Lidocaine mediates the progression of cerebral ischemia/reperfusion injury in rats via inhibiting the activation of NF-κB p65 and p38 MAPK
Introduction
Ischemic stroke, which can lead to permanent or transient reduction or suspension of cerebral blood flow, is one of the leading causes of global morbidity and mortality (1,2). This disorder impairs neuronal function and leads to cell death and subsequent neurological dysfunction. More than 5 million people die from stroke and another 5 million are permanently disabled each year (3). Early thrombolysis or reperfusion is the best treatment intervention for ischemic stroke, but this treatment aggravates the inflammation or glutamate excitotoxicity induced by ischemic stroke (4), which is considered to be the pathogenesis of stroke nerve injury (5). Although considerable advances have been made in treatment, the prognosis of ischemia/reperfusion (I/R) injury is generally poor (6), and there is still a need to develop safe and effective therapeutic drugs.
In different types of surgery, local anesthetics not only block the nerve signal, but also have a systemic anti-inflammatory effect (7). Among them, lidocaine, as an amide-derived local anesthetic, is commonly used to prevent pain during minor surgery or to relieve post-injury pain, with anti-inflammatory and antibacterial properties (8). In addition, lidocaine suppresses the expression of cytokine and free oxygen radicals by reducing cell mobility and adhesion (9). More recently, lidocaine has been used clinically for the treatment of I/R injury. Previous studies have examined lidocaine in relation to I/R damage in various tissues such as those of the heart (10), liver (11), and lung (12). Nevertheless, the role of lidocaine in cerebral I/R (CI/R) injury is still unknown.
Brain experiments show that the pathology of I/R is very complicated. Ischemia can cause rapid changes in the cell interior, leading to interference of signal transduction and surface molecules (13,14). There is evidence that inflammation is strongly associated with neuronal cell necrosis and causes neurological deficits (15). After transient cerebral ischemia, NF-κB is activated in neurons and confers neuroprotective effects to prolong subsequent ischemic time (16). In addition, the role of mitogen-activated protein kinase (MAPK) signal pathway in CI/R has been reported, with focus on the ERK, JNK, and p38 pathways (17).
In this study, we investigated the protective effect of lidocaine on brain I/R injury in male Sprague-Dawley rats, which were commonly used as a MCAO model. Furthermore, this study attempted to clarify along with the relationship between I/R-induced nervous injury and the NF-κB and p38 MAPK signaling pathways, and further explored its underlying mechanisms. We present the following article in accordance with the ARRIVE guideline checklist (available at http://dx.doi.org/10.21037/atm-20-3066).
Methods
Primary materials and chemicals
Lidocaine (molecular formula: C14H22N2O; molecular weight: 234.34; purity: 99%) was obtained from TCI-Europa (Eschborn, Germany). All antibodies were obtained from Abcam (Cambridge, UK).
Animals
Male healthy Sprague–-Dawley (SD) rats (6–8 weeks old, 200±20 g) were purchased from the Institute of Zoology, Chinese Academy of Sciences (Beijing, China). Eighty rats were housed in appropriate conditions (22±2 °C, humidity of 55%±5%, and 12 h light/dark cycle), and had free access to food and water. The protocols were performed in accordance with Declaration of Helsinki and approved by the ethics committees at Sichuan Academy of Medical Sciencesour institute .
Animal model
As previously described (18), (CI/R) was induced by middle cerebral artery occlusion (MCAO) in rats. Rats were randomly divided into 5 groups (n=8): control, model, CI/R + lidocaine 1 mg/kg, CI/R + lidocaine 2.5 mg/kg, and CI/R + lidocaine 5 mg/kg. Rats were anesthetized with pentobarbital sodium (42 mg/kg). Following complete loss of consciousness, the right common carotid artery was exposed by a midline cervical incision. Then, an A4-0 nylon filament with a circular tip was inserted from the common carotid artery to the end of the internal carotid artery to block the origin of the right middle cerebral artery. After 2-h occlusion, the nylon filament was carefully removed to enable middle cerebral artery (MCA) reperfusion for 24 h. For the sham group, the rats received the same operation. Under deep anesthesia with 2% pentobarbital sodium, rats were killed, and brain tissues were collected and stored at −80 °C.
CI/R rat group
Another 40 rats with CI/R lasting 2 h were randomly divided into 5 groups (subcutaneous injection once a day for 7 days): control, model, CI/R + lidocaine 5 mg/kg, CI/R + lipopolysaccharide (LPS) 10 mg/kg, and CI/R + LPS + lidocaine. LPS was used as a NF-κB signaling pathway activator (19).
Hematoxylin and eosin (HE) staining
Briefly, rat brain tissues were fixed in 4% paraformaldehyde buffer for 2 h. The specimens were continuously cut into 4 µm thick slices after embedding in paraffin. Next, slices were stained with hematoxylin for 5 min, and eosin for 3 min. Finally, the slices were dehydrated and blocked for pathological diagnosis in hippocampal cells.
Morris water maze test
We conducted the Morris water maze test to evaluate the spatial learning and memory ability of rats. As Zhou et al. describe (20), the experiment consists of a circular water pool, 120 cm in diameter and 50 cm in height. Briefly, the water temperature is kept at 22±2 °C, and a concealed underwater platform is set 2 cm below the water surface. In the study, 3 swimming tests for each rat were performed in the morning and afternoon for 4 consecutive days. On the fifth day, the platform was removed, and the rats were put into the water maze for 120 s (rats were allowed up to 120 s to reach the platform), and then the swimming distance and crossing times were measured.
Measurement of infarct volume
Rats were immediately decapitated after reperfusion, and the brain was rapidly frozen at −80 °C for 20 min. The brain was cut into sections 2.0 mm thick and incubated at 37 °C for 30 min in 2% 2,3,5-triphenyltetrazole chloride (TTC) solution and then fixed with 4% paraformaldehyde. Finally, the images were captured using a digital scanner (Canon EOS M2, Japan). The infarct volume from unstained areas was measured using Image J analysis software, and calculated as follows: Infarct volume (%) = (contralateral hemisphere area − non-infarction area of ipsilateral hemisphere)/contralateral hemisphere area) × 100%. The infarct volume was evaluated by an unknown examiner.
Measurement of brain water content
The brain water content was determined by the wet-dry method (21); 24 h after MCAO, all the rats were killed with chloral hydrate, and the brain was immediately excised. The wet weight of the brain was weighed on an electronic analytical scale and then dried in an oven at 110 °C for 24 h to obtain a dry weight. The brain water content was calculated as follows: brain water content = (wet weight-dry weight)/wet weight × 100%.
Evaluation of neurological deficit score
The neurological deficits score of rats were graded after 24 h of MCAO using Bederson neurological scale (22) in the following manner: 0 = no neurological deficits; 1 = failure to fully extend the left forepaw; 2 = reduced resistance to the left side push or circle when the rat is fixed on a flat surface, but normal posture at rest; 3 = spontaneous rotation to the left; 4 = no spontaneous movement or unconsciousness.
Real- time quantitative polymerase chain reaction (RT-qPCR)
Total RNA from hippocampi was extracted using a TRIzol reagent (Invitrogen, USA), and cDNA was synthesized with a Script RT Reagent kit (TaKaRa, Japan), according to the manufacturer’s instructions. The mRNA levels of relative genes were performed using SYBR Green Master mix (TaKaRa) and 1 µM primers by ProFlex™ PCR (Thermo Scientific, USA). β-actin was used as the endogenous control.
Apoptosis of hippocampal tissue
In brief, fresh brain tissue was fixed with paraformaldehyde overnight, processed, and embedded in paraffin. Brain slices were cut along the coronal surface to 5-µm thick and routinely dewaxed to water. The specimens were then stained in Nissl (Cresyl violet) Staining Solution (Solarbio, Beijing, China) for 1 h at 56 °C. Finally, the neuronal density of hippocampal CA1 pyramidal cells was examined using a light microscope.
Enzyme-linked immunosorbent assay (ELISA) assay
After lidocaine treatment was conducted as described above, serum was collected after centrifugation for 10 min (4 °C; 2,000 g). Serum levels of related proteins were detected using enzyme-linked immunosorbent assay (ELISA) kits (MSKBIO, Wuhan, China). The operational steps were performed according to the manufacturer’s instructions, and the results at 450 nm were recorded.
Western blotting
Total proteins were lysed with RIPA Lysis and Extraction Buffer (Thermo Fisher Scientific, Waltham, MA, USA), incubated on ice for 15 min, and centrifuged at 10,000 g for 10 min. BCA kit (Abcam, Cambridge, UK) was used to measure the protein concentration. Afterwards, protein samples were split by 12% SDS-PAGE, then transferred to polyvinylidene difluoride membrane (PVDF) (Thermo Fisher Scientific, Waltham, MA, USA). Next, the membranes were blocked with 5% non-fat milk at 37 °C for 1 h, and subsequently incubated with the matching primary antibody at 4 °C overnight. β-actin was represented as the internal control. After being fully cleaned 3 times, the membranes were incubated with goat anti-rabbit antibody coupled with horseradish peroxidase (HRP) for 2 h at 37 °C. Finally, the protein blotting was visualized with enhanced chemiluminescence (ECL) reagent (Beyotime, Haimen, China).
Statistical analyses
All operations are repeated at least 3 times, and the results are represented as mean ± SD. Data were analyzed using SPSS 21.0 (IBM, Armonk, NY, USA). The comparison between the 2 groups was performed using Student’s t-test, while comparison among the multiple groups was conducted using one-way analysis of variance (ANOVA) followed by Dunnett’s post hoc test. P values <0.05 were considered statistically significant.
Results
Lidocaine improves spatial learning and memory impairment in rats after CI/R
We first performed a Morris water maze test to examine the learning and memory abilities in rats. As shown in Figure 1, the escape latency of all rats was gradually shortened during the test, and there were significant differences between the groups. Compared with the control group, the escape latency in the CI/R group was significantly extended (P<0.05). Compared with the CI/R group, the lidocaine treatment significantly reduced the escape latency in rats (P<0.05).
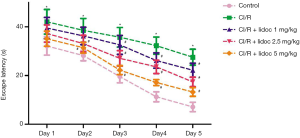
Lidocaine protected brain injury after I/R
In order to assess the extent of brain injury after CI/R, we observed morphological changes in hippocampal cells by HE staining. As shown in Figure 2A, the neurons in the control group were closely arranged and the structure was clear, and, while the neurons in the CI/R group were disordered, the cytoplasm was loose and the nucleus was contracted. Notably, with the increase of lidocaine concentration (1, 2.5, and 5 mg/kg), the number of cell layers increased and neuronal damage was restored in the hippocampus. Furthermore, TTC staining was employed to detect cerebral infarction. As shown in Figure 2B, no infarction was observed in the control group. Compared with the control group, I/R injury in the model group had significant infarction (58.69%±9.27%, P<0.05). However, lidocaine treatment (1, 2.5, and 5 mg/kg) reduced the area of infarction to 41.78%±6.36%, 20.64%±5.78%, and 17.53%±5.14%, respectively (P<0.05).
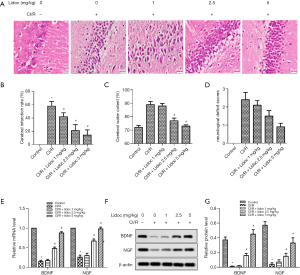
Lidocaine exerted neuroprotective effects against CI/R injury
We also measured the effects of lidocaine on brain water content and neurological deficit scores. As shown in Figure 2C, the brain edema in rats 24 h after I/R was more obvious than that in the control group (88.64%±0.54% vs. 72.31%±0.28%, P<0.05), and lidocaine (1, 2.5, and 5 mg/kg) reduced the water content of the cerebral hemisphere (86.18%±0.37%, 77.34%±0.46%, and 73.22%±0.19%, respectively, P<0.05), compared with the CI/R group. In similar fashion, compared with the control group, the score of the I/R group was significantly increased (2.4±0.56, P<0.05), while after lidocaine treatment, the scores were significantly decreased (2.1±0.72, 1.5±0.65, and 0.9±0.52, respectively), compared with the CI/R group (Figure 2D, P<0.05).
Lidocaine inhibited neuronal death and apoptosis in the hippocampus after CI/R
To determine the effect of lidocaine on neuronal death in the hippocampus, we detected neuronal apoptosis using Cresyl violet staining. As shown in Figure 3A, the control had many deeply stained round cells and intact Nissl bodies, but the number of cells in the CI/R group was sharply reduced. Compared with the control group, the number of Nissl bodies were markedly reduced (P<0.05), which is consistent with previous studies (23). Nevertheless, in the CI/R + lidocaine group, lidocaine improved Nissl body abnormality and significantly increased the number of living neurons (P<0.05). In addition, we examined the protein levels of cleaved-caspase-3, Bcl-2, Bax, and c-Myc by western blot. The results showed that the expression of cleaved-caspase-3 and Bax were increased, while the levels of Bcl-2 and c-Myc were decreased, with lidocaine effectively reversing this appearance (Figure 3B, P<0.05). These data suggested that lidocaine effectively attenuated I/R-induced neuronal death.
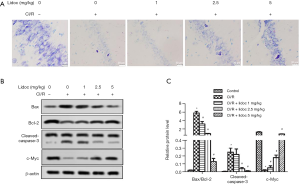
The expression of oxidative stress markers was detected by ELISA assay at 24 h after I/R. Compared with the control, the levels of superoxide dismutase (SOD) and glutathione (GSH) were significantly increased in the rats. Conversely, the malondialdehyde (MDA) level was decreased (P<0.05). After lidocaine treatment, the expression of SOD and GSH was increased while the expression of MDA was decreased (Figure 4A, P<0.05). Moreover, the expression of inflammatory factors were detected by western blot assay. The data showed that, compared with the control group, the IL-6 level in the CI/R was elevated while the level of IL-10 was reduced (P<0.05). Excitingly, lidocaine reversed this phenomenon (Figure 4B, P<0.05). Furthermore, the results of iNOS and IL-4 were consistent with IL-6 and IL-10, respectively (Figure 4C, P<0.05).
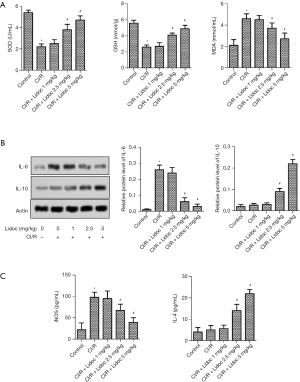
Lidocaine suppressed the activation of NF-κB p65 and p38 MAPK
To investigate the underlying mechanism of CI/R injury, we detected the level of p65 and p38 by western blotting (WB). As shown in Figure 5A, compared with the control group, CI/R injury promoted the expression of p65 and p38. However, lidocaine significantly decreased the levels of p65 and p38 expression (P<0.05).
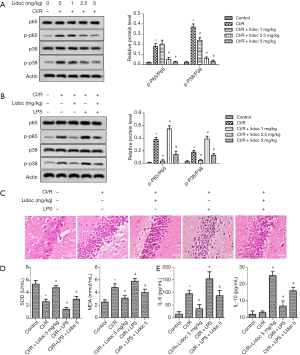
After adding LPS, the expression of p65 and p38 in the CI/R group was significantly higher than that in the control group (P<0.05). Compared with the CI/R group, the high expression of p65 and p38 in the CI/R + LPS group was more obvious. Importantly, the levels of p65 and p38 were significantly reduced both in the CI/R + lidocaine and CI/R + LPS + lidocaine groups (Figure 5B, P<0.05). In addition, the levels of SOD, MDA, IL-6, and IL-10 were reduced according to the ELISA assay. As shown in Figure 5C, compared with the control group, the levels of SOD and IL-10 were significantly decreased both in the CI/R and CI/R + LPS groups (P<0.05). Conversely, the MDA and IL-6 levels were increased. Similarly, lidocaine reversed the changes in these factors (P<0.05).
Discussion
With its high morbidity rates, CI/Rc is a serious threat to human health and survival. In recent years, lidocaine has been used in clinical cases to treat I/R injury (24). In this study, we adopted a rat model of CI/R injury induced by MCAO. We demonstrated that lidocaine protected CI/R injury in rats, and improved cognitive impairment, histopathological changes, and neuronal apoptosis. In addition, lidocaine remarkably attenuated the exacerbation of inflammation and oxidative stress. These findings suggest that lidocaine protects against CI/R injury by mediating NF-κB p65 and p38 MAPK pathway.
As we know, the hippocampus is one of the important areas of learning and memory, and CI/R induces neuronal injury and death in the hippocampus (25). Importantly, cognitive impairment is strongly associated with the degree of hippocampal neuronal cell injury (26). In our study, the Morris water maze task confirmed that lidocaine improved spatial learning and memory impairment in rats. Most noteworthy, it is reported that apoptosis is also involved in I/R injury (27). Bcl-2 family proteins, including pro-apoptotic protein Bax and anti-apoptotic protein Bcl-2, are key factors in the occurrence of apoptosis (28,29). Bcl-2 or Bax promotes the release of cytochrome C or other inducing factors, triggering the caspase cascade (especially the activation of caspase-9 and caspase-3) and causing apoptosis (30). In this study, lidocaine significantly reduced the expression of caspase-3 and the ratio of Bax/Bcl-2, subsequently increasing the expression of c-Myc. In addition,hematoxylin and eosin (HE) and Nissl staining were used to evaluate the neuronal injury in the hippocampus. Our results indicate that lidocaine exerted neuroprotective effects.
Ischemia causes adverse cascade reactions in the body, such as inflammation, oxidative stress, and toxicity, eventually leading to neuronal cell death (31). Previous reports showed that excessive reactive oxygen species (ROS) induces oxidative stress in CI/R injury (32). MDA is a hallmark of oxidative stress and also the end product of lipid peroxidation (33). SOD and GSH are considered to be 2 central endogenous anti-oxidant enzymes which provide a protective effect against cellular apoptosis induced by oxygen free radicals (34,35). In our study, lidocaine treatment significantly inhibited MDA level and increased SOD and GSH contents, indicating that lidocaine reduced I/R-induced oxidative stress in rats. Similarly, I/R injury is known to trigger inflammatory response (36), leading to the release of pro-inflammatory factors (TNF-α, IL-1β, IL-6, etc.). To determine the relationship between I/R injury and inflammation, we measured the levels of IL-6, IL-10, iNOS, and IL-4 in rats. The data showed that the levels of IL-6 and iNOS were significantly reduced while the levels of IL-10 and IL-4 were increased in lidocaine-treated I/R injury. These results suggest that lidocaine protects from brain injury through an anti-inflammatory response.
Our study revealed that the expressions of p65 and p38 proteins in the I/R + lidocaine and I/R + LPS + lidocaine groups were decreased, the levels of SOD and IL-10 were significantly increased, and the MDA and IL-6 levels were decreased. Based on previous research, we knew that NF-κB signaling pathway participated in survival signal transmission after transient ischemia, and exhibited an obvious neuroprotective effect in the hippocampus and striatum (37). In addition, P38 signal was found to be stimulated in the early stage of cerebral ischemia, which mediated free radical damage, inflammation, cell apoptosis, and cytotoxicity (38). In other studies, NF-κB expression was found to trigger the production of abundant pro-inflammatory cytokines (TNF-α, IL-1, and IL-6), which aggravated brain damage after I/R (39). Under the stimulation of ultraviolet radiation or pro-inflammatory cytokines, p38 MAPK signaling pathway preferentially accumulated in the cytoplasm and accelerated neuronal death (40,41). Furthermore, lidocaine attenuated LPS-induced acute lung injury via inhibiting NF-κB activation (42), and also suppressed the upstream phosphorylation of p38 MAPK signaling pathway (43) in LPS-activated microglia. Combined with previous findings, these data imply that lidocaine can alleviate CI/R injury in rats by inhibiting the activation of p65 and p38 signal pathway.
In summary, lidocaine reduced cerebral injury in rats, attenuated hippocampal neuronal apoptosis, and improved cognitive ability by regulating inflammation and oxidative stress through the NF-κB p65 and p38 MAPK signaling pathway. Therefore, lidocaine may provide a new avenue for treatment of CI/R injury in the future.
Acknowledgments
Funding: None.
Footnote
Guideline Checklist: The authors have completed the ARRIVE guideline checklist. Available at http://dx.doi.org/10.21037/atm-20-3066.
Data Sharing Statement: Available at http://dx.doi.org/10.21037/atm-20-3066.
Conflicts of Interest: All authors have completed the ICMJE uniform disclosure form (available at http://dx.doi.org/10.21037/atm-20-3066). The authors have no conflicts of interest to declare.
Ethical Statement: The authors are accountable for all aspects of the work in ensuring that questions related to the accuracy or integrity of any part of the work are appropriately investigated and resolved. The protocols were performed in accordance with Declaration of Helsinki and approved by the ethics committees at Sichuan Academy of Medical Sciencesour institute (No. 20200037).
Open Access Statement: This is an Open Access article distributed in accordance with the Creative Commons Attribution-NonCommercial-NoDerivs 4.0 International License (CC BY-NC-ND 4.0), which permits the non-commercial replication and distribution of the article with the strict proviso that no changes or edits are made and the original work is properly cited (including links to both the formal publication through the relevant DOI and the license). See: https://creativecommons.org/licenses/by-nc-nd/4.0/.
References
- Flynn RW, MacWalter RS, Doney AS. The cost of cerebral ischaemia. Neuropharmacology 2008;55:250-6. [Crossref] [PubMed]
- Giebel B, Hermann DM. Identification of the right cell sources for the production of therapeutically active extracellular vesicles in ischemic stroke. Ann Transl Med 2019;7:188. [Crossref] [PubMed]
- Banerjee S, Williamson D, Habib N, et al. Human stem cell therapy in ischaemic stroke: a review. Age Ageing 2011;40:7-13. [Crossref] [PubMed]
- Liang Y, Xu J, Wang Y, et al. Inhibition of MiRNA-125b Decreases Cerebral Ischemia/Reperfusion Injury by Targeting CK2α/NADPH Oxidase Signaling. Cell Physiol Biochem 2018;45:1818-26. [Crossref] [PubMed]
- Zhao H, Sapolsky RM, Steinberg GK. Interrupting reperfusion as a stroke therapy: ischemic postconditioning reduces infarct size after focal ischemia in rats. J Cereb Blood Flow Metab 2006;26:1114-21. [Crossref] [PubMed]
- Thompson BJ, Ronaldson PT. Drug delivery to the ischemic brain. Adv Pharmacol 2014;71:165-202. [Crossref] [PubMed]
- Hollmann MW, Gross A, Jelacin N, et al. Local anesthetic effects on priming and activation of human neutrophils. Anesthesiology 2001;95:113-22. [Crossref] [PubMed]
- Adler DMT, Damborg P, Verwilghen DR. The antimicrobial activity of bupivacaine, lidocaine and mepivacaine against equine pathogens: An investigation of 40 bacterial isolates. Vet J 2017;223:27-31. [Crossref] [PubMed]
- Picardi S, Cartellieri S, Groves D, et al. Local anesthetic-induced inhibition of human neutrophil priming: the infuence of structure, lipophilicity, and charge. Reg Anesth Pain Med 2013;38:9-15. [Crossref] [PubMed]
- Ebel D, Lipfert P, Frässdorf J, et al. Lidocaine reduces ischaemic but not reperfusion injury in isolated rat heart. Br J Anaesth 2001;86:846-52. [Crossref] [PubMed]
- Chen MY, Li CