thigh of all mice. After 5 days, the formation of tumors was confirmed, and mice were randomly divided into two groups and administered as: crocin (50 mg/kg/d) or saline (vehicle) by referring to previous research (10). After 4 weeks, the mice were sacrificed. The tumors were surgically removed, fixed in 10% formalin, and subjected to routine histological examination.
Immunohistochemical staining
Caspase-3 and vascular endothelial growth factor (VEGF) protein expression in xenograft tumors was determined by immunohistochemistry (IHC) (11). The primary antibodies used were the same as those used for western blotting. The fixed tumors were embedded in paraffin and 4 µm slices were sectioned. The sections were rehydrated and incubated in citrate buffer for 3 min at 100 °C. The sections were then incubated with primary antibodies against caspase-3 and VEGF overnight at 4 °C. After further incubation with goat anti-rabbit IgG (Boster Biological Technology, Wuhan, China) for 30 min, the sections were stained with diaminobenzidine and photographed under a microscope. Four random and nonoverlapping positively stained microscopic fields at 400× magnification were examined in each section. The protein expression levels in each section were measured with integral optical density values (IOD) (IOD = sum of four discontinuous visual fields/4) measured with Image-Pro Plus 6.0 software (Media Cybernetics Inc., Maryland, USA).
Statistical analysis
All in vitro experiments were repeated three times. Two-tailed unpaired Student’s t-tests and one-way ANOVA (analysis of variance) were used to evaluate the differences between the two groups. GraphPad Prism 6 software (San Diego, CA) was used for the statistical analysis. All quantitative data were presented as mean ± standard error of mean. P<0.05 was considered statistically significant.
Results
Crocin inhibited viability and invasion capacity of SiHa cells
To test the effects of crocin on SiHa cells, cells were incubated with 0.06, 0.13, 0.25, 0.5, 1, 2, 4, 8 and 16 mM of crocin in 100 µL of medium for 24 hours. The viability of SiHa cells were dose-dependently reduced with crocin, with 2, 4, 6 and 8 mM significantly reducing cell viability (Figure 1A). In later experiments, 0, 1, 2 and 4 mM concentrations of crocin were established to show its underlying mechanism. The invasive cells tested with a transwell migration assay showed a significant reduction in the 2 mM group, and even fewer in 4 mM group (Figure 1B,C). The results demonstrate the inhibition of cell viability and invasion of SiHa cells treated with crocin.
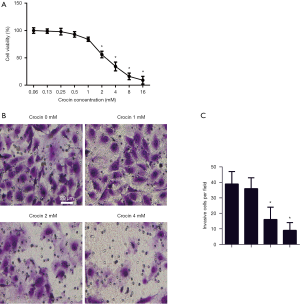
Crocin promoted apoptosis of SiHa cells
Apoptosis is a common downstream mechanism of most anticancer therapeutics. In crocin treated SiHa cells, apoptosis was significantly induced by 2 and 4 mM of crocin (Figure 2A,B). The induced apoptosis was further validated with the increased ratio of cleaved caspase-3/caspase-3 and cleaved caspase-9/caspase-9 (Figure 2C,D). The results indicated that apoptosis was also the downstream mechanism of crocin in the suppression of SiHa cells.
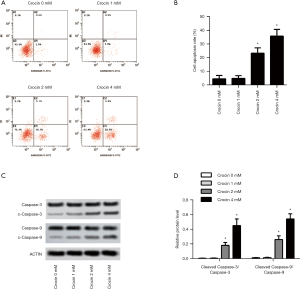
Crocin enhanced autophagy of SiHa cells
The ratio of LC3II/I, Beclin1 and ATG7 were all markedly increased after 2 and 4 mM of crocin treatment (Figure 3A,B). The induced autophagy was also validated by the presence of increased LC3 puncta positive cells after 2 and 4 mM of crocin treatment (Figure 3C,D). These results suggest that autophagy was induced dose-dependently after crocin exposure.
