Optimal target in septic shock resuscitation
Introduction
Septic shock is operationally defined as an infection-related circulatory dysfunction on which vasopressors are required to maintain a mean arterial pressure (MAP) of 65 mmHg or greater, and with a serum lactate level greater than 2 mmol/L in the absence of hypovolemia. This condition is associated with organ dysfunction and a very high mortality risk (1). Critical aspects of management are early recognition, effective and timely source control, and hemodynamic resuscitation (2). Septic shock resuscitation means the use of therapeutic strategies aimed at rapidly restoring tissue perfusion by improving systemic blood flow with titration of fluids and vasoactive agents (3). At the same time, it is imperative for an optimal management to avoid excessive interventions that could lead to over-resuscitation, and thus, paradoxically, increase mortality or morbidity (4–6). This latter aspect requires a fine tuning of interventions with the aim of stopping resuscitation when physiologically sound targets have been achieved, and thus, there are reasonable odds of tissue perfusion being restored (7). It is however relevant to emphasize that macrocirculatory variables should be adjusted to meet perfusion requirements, and no absolute value can be considered as normal or pursued during resuscitation.
The purpose of this article is to give some perspective on perfusion monitoring in septic shock, and to provide an integrative view and recommendations after the ANDROMEDA-SHOCK trial (8). Definitions for some terms used in this review are provided in Table 1.
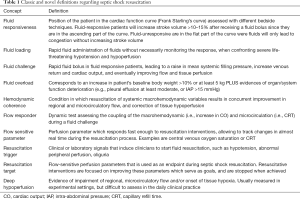
Full table
Stages of septic shock, hemodynamic coherence and perfusion assessment
Early stage
The pathogenic mechanisms involved in sepsis-related acute circulatory dysfunction are multiple and change over time. Initial stage is characterized by loss of vascular tone, a fundamental pathophysiological mechanism (9). This leads to hypotension by arterial vasodilation but also to an important decrease in venous tone. Consequently, stressed volume, mean systemic filling pressure and the driving pressure for venous return decrease, leading to a fall in preload (relative hypovolemia) and cardiac output (CO). These events induce a decrease in systemic blood flow and mean arterial pressure (MAP) which is considered the perfusion pressure (10,11). A strong adrenergic and neurohumoral compensatory response is triggered generating vasoconstriction at the splanchnic venous reservoir improving venous return, but also on the arterial side increasing MAP. In addition, CO increases through the inotropic effect of epinephrine and norepinephrine (12). Unfortunately, there are several drawbacks for this response such as myocardial over-stimulation, and hepatosplanchnic vasoconstriction that could be deleterious if sustained over time (13).
It is interesting that in this early stage, and depending on the magnitude of the compensatory response, the microcirculation is still functionally preserved although with low flow (13). Early resuscitation with maneuvers like fluids or vasopressors that restore CO and/or MAP may improve microcirculatory flow and tissue perfusion. This coupling between macrocirculation and microcirculation is called hemodynamic coherence, which is a physiological state (14,15). The dynamic counterpart is flow responsiveness, implying that in patients with preserved coupling an increase in systemic blood flow as for example with fluid boluses may improve regional and microcirculatory flow, and hypoperfusion-related markers.
Perfusion assessment in this early stage is simple. Peripheral perfusion reflects high adrenergic tone and low blood flow, and is obviously impaired with prolonged capillary refill time (CRT) and mottling (16). After successful resuscitation, adrenergic tone is abated and CO increases, and thus skin flow improves. In fact, CRT normalization after a rapid fluid loading or challenge, may be a signal of a satisfactory reperfusion (17). Low central venous oxygen saturation (ScvO2) reflecting increased O2 extraction (18,19), and high venous to arterial pCO2 difference (pCO2 gap) signaling venous pCO2 stagnation due to low flow (20,21), improve also very rapidly in response to increases in systemic blood flow. These three parameters are considered flow-sensitive as they respond in minutes to increments in CO, and thus are suitable to assess the response to a fast-acting therapy such as a fluid bolus almost in real-time or after a very short time-period (e.g., 15 minutes) (22).
Lactate, on the contrary, which is usually abnormal due to increased anaerobic production in hypoperfused tissues or adrenergic-driven muscle aerobic production, may show an initial relatively rapid decrease due to microcirculatory flow improvement and a decrease in adrenergic tone (23-25). However, residual lactate is redistributed or metabolized at slower rate, and thus lactate cannot be used to evaluate the effect of a single fluid bolus or other fast-acting therapies. Moreover, expert recommend to measure lactate only after 2-hour intervals (26).
From a clinical perspective and a hierarchical point of view, the most appropriate parameter for this early stage may be CRT. Among several reasons, it is simple, universally available, free of cost, and does not require invasive catheterization. Standardization of the technique as used in the ANDROMEDA-SHOCK trial should decrease inter-rater reliability.
In addition, it may serve for triage decisions. Lara et al. performed a prospective observational study at the emergency department (ED) of a major teaching hospital (17). Ninety-five patients with sepsis-related circulatory dysfunction and hyperlactatemia were included. CRT was assessed before and after the initial fluid loading of 30 mL/kg as recommended by the Surviving Sepsis Campaign. Patients with abnormal CRT after this very early fluid resuscitation presented an increased risk of adverse outcomes [RR 4.4 (2.7–7.4), P<0.001] and mortality [RR 6.7 (2.9–16)] compared to those with normal CRT. Eventually, the status of CRT after initial fluid loading could be used for triage purposes, meaning that patients with abnormal CRT should be rapidly transferred to the intensive care unit (ICU).
Progressive (late) stage
In patients evolving into advanced septic shock, progressive endothelial and coagulation dysfunction induce severe derangements at the microcirculatory level with alterations in flow, perfused density and heterogeneity which compromises tissue/cellular oxygenation (9). An increase in capillary permeability produces leakage. Fluid overload worsens alterations by increasing venous congestion, interstitial edema and diffusion distance for oxygen (4). This and other abnormalities are responsible for the loss of hemodynamic coherence (14), generating a new status where macrocirculation and microcirculation are dissociated, meaning that an increase in systemic blood flow does not improve microcirculatory perfusion. On the contrary, persisting on fluid administration will only hasten microcirculatory dysfunction as stated above. The presence of tissue hypoxia is likely and the reperfusion process is severely limited by the status of microcirculation.
What is the role of perfusion assessment in this late stage? As a difference with earlier stages a myriad of sometimes contrasting abnormalities may be found. Persistent hyperlactatemia is universally present in the context of microcirculatory dysfunction with tissue hypoxia and acidosis. In addition, many patients evolve with progressive liver dysfunction, severe inflammation and hyperadrenergia contributing to hyperlactatemia (24). ScvO2 however, might be normal or even supranormal reflecting derangements in oxygen extraction capabilities due to microcirculatory alterations (27). pCO2 gap is frequently abnormal reflecting not only stagnation but eventually also anaerobic metabolism and hypoxia in advanced shock states (28). Interestingly, Ospina-Tascón et al., demonstrated that an increased pCO2 gap can track microcirculatory abnormalities at this stage (28). Peripheral perfusion is almost always abnormal and does not respond to increases in systemic flow. Many experts consider that somehow abnormal peripheral perfusion at this stage represents more skin microcirculatory abnormalities than a flow-sensitive parameter.
Complexities in the interpretation of traditional perfusion-related variables
One basic principle in septic shock resuscitation is that early goal-directed therapies are aimed at preventing or reverting tissue hypoxia (29). Unfortunately, hypoxia is a cellular/mitochondrial phenomenon that is beyond any routine clinical and/or laboratory assessment or detection technique. All the commonly used perfusion-related variables such as ScvO2, pCO2 gap, lactate or peripheral perfusion can only be considered as surrogates of this process as we will discuss below.
A low ScvO2 represents a disequilibrium between global or regional (superior vena cava territory) oxygen transport and consumption (18). However, an increase in O2 extraction when demands are incremented or delivery is decreased is an early physiological response that tends to prevent tissue hypoxia. A low ScvO2 is not indicative of tissue hypoxia per se, but a high or supranormal ScvO2 in patients clearly deteriorating their clinical hemodynamic condition may be suggestive of severe microcirculatory dysfunction (27).
Monitoring of pCO2 gap early in septic shock resuscitation may allow to assess the adequacy of CO to meet tissue perfusion demands (20). Venous CO2 stagnation is cleared when systemic flow increases. A high pCO2 gap in a patient with abnormal lactate or peripheral perfusion should lead to optimize CO as part of resuscitation efforts (25). When tissue hypoxia appears, anaerobic CO2 is generated inducing a sharp rise in pCO2 gap, but this is a late event (20).
Lactate is a complex metabolic parameter (24). Traditionally thought as representing tissue hypoxia, current knowledge has moved to heterogeneous sources for persistent hyperlactatemia including stress-related adrenergic-driven generation in skeletal muscles and decreased hepatic lactate clearance (23). This is very relevant since to pursue lactate normalization as recommended by current guidelines (2) may lead to over-resuscitation with increased mortality and morbidity in an unknown number of patients. A couple of observational studies suggest that a multimodal perfusion monitoring could aid in the interpretation of hyperlactatemia (7,25). Normal simultaneous ScvO2, pCO2 gap and peripheral perfusion disclose a predominately non-hypoperfusion related hyperlactatemia and may be treated more conservatively (20). Other authors propose central venous-arterial pCO2 gradient/arterial-venous O2 content difference ratio to ascertain a hypoxia-related hyperlactatemia but more studies are required to settle this point (30).
The complexity of sepsis-related acute circulatory dysfunction makes it impossible to consider just one single parameter as the unique way to monitor tissue perfusion or guide septic shock resuscitation. All individual parameters have limitations and interpretation difficulties which makes a multimodal perfusion monitoring as imperative.
Potential role for CRT to determine the status of hemodynamic coherence
An early identification of patients that already lost hemodynamic coherence could be relevant since in this setting it is probably futile and even harmful to continue pushing CO risking the toxicity of over-resuscitation. Eventually, an early identification of this phenotype could be useful in the future for research purposes on rescue or immunomodulatory therapies focused on a specific cohort.
A major risk of ICU-based fluid resuscitation is to induce fluid overload which increases mortality and morbidity due to worsening organ dysfunction, prolonged mechanical ventilation, and intra-abdominal hypertension, among other consequences (4,6). Administering fluids to patients with septic shock after they lost hemodynamic coherence might deteriorate tissue oxygenation, even if they are still fluid-responsive in cardiac function terms. This is a very important consideration. Assessment of hemodynamic coherence or flow responsiveness is a step forward over the fluid responsiveness concept. The latter looks at the cardiac function curve, but the former instead at the holistic relationship between different components of the cardiovascular system (Figure 1).
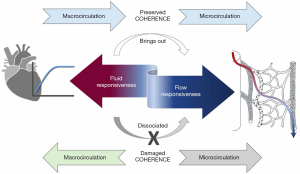
The problem is that no single static parameter can predict the status of hemodynamic coherence, and therefore, fluids are abused and probably contribute to progression to refractory shock and death. This is a fundamental contradiction in septic shock resuscitation and highlights the difference between the concepts of fluid responsiveness and hemodynamic coherence (Table 1). As an example, patients with capillary leak maintain fluid responsiveness along the process because fluids are rapidly lost to the interstitium, and the severe endothelial/microcirculatory dysfunction precludes reperfusion. So, these patients are both fluid-responsive and uncoupled. Moreover, clinicians in despair keep pushing more fluids to try to correct hypoperfusion, which only worsens microcirculatory abnormalities and further impairs perfusion.
Only a dynamic test could reveal if the macrocirculation is still coupled or not to regional/microcirculatory blood flow and prevent mismanagement and fluid overload as stated above. As a hypothesis, CRT response to a single rapid fluid challenge may be used as a novel “hemodynamic coherence or flow responsiveness test” (31) (Figure 2). CRT is a bridge between macro-and microcirculation, since it directly represents systemic blood flow due to the lack of autoregulation, and microcirculation, at least of the skin as has been suggested by several authors (30). Normalization of CRT represents an improvement in regional and microcirculatory skin perfusion secondary to an increase in systemic blood flow and/or a reactive decrease in adrenergic tone, thus reflecting hemodynamic coherence. On the contrary, CRT non-response after a rapid fluid challenge is abnormal and a signal of loss of coherence. However, there are no data supporting the usefulness of CRT monitoring in situations such as extreme capillary leak where preload and flow change dramatically sometimes in short periods of time.
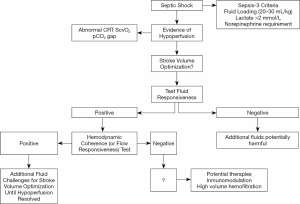
There are supporting data for this hypothesis: (I) patients with normal vs. abnormal CRT after fluid resuscitation in our previous studies exhibited a highly significant difference in hospital or 28-day mortalities [ED observational: 8.8% vs. 55.6% (17); ICU observational 15% vs. 50% (22); ANDROMEDA-SHOCK: 23% vs. 49% (at 8 h) (8)]. (II) In a pilot study, patients that improved CRT after fluid resuscitation, also exhibited an increase in hepatosplanchnic blood flow (32), eventually representing a decrease in adrenergic tone. (III) CRT exhibited the fastest kinetics of recovery in septic shock survivors which can only be explained by preserved coherence (7). (IV) Normalization of CRT in the ANDROMEDA-shock study was associated to less organ dysfunction which points in the same direction (8). (V) Early fluid resuscitation improved microcirculatory flow in a classic ED study (33).
Concerning the results of the ANDROMEDA-SHOCK study, although results are very encouraging some limitations should be kept in mind, particularly the potential inter-observer variability in CRT assessment. In the study a standardized approach was used but this is not a common practice elsewhere. In addition, some results were close to significance and thus it is imperative to confirm the trends in further large studies.
There are many possible explanations for why CRT might not respond to a stroke volume increase induced by a rapid fluid challenge: (I) a more advanced stage of septic shock with uncoupling between macro- and microcirculation (14). (II) An insufficient increase in stroke volume after the fluid challenge. (III) An excessive adrenergic tone with regional/microcirculatory hypoperfusion. (IV) A more severe endothelial or coagulation activation/dysfunction which derangements in microcirculatory perfusion (34).
If this hypothesis is confirmed in the future, the CRT-response to a rapid fluid challenge could be used as a hemodynamic coherence or flow responsiveness test, and help to avoid futile and dangerous further fluid administration in uncoupled patients, and eventually reduce additional iatrogenic-related excess mortality. Fluid resuscitation could then be focused in fluid responsive patients in whom hemodynamic coherence is still preserved while other perfusion parameters such as ScvO2, and pCO2 gap are still not normalized.
Furthermore, establishing the status of hemodynamic coherence with this simple test in pre-ICU or resource-limited settings, could eventually aid in taking triage decisions. CRT non-responders who exhibit very high mortality should be rapidly transferred to hospitals with ICU facilities for advanced monitoring and treatment, including reinforcement of source control and eventually rescue therapies (17).
Relationship between perfusion-related variables and regional/microcirculatory flow and hypoxia parameters: the deep hypoperfusion concept
In experimental settings, regional blood flow and distribution can be assessed directly and this allowed in the past to explore the impact of fluids and vasoactive agents on renal, mesenteric and hepatic blood flows. Gastric tonometry, intragastric Laser-Doppler, hepatic vein catheterization and pulsatility indexes of some hepatosplanchnic arteries have been used in patients to assess different aspects of flow and perfusion (35). Sublingual microcirculatory evaluation has not entered routine clinical practice and remains restricted to the research arena (13,36). Technical and logistical aspects, and disagreement between experts on the best way to analyze images have precluded further development.
The concept of deep hypoperfusion refers to the evidence of impairment in regional or microcirculatory flow, or abnormalities of hypoxia surrogate markers such as lactate/pyruvate or central venous-arterial pCO2 gradient/arterial-venous O2 content difference ratios (Table 1). The relation between clinically assessable parameters and deep hypoperfusion markers has been scarcely addressed by experimental or clinical research.
Brunauer et al. found a significant correlation between changes in CRT and mottling score with the pulsatility index of various hepatosplanchnic arteries during septic shock resuscitation (32). This correlation is physiologically coherent since both territories are affected by the same adrenergic response to circulatory stress that could be reversed at least partially by increments in systemic flow as was demonstrated in that study.
In an earlier study, association between perfusion-related parameters with 6-hour lactate clearance was studied in a cohort of hyperdynamic septic shock patients (37). Patients with a 6-hour lactate clearance <10% presented a worse hepatosplanchnic flow as represented by significantly more severe derangements of indocyanine green plasma disappearance rate [9.7% (8–19%) vs. 19.6% (9–32%)/min, P<0.05] and intragastric tonometric pCO2 {33 [9.1–62] vs. 7.7 [3–58] mmHg, P<0.05} at 6 hours. Thus, delayed lactate clearance appears related at least in part to hepatosplanchnic hypoperfusion.
In an experimental endotoxic shock model the relationship between liver blood flow and lactate clearance was explored in depth (38). After anesthesia and invasive monitoring including total hepatic blood flow with an ultrasound flow probe, and portal/hepatic vein catheterization, an endotoxin bolus was administered and followed by a continuous infusion. Exogenous lactate clearance was calculated by IV administration of lactate sodium and repeated sampling. Compared to sham, endotoxic animals exhibited a dramatic decrease of lactate clearance to less than 10% of controls [46 (30 to 180) versus 1,212 (743 to 2,116) mL/min, P<0.01]. However, this finding was not associated with liver hypoperfusion since total liver blood flow, exogenous sorbitol clearance (determined by flow), liver enzymes, and ex vivo mitochondrial respiration were comparable between endotoxic shock and controls. The authors speculated that a metabolic blockade or liver microcirculatory abnormalities could explain the results but unfortunately these mechanisms were not explored in the study. These data suggest that both hepatosplanchnic hypoperfusion and some sort of liver dysfunction but with preserved flow, may be involved in abnormal lactate clearance in early septic shock.
A recent clinical physiological randomized controlled trial compared CRT versus lactate –targeted fluid resuscitation in early septic shock including deep hypoperfusion parameters (unpublished data). Among patients that achieved targets in both groups, values of perfusion-related parameters [e.g., microcirculatory flow index 3 (2.5–3) vs. 2.9 (2.75–3), P=0.9; lactate/pyruvate ratios 7.9 (3.77–13.6) vs. 6.3 (2.46–13.9), P=0.68; muscle oxygen saturation 75% (68–85%) vs. 79% (68–85%), P=0.67], were comparable. Interestingly, less patients in CRT group had evidence of deep hypoperfusion, defined by a composite outcome of regional/microcirculatory flow impairment and hypoxia surrogates (7% vs. 43%, P=0.07) (unpublished data). Additionally, another study that compared dobutamine vs. placebo on macrohemodynamics, regional, peripheral and microcirculatory perfusion-related parameters in hyperdynamic septic shock, demonstrated that patients who normalized CRT exhibited also normal muscle O2 saturation and plasma disappearance rate for indocyanine green (35).
The sum of the previous data supports the hierarchical role for CRT where at least some studies with different designs tend to support that a normal CRT is associated with preserved hepatosplanchnic and muscle flow, and hypoxia surrogates (7,32,35). Lactate is much more complex as exemplified in the lactate clearance’s cited studies.
In any case, evidence is scanty and incidental, and therefore more research is required to better define the relationship between systemic perfusion variables and deep hypoperfusion markers.
The concept and complexities of a fluid challenge
Since absolute or relative hypovolemia is almost universally present in early septic shock, resuscitation starts with fluid loading in pre-ICU settings, such as the ED or wards (39). Fluid loading is rapid administration of fluids without necessarily monitoring the response in real-time when confronting severe life threatening hypotension and hypoperfusion. This constitutes the “R = rescue” of the acronym ROSE for describing the phases of fluid administration, and usually involves 20–30 mL/kg of crystalloids as recommended by current guidelines (40). Fluid challenge on the contrary, is a fluid bolus, rapid and large enough to raise the mean systemic filling pressure and increase venous return and CO in fluid-responsive patients, eventually tissue perfusion.
If circulatory dysfunction is not resolved with this early management, patients are transferred to the ICU, where advanced fluid resuscitation is started with the fundamental objective to increase systemic blood flow. This is mentioned as “O = optimization” in ROSE (41). The initial step is assessment of fluid responsiveness (4,41). In the ANDROMEDA-SHOCK study (8,42,43), we found that only 70% of the patients were fluid-responsive at ICU admission, and thus we could avoid unnecessary fluids in the other 30%. Patients that were fluid unresponsive could be managed with alternative protocol steps such as vasopressor adjustment and/or inodilators depending on the context with similar rates of target achievement despite receiving significantly less fluids.
The standard practice is to perform a fluid challenge in fluid-responsive patients who are still hypoperfused (41,44), eventually improving tissue perfusion, depending on the status of hemodynamic coherence (14). Fluid is given as a fluid challenge so that response can be assessed looking at the target, and the need for ongoing fluid therapy ascertained (Table 1).
There is also a fundamental distinction between triggers and targets for fluid resuscitation (39,45). Clinicians use a wide variety of triggers to start fluid administration as the FENICE study demonstrated (44). The most cited ones were hypotension, oliguria, and weaning of vasopressors, indications that are highly debatable and could lead to fluid-overload. Perfusion-related variables were mentioned by only 8% of respondents.
Targets are parameters against which fluid boluses are directed. The correct targets for septic shock resuscitation are perfusion-related variables whose correction correlates with improvement in tissue or microcirculatory flow, and that ideally exhibit a real-time response to increments in CO or perfusion pressure. In addition, they should be simple, and universally available. Results of the ANDROMEDA-SHOCK study support the use of CRT as a target for fluid resuscitation (8,46).
A physiological and conceptual view of the best way to perform a fluid challenge is shown in Figure 3. Triggers should be rational. For example, a septic shock patient with low diastolic blood pressure (<40 mmHg and with tachycardia) has clearly a dramatic decrease in vascular tone, and according to basic physiology, vascular tone is not restored with crystalloids but instead might be a good indication for early vasopressors (12). A hypotensive patient with low pulse pressure on the contrary may be a good candidate for fluid administration aimed at preload recovery provided there are no clinical or echocardiographic signs of cardiac dysfunction. Oliguria appears as a very irrational trigger and target for several reasons discussed in detail elsewhere (39). Fluid administration does not necessarily lead to restoration of normal diuresis. Urine output is a variable that takes time to change and is influenced by factors other than hemodynamic status. Therefore, pursuing a reversion of oliguria may be harmful and could lead to fluid overload.
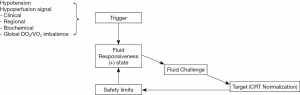
If the patient is fluid-responsive, a fluid challenge (typically 500 mL crystalloids administered in 30 minutes) is performed and the target (as CRT) is reassessed (Figure 3) (10). If the target is corrected, it is only monitored periodically thereafter. If still abnormal, a new fluid challenge is considered provided two conditions are met, first that a safety limit is not reached as for example a rise in central venous pressure (CVP) ≥5 mmHg (47), and second, that the patient is still fluid responsive when reassessed.
Impact of MAP level/and or vasopressor dose on tissue perfusion
In fluid unresponsive patients, other alternatives to restore tissue perfusion should be tested. An initial MAP of 60–65 mmHg for all patients appears as appropriate since this level is probably over the theoretical autoregulation threshold for the majority. Patients might need higher MAPs if they have chronic hypertension, however, the impact of vasopressors is debatable, and there is no consensus on this topic. Microcirculation-focused studies using the side-dark stream technique suggest that patients with impaired sublingual microcirculation at baseline may improve flow when MAP is increased from 65 to 85 mmHg, but unfortunately some patients with preserved microcirculation at baseline deteriorated microcirculatory flow when increasing MAP (48). Therefore, and considering the controversial results of the SEPSISPAM study, no conclusions can be firmly drawn (49).
In the ANDROMEDA-SHOCK trial a pragmatic approach was selected, the vasopressor test (8,43). MAP was transiently raised to 80–85 mmHg in patients with past medical history of arterial hypertension who had not achieved targets with the fluid protocol step. After one or two hours depending on the allocated group, targets were reevaluated and if still abnormal, MAP was decreased to the original level of 60–65 mmHg. On the contrary, patients who achieved targets maintained this MAP level until the end of the 8-hour intervention period. Fewer patients in the peripheral perfusion group (28.8%) than in the lactate group (40.1%) required a vasopressor test (P=0.02), although success was not different (44% vs. 38%, respectively; P=0.86). The vasopressor test is somehow a way to select patients in whom an increase in perfusion pressure predominates over local precapillary vasoconstriction induced by vasopressors and by performing a transient maneuver no harm is induced.
Inodilators as a last step
Inodilators, especially dobutamine, have been used in the past, and are still recommended by some guidelines, to increase systemic blood flow and thus improve tissue perfusion (2). A direct local vasodilation independent of cardiac effects has been suggested by some previous clinical and experimental studies, but not clearly demonstrated (50). In several contemporary protocols the addition of dobutamine has been the last step in the sequence of interventions aimed at achieving resuscitation targets (44,51,52). However, most of the studies were performed in the pre-echocardiography era and it is not clear if patients without clinically relevant myocardial dysfunction are benefited. In the previously cited cross-over double blind randomized controlled trial testing dobutamine versus placebo as a short intervention, no benefit could be demonstrated as evaluated with a comprehensive multimodal perfusion assessment that included gut tonometry, sublingual microcirculation, plasma disappearance rate of indocyanine green, muscle O2 saturation, among others (35). In the ANDROMEDA-SHOCK trial, 15% of the patients received inodilators without any significant difference between groups (8). Thus, the role of inodilators is still questionable.
How to integrate all these concepts into a CRT-targeted resuscitation algorithm
A conceptual management algorithm for septic shock resuscitation is provided in Figure 4. Multimodal perfusion monitoring is pivotal in the process. Basic hemodynamic monitoring with a central venous catheter and an arterial line allows calculation of ScvO2 and pCO2 gap. An integrated analysis of these 2 parameters can determine flow status and lead to optimize DO2/VO2 relationship. The concept of flow responsiveness as signal of preserved hemodynamic coherence may be tested by a rapid fluid challenge (e.g., 4 mL/kg in 5 min) in fluid responsive patients and evaluating CRT response (see text above). The sequential steps proposed in ANDROMEDA-SHOCK may be used to pursue the CRT target in flow-responsive patients, while in parallel other aspects of DO2/VO2 relationship are optimized. Lactate can be monitored periodically for prognostic purposes, and to assess the global adequacy of the resuscitation process and adjust other aspects such as source control.
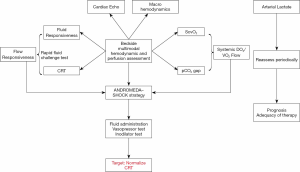
Conclusions
Advances in our pathophysiological understanding of the mechanisms and progressive stages of septic shock can lead to more rational resuscitation algorithms. New concepts such as hemodynamic coherence and flow responsiveness can be used at the bedside to select the best treatment strategies at any time-point. A multimodal perfusion monitoring and an integrated analysis with macrohemodynamic parameters is obligatory to optimize the resuscitation process.
CRT appears as a hierarchical resuscitation target that can be pursued following the successful ANDROMEDA-SHOCK protocol. A normal CRT is probably associated with resolution of deep hypoperfusion markers.
Acknowledgments
Funding: None.
Footnote
Provenance and Peer Review: This article was commissioned by the Guest Editors (Glenn Hernández and Guo-Wei Tu) for the series “Hemodynamic monitoring in critically ill patients” published in Annals of Translational Medicine. The article was sent for external peer review organized by the Guest Editors and the editorial office.
Conflicts of Interest: All authors have completed the ICMJE uniform disclosure form (available at http://dx.doi.org/10.21037/atm-20-1120). The series “Hemodynamic monitoring in critically ill patients” was commissioned by the editorial office without any funding or sponsorship. GH served as the unpaid Guest Editor of the series. The other authors have no other conflicts of interest to declare.
Ethical Statements: The authors are accountable for all aspects of the work in ensuring that questions related to the accuracy or integrity of any part of the work are appropriately investigated and resolved.
Open Access Statement: This is an Open Access article distributed in accordance with the Creative Commons Attribution-NonCommercial-NoDerivs 4.0 International License (CC BY-NC-ND 4.0), which permits the non-commercial replication and distribution of the article with the strict proviso that no changes or edits are made and the original work is properly cited (including links to both the formal publication through the relevant DOI and the license). See: https://creativecommons.org/licenses/by-nc-nd/4.0/.
References
- Singer M, Deutschman CS, Seymour CW, et al. The Third International Consensus Definitions for Sepsis and Septic Shock (Sepsis-3). JAMA 2016;315:801-10. [Crossref] [PubMed]
- Rhodes A, Evans L, Alhazzani W, et al. Surviving Sepsis Campaign: International Guidelines for Management of Sepsis and Septic Shock: 2016. Crit Care Med 2017;45:486-552. [Crossref] [PubMed]
- Hernandez G, Bruhn A, Castro R, et al. The holistic view on perfusion monitoring in septic shock. Curr Opin Crit Care 2012;18:280-6. [Crossref] [PubMed]
- Bagshaw SM, Brophy PD, Cruz D, et al. Fluid balance as a biomarker: impact of fluid overload on outcome in critically ill patients with acute kidney injury. Crit Care 2008;12:169. [Crossref] [PubMed]
- Boyd JH, Forbes J, Nakada TA, et al. Fluid resuscitation in septic shock: A positive fluid balance and elevated central venous pressure are associated with increased mortality. Crit Care Med 2011;39:259-65. [Crossref] [PubMed]
- Payen D, de Pont AC, Sakr Y, et al. A positive fluid balance is associated with a worse outcome in patients with acute renal failure. Crit Care 2008;12:R74. [Crossref] [PubMed]
- Hernandez G, Luengo C, Bruhn A, et al. When to stop septic shock resuscitation: clues from a dynamic perfusion monitoring. Ann Intensive Care 2014;4:30. [Crossref] [PubMed]
- Hernández G, Ospina-Tascon G, Petri Damiani L, et al. Effect of a Resuscitation Strategy Targeting Peripheral Perfusion Status vs Serum Lactate Levels on 28-Day Mortality Among Patients With Septic Shock. The ANDROMEDA-SHOCK Randomized Clinical Trial. JAMA 2019;321:654-64. [Crossref] [PubMed]
- Hernández G, Teboul JL. Is the macrocirculation really dissociated from the microcirculation in septic shock? Intensive Care Med 2016;42:1621-4. [Crossref] [PubMed]
- Monnet X, Teboul JL. My patient has received fluid. How to assess its efficacy and side effects? Ann Intensive Care 2018;8:54. [Crossref] [PubMed]
- Berlin DA, Bakker J. Understanding venous return. Intensive Care Med 2014;40:1564-6. [Crossref] [PubMed]
- Hernández G, Teboul JL, Bakker J. Norepinephrine in septic shock. Intensive Care Med 2019;45:687-9. [Crossref] [PubMed]
- Dubin A, Henriquez E, Hernández G. Monitoring peripheral perfusion and microcirculation. Curr Opin Crit Care 2018;24:173-80. [Crossref] [PubMed]
- Ince C. Hemodynamic coherence and the rationale for monitoring the microcirculation. Crit Care 2015;19:S8. [Crossref] [PubMed]
- Morelli A, Passariello M. Hemodynamic coherence in sepsis. Best Pract Res Clin Anaesthesiol 2016;30:453-63. [Crossref] [PubMed]
- Lima A, Jansen TC, Van Bommel J, et al. The prognostic value of the subjective assessment of peripheral perfusion in critically ill patients. Crit Care Med 2009;37:934-8. [Crossref] [PubMed]
- Lara B, Enberg L, Ortega M, et al. Capillary refill time during fluid resuscitation in patients with sepsis-related hyperlactatemia at the emergency department is related to mortality. PLoS One 2017;12:e0188548. [Crossref] [PubMed]
- Bauer P, Reinhart K, Bauer M. Significance of venous oximetry in the critically ill. Med Intensiva 2008;32:134-42. [Crossref] [PubMed]
- Boulain T, Garot D, Vignon P, et al. Prevalence of low central venous oxygen saturation in the first hours of intensive care unit admission and associated mortality in septic shock patients: a prospective multicentre study. Crit Care 2014;18:609. [Crossref] [PubMed]
- Gavelli F, Teboul JL, Monnet X. How can CO2-derived indices guide resuscitation in critically ill patients? J Thorac Dis 2019;11:S1528-37. [Crossref] [PubMed]
- Scheeren TWL, Wicke JN, Teboul JL. Understanding the carbon dioxide gaps. Curr Opin Crit Care 2018;24:181-9. [Crossref] [PubMed]
- Hernandez G, Pedreros C, Veas E, et al. Evolution of peripheral vs metabolic perfusion parameters during septic shock resuscitation. A clinical-physiologic study. J Crit Care 2012;27:283-8. [Crossref] [PubMed]
- Bakker J, de Backer D, Hernandez G. Lactate-guided resuscitation saves lives: we are not sure. Intensive Care Med 2016;42:472-4. [Crossref] [PubMed]
- Hernandez G, Bellomo R, Bakker J. The ten pitfalls of lactate clearance in sepsis. Intensive Care Med 2019;45:82-5. [Crossref] [PubMed]
- Alegría L, Vera M, Dreyse J, et al. A hypoperfusion context may aid to interpret hyperlactatemia in sepsis-3 septic shock patients: a proof-of-concept study. Ann Intensive Care 2017;7:29. [Crossref] [PubMed]
- Vincent JL, Silva AQ, Couto L, et al. The value of blood lactate kinetics in critically ill patients: A systematic review. Crit Care 2016;20:257. [Crossref] [PubMed]
- Textoris J, Fouché L, Wiramus S, et al. High central venous oxygen saturation in the latter stages of septic shock is associated with increased mortality. Crit Care 2011;15:R176. [Crossref] [PubMed]
- Ospina-Tascón GA, Umaña M, Bermúdez WF, et al. Can venous-to-arterial carbon dioxide differences reflect microcirculatory alterations in patients with septic shock? Intensive Care Med 2016;42:211-21. [Crossref] [PubMed]
- MacIntyre NR. Tissue hypoxia: Implications for the respiratory clinician. Respir Care 2014;59:1590-6. [Crossref] [PubMed]
- Ospina-Tascón GA, Hernández G, Cecconi M. Understanding the venous-arterial CO2 to arterial-venous O2 content difference ratio. Intensive Care Med 2016;42:1801-4. [Crossref] [PubMed]
- Hernández G, Castro R, Bakker J. Capillary refill time: the missing link between macrocirculation and microcirculation in septic shock? J Thorac Dis 2020;12:1127-9. [Crossref] [PubMed]
- Brunauer A, Koköfer A, Bataar O, et al. Changes in peripheral perfusion relate to visceral organ perfusion in early septic shock: A pilot study. J Crit Care 2016;35:105-9. [Crossref] [PubMed]
- Trzeciak S, McCoy JV, Dellinger RP, et al. Early increases in microcirculatory perfusion during protocol-directed resuscitation are associated with reduced multi-organ failure at 24 h in patients with sepsis. Intensive Care Med 2008;34:2210-7. [Crossref] [PubMed]
- Ince C, Mayeux PR, Nguyen T, et al. The endothelium in sepsis. Shock 2016;45:259-70. [Crossref] [PubMed]
- Hernandez G, Bruhn A, Luengo C, et al. Effects of dobutamine on systemic, regional and microcirculatory perfusion parameters in septic shock: A randomized, placebo-controlled, double-blind, crossover study. Intensive Care Med 2013;39:1435-43. [Crossref] [PubMed]
- Vellinga NAR, Boerma EC, Koopmans M, et al. International Study on Microcirculatory Shock Occurrence in Acutely Ill Patients. Crit Care Med 2015;43:48-56. [Crossref] [PubMed]
- Hernandez G, Regueira T, Bruhn A, et al. Relationship of systemic, hepatosplanchnic, and microcirculatory perfusion parameters with 6-hour lactate clearance in hyperdynamic septic shock patients: an acute, clinical-physiological, pilot study. Ann Intensive Care 2012;2:44. [Crossref] [PubMed]
- Tapia P, Soto D, Bruhn A, et al. Impairment of exogenous lactate clearance in experimental hyperdynamic septic shock is not related to total liver hypoperfusion. Crit Care 2015;19:188. [Crossref] [PubMed]
- Cecconi M, Hernandez G, Dunser M, et al. Fluid administration for acute circulatory dysfunction using basic monitoring: narrative review and expert panel recommendations from an ESICM task force. Intensive Care Med 2019;45:21-32. [Crossref] [PubMed]
- Malbrain MLNG, Van Regenmortel N, Saugel B, et al. Principles of fluid management and stewardship in septic shock: it is time to consider the four D’s and the four phases of fluid therapy. Ann Intensive Care 2018;8:66. [Crossref] [PubMed]
- Monnet X, Teboul JL. Assessment of fluid responsiveness: Recent advances. Curr Opin Crit Care 2018;24:190-5. [Crossref] [PubMed]
- Kattan E, Ospina-Tascón GA, Teboul JL, et al. Systematic assessment of fluid responsiveness during early septic shock resuscitation: secondary analysis of the ANDROMEDA-SHOCK trial. Crit Care 2020;24:23. [Crossref] [PubMed]
- Hernández G, Cavalcanti AB, Ospina-Tascón G, et al. Early goal-directed therapy using a physiological holistic view: the ANDROMEDA-SHOCK—a randomized controlled trial. Ann Intensive Care 2018;8:52. [Crossref] [PubMed]
- Cecconi M, Hofer C, Teboul JL, et al. Fluid challenges in intensive care: the FENICE study: A global inception cohort study. Intensive Care Med 2015;41:1529-37. [Crossref] [PubMed]
- Cecconi M, Parsons AK, Rhodes A. What is a fluid challenge? Curr Opin Crit Care 2011;17:290-5. [Crossref] [PubMed]
- Zampieri FG, Damiani LP, Bakker J, et al. Effect of a Resuscitation Strategy Targeting Peripheral Perfusion Status vs Serum Lactate Levels on 28-Day Mortality Among Patients with Septic Shock: A Bayesian Reanalysis of the ANDROMEDA-SHOCK Trial. Am J Respir Crit Care Med 2020. [Crossref]
- De Backer D, Vincent JL. Should we measure the central venous pressure to guide fluid management? Ten answers to 10 questions. Crit Care 2018;22:43. [Crossref] [PubMed]
- Dubin A, Pozo MO, Casabella CA, et al. Increasing arterial blood pressure with norepinephrine does not improve microcirculatory blood flow: A prospective study. Crit Care 2009;13:R92. [Crossref] [PubMed]
- Asfar P, Meziani F, Hamel JF, et al. High versus Low Blood-Pressure Target in Patients with Septic Shock. N Engl J Med 2014;370:1583-93. [Crossref] [PubMed]
- De Backer D, Creteur J, Dubois MJ, et al. The effects of dobutamine on microcirculatory alterations in patients with septic shock are independent of its systemic effects. Crit Care Med 2006;34:403-8. [Crossref] [PubMed]
- Rivers E, Nguyen B, Havstad S, et al. Early-goal directed therapy in the treatment of severe sepsis and septic shock. N Engl J Med 2001;345:1368-77. [Crossref] [PubMed]
- Jansen TC, Van Bommel J, Schoonderbeek FJ, et al. Early lactate-guided therapy in intensive care unit patients: A multicenter, open-label, randomized controlled trial. Am J Respir Crit Care Med 2010;182:752-61. [Crossref] [PubMed]