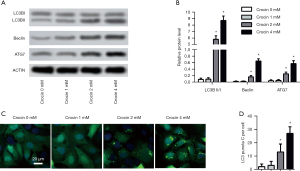
Crocin inactivated AMPK/MTOR
AMPK, AKT and mTOR are implicated in the regulation of autophagy and apoptosis in crocin treated cells (12). As shown in Figure 4A,B, the ratio of p-AMPK/AMPK and p-mTOR/mTOR were inhibited dose-dependently, while p-AKT/AKT increased. These results indicate that AMPK and mTOR signaling were potentially involved in the mechanism of action of crocin in suppressing SiHa cell proliferation.
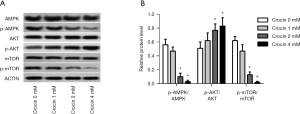
Crocin suppressed tumor progression in vivo
In SiHa cell-bearing mice, 50mg/kg/d of crocin significantly reduced tumor formation (Figure 5A). The tumor volumes in the crocin group were significantly smaller than the control group (Figure 5B). Proliferation and metastases were inhibited with crocin (Figure 5C), as seen through increased caspase-3 (Figure 5D) and reduced VEGF (Figure 5E) in treated tumors. Autophagy in the tumors was assessed using western blotting (Figure 5F), and demonstrated that the ratio of LC3II/I was significantly elevated in tumors of crocin treated mice (Figure 5G).
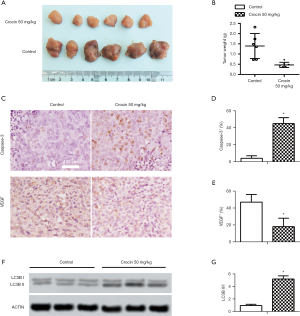
Discussion
Persistent viral infection of the high-risk HPV subtype is the main cause of cervical cancer worldwide. The risk for cervical cancer remains high in certain countries, despite the increasing availability of vaccination (13). Chemotherapy for late stage cervical cancer mainly includes carboplatin/paclitaxel or topotecan/paclitaxel combined with bevacizumab (14,15). Development of novel therapeutic strategies is still required, as current treatment options remain limited. In the present study, we showed that crocin inhibited the progression of cervical cancer in vitro and in vivo, as indicated by the inhibition of proliferation and invasion, and the promotion of autophagy and apoptosis in SiHa cells and in xenograft mice. These effects were at least partly the result of inactivated AMPK/mTOR pathway, supporting the value of autophagy promoting effects in treating cervical cancer. We suggest that crocin is worthy of further study to combat cervical cancer.
Crocin has been implicated in tumor suppression across multiple cancers, through several complex pathways and mechanisms (16). For example, crocin exerts anti-tumor effects through the activation of p53 and Bax while inactivating Bcl-2 in gastric cancer and lung cancer (17,18), inactivating NF-κB and inflammation in colorectal cancer (19), and inhibiting histone deacetylase 2, Wnt/β-catenin and CD34 in breast cancer (20-22). Molecular docking has predicted several targets for crocin, including telomeric DNA (23), coronavirus (nCoV-2019) main protease (24), human tyrosinase (25), human serum albumin (26), glucagon-like peptide-1 (GLP-1) receptor (27), catalase (28), TLR4 and TGF-βR1 (29). Most of these predicted targets have been validated in specific cells. The many predicted targets partly explain the complex mechanism of action for crocin. On the other hand, some of these targets highlight the potential for adverse effects and interactions with other therapeutics that may limit the development and application of crocin, such as raising blood glucose, competing receptors, or changing the in vivo distribution of drugs.
Currently, the mechanism of action for crocin in cervical cancer mainly includes the induction of apoptosis and the inhibition of proliferation (8). Crocin also suppressed spindle microtubule dynamics and reduced the cell viability of HeLa cells without significantly increasing cellular ROS (30,31). In the present study, we also demonstrated a dose-dependent inhibition of cell proliferation. Additionally, invasive cells were reduced after crocin exposure, and apoptosis and autophagy were induced after crocin treatment. These results suggest that autophagy induced apoptosis in crocin treated SiHa cells. The AMPK, AKT and mTOR pathways are implicated in the regulation of autophagy and apoptosis in crocin treated cells in other tumors (12). In the present study, AMPK and mTOR were inactivated while PI3K was activated with crocin treatment. These results suggest that induced autophagy was a result of inhibited AMPK rather than PI3K.
Using a relatively high concentration, Jiang et al. showed that 125 mg/L of crocin inhibited the proliferation of HeLa cells in vitro; and 120 mg/kg of crocin in male albino rats decreased alanine transaminase (ALT), aspartate transaminase (AST), lactate dehydrogenase (LDH), alkaline phosphatase (ALP), blood urea nitrogen (BUN), creatinine, bilirubin, albumin and total protein, while increasing glucose, cholesterol, thyroglobulin (TG), and glutathione (GSH) (32). Based on these results, they argued that the adverse effects of crocin might limit its clinical application. However, crocin has long been utilized as a food colorant and additive in the food industry worldwide, which strongly supports its safety profile (33). On the other hand, less protein/peptides and more glucose or TG in serum may be of benefit in combating tumors, as the protein supply of tumors can be limited and the immune system can be activated. In this study, we observed no significant adverse effects in mice. Crocin administration significantly reduced tumor growth, and promoted autophagy and apoptosis. These results support the efficacy and safety of crocin for cancer treatment.
There are limitations to this study. There are many reported targets of crocin, and the mechanism of action for crocin in cervical cancer is complicated, but only one aspect was investigated in the current study. The complexity of crocin also raises concerns about potential adverse effects and possible interactions with other therapeutics, which remain largely unexplored.
In conclusion, we showed that crocin inhibited proliferation and invasion, and promoted autophagy and apoptosis of SiHa cells and in xenograft mice. We propose that the anti-tumor effects of crocin was a result of induced autophagy through AMPK and mTOR inhibition but not PI3K. Also, the results suggest that crocin could be a potential prodrug for cervical cancer treatment.
Acknowledgments
Funding: None.
Footnote
Reporting Checklist: The authors have completed the ARRIVE reporting checklist. Available at http://dx.doi.org/10.21037/atm-20-5882
Data Sharing Statement: Available at http://dx.doi.org/10.21037/atm-20-5882
Conflicts of Interest: All authors have completed the ICMJE uniform disclosure form (available at http://dx.doi.org/10.21037/atm-20-5882). The authors have no conflicts of interest to declare.
Ethical Statement: The authors are accountable for all aspects of the work in ensuring that questions related to the accuracy or integrity of any part of the work are appropriately investigated and resolved. The study was approved by the Medical Ethics Committee of West China Second Hospital, Sichuan University (No. 2014-2020). All animal experiments were approved by the Institutional Animal Care and Use Committee of Sichuan Academy of Medical Sciences & Sichuan Provincial People’s Hospital, and were performed according to institutional guidelines.
Open Access Statement: This is an Open Access article distributed in accordance with the Creative Commons Attribution-NonCommercial-NoDerivs 4.0 International License (CC BY-NC-ND 4.0), which permits the non-commercial replication and distribution of the article with the strict proviso that no changes or edits are made and the original work is properly cited (including links to both the formal publication through the relevant DOI and the license). See: https://creativecommons.org/licenses/by-nc-nd/4.0/.
References
- Siegel RL, Miller KD, Jemal A. Cancer statistics, 2019. CA Cancer J Clin 2019;69:7-34. [Crossref] [PubMed]
- Canfell K, Kim JJ, Brisson M, et al. Mortality impact of achieving WHO cervical cancer elimination targets: a comparative modelling analysis in 78 low-income and lower-middle-income countries. Lancet 2020;395:591-603. [Crossref] [PubMed]
- Xue C, Zhu D, Chen L, et al. Expression and prognostic value of PD-L1 and PD-L2 in ovarian cancer. Transl Cancer Res 2019;8:111-9. [Crossref]
- Mattoscio D, Medda A, Chiocca S. Human Papilloma Virus and Autophagy. Int J Mol Sci 2018;19. [Crossref] [PubMed]
- Hoshyar R, Mollaei H. A comprehensive review on anticancer mechanisms of the main carotenoid of saffron, crocin. J Pharm Pharmacol 2017;69:1419-27. [Crossref] [PubMed]
- Moradzadeh M, Kalani MR, Avan A. The antileukemic effects of saffron (Crocus sativus L.) and its related molecular targets: A mini review. J Cell Biochem 2019;120:4732-8. [Crossref] [PubMed]
- Mollaei H, Safaralizadeh R, Babaei E, et al. The anti-proliferative and apoptotic effects of crocin on chemosensitive and chemoresistant cervical cancer cells. Biomed Pharmacother 2017;94:307-16. [Crossref] [PubMed]
- Yao C, Liu BB, Qian XD, et al. Crocin induces autophagic apoptosis in hepatocellular carcinoma by inhibiting Akt/mTOR activity. OncoTargets and therapy 2018;11:2017-28. [Crossref] [PubMed]
- Liu S, Wang H, Mu J, et al. MiRNA-211 triggers an autophagy-dependent apoptosis in cervical cancer cells: regulation of Bcl-2. Naunyn Schmiedebergs Arch Pharmacol 2020;393:359-70. [Crossref] [PubMed]
- Rastgoo M, Hosseinzadeh H, Alavizadeh H, et al. Antitumor activity of PEGylated nanoliposomes containing crocin in mice bearing C26 colon carcinoma. Planta Med 2013;79:447-51. [Crossref] [PubMed]
- Zhang X, Zhang X, Dang Z, et al. Cognitive Protective Mechanism of Crocin Pretreatment in Rat Submitted to Acute High-Altitude Hypoxia Exposure. Biomed Res Int 2020;2020:3409679. [PubMed]
- Zeng C, Li H, Fan Z, et al. Crocin-Elicited Autophagy Rescues Myocardial Ischemia/Reperfusion Injury via Paradoxical Mechanisms. Am J Chin Med 2016;44:515-30. [Crossref] [PubMed]
- Utada M, Chernyavskiy P, Lee WJ, et al. Increasing risk of uterine cervical cancer among young Japanese women: Comparison of incidence trends in Japan, South Korea and Japanese-Americans between 1985 and 2012. Int J Cancer 2019;144:2144-52. [Crossref] [PubMed]
- Johnson CA, James D, Marzan A, et al. Cervical Cancer: An Overview of Pathophysiology and Management. Semin Oncol Nurs 2019;35:166-74. [Crossref] [PubMed]
- Naga Ch P, Gurram L, Chopra S, et al. The management of locally advanced cervical cancer. Curr Opin Oncol 2018;30:323-9. [Crossref] [PubMed]
- Veisi A, Akbari G, Mard SA, et al. Role of crocin in several cancer cell lines: An updated review. Iran J Basic Med Sci 2020;23:3-12. [PubMed]
- Luo Y, Cui S, Tang F, et al. The combination of crocin with cisplatin suppresses growth of gastric carcinoma cell line BGC-823 and promotes cell apoptosis. Pak J Pharm Sci 2017;30:1629-34. [PubMed]
- Chen S, Zhao S, Wang X, et al. Crocin inhibits cell proliferation and enhances cisplatin and pemetrexed chemosensitivity in lung cancer cells. Transl Lung Cancer Res 2015;4:775-83. [PubMed]
- Kawabata K, Tung NH, Shoyama Y, et al. Dietary Crocin Inhibits Colitis and Colitis-Associated Colorectal Carcinogenesis in Male ICR Mice. Evid Based Complement Alternat Med 2012;2012:820415. [Crossref] [PubMed]
- Mir MA, Ganai SA, Mansoor S, et al. Isolation, purification and characterization of naturally derived Crocetin beta-d-glucosyl ester from Crocus sativus L. against breast cancer and its binding chemistry with ER-alpha/HDAC2. Saudi J Biol Sci 2020;27:975-84. [Crossref] [PubMed]
- Arzi L, Farahi A, Jafarzadeh N, et al. Inhibitory Effect of Crocin on Metastasis of Triple-Negative Breast Cancer by Interfering with Wnt/β-Catenin Pathway in Murine Model. DNA Cell Biol 2018;37:1068-75. [Crossref] [PubMed]
- Chen SS, Gu Y, Lu F, et al. Antiangiogenic effect of crocin on breast cancer cell MDA-MB-231. J Thorac Dis 2019;11:4464-73. [Crossref] [PubMed]
- Hoshyar R, Bathaie SZ, Kyani A, et al. Is there any interaction between telomeric DNA structures, G-quadruplex and I-motif, with saffron active metabolites? Nucleosides Nucleotides Nucleic Acids 2012;31:801-12. [Crossref] [PubMed]
- Aanouz I, Belhassan A, El-Khatabi K, et al. Moroccan Medicinal plants as inhibitors against SARS-CoV-2 main protease: Computational investigations. J Biomol Struct Dyn 2020.1-9. [Crossref] [PubMed]
- Patil S, Sistla S, Jadhav J. Interaction of small molecules with human tyrosinase: A surface plasmon resonance and molecular docking study. Int J Biol Macromol 2016;92:1123-9. [Crossref] [PubMed]
- Salem AA, Lotfy M, Amin A, et al. Characterization of human serum albumin’s interactions with safranal and crocin using multi-spectroscopic and molecular docking techniques. Biochem Biophys Rep 2019;20:100670. [Crossref] [PubMed]
- Luo S, Gill H, Feltis B, et al. The Effects of a Weight-Loss Herbal Formula RCM-107 and Its Eight Individual Ingredients on Glucagon-Like Peptide-1 Secretion-An In Vitro and In Silico Study. Int J Mol Sci 2020;21:2854. [Crossref] [PubMed]
- Hashemi SA, Bathaie SZ, Mohagheghi MA. Interaction of saffron carotenoids with catalase: in vitro, in vivo and molecular docking studies. J Biomol Struct Dyn 2020;38:3916-26. [Crossref] [PubMed]
- Khedr LH, Rahmo RM, Farag DB, et al. Crocin attenuates cisplatin-induced hepatotoxicity via TLR4/NF-κBp50 signaling and BAMBI modulation of TGF-β activity: Involvement of miRNA-9 and miRNA-29. Food Chem Toxicol 2020;140:111307. [Crossref] [PubMed]
- Kim SH, Lee JM, Kim SC, et al. Proposed cytotoxic mechanisms of the saffron carotenoids crocin and crocetin on cancer cell lines. Biochem Cell Biol 2014;92:105-11. [Crossref] [PubMed]
- Sawant AV, Srivastava S, Prassanawar SS, et al. Crocin, a carotenoid, suppresses spindle microtubule dynamics and activates the mitotic checkpoint by binding to tubulin. Biochem Pharmacol 2019;163:32-45. [Crossref] [PubMed]
- Jiang Z, Gu M, Liu J, et al. Anticancer activity of crocin against cervical carcinoma (HeLa cells): Bioassessment and toxicity evaluation of crocin in male albino rats. J Photochem Photobiol B 2018;180:118-24. [Crossref] [PubMed]
- Mohan S, Hemachandran H, Sneha P, et al. Structural insights into the binding mode and conformational changes of BSA induced by bixin and crocin. J Biomol Struct Dyn 2018;36:2085-98. [Crossref] [PubMed]
(English Language Editor: C. Belazar-Maseh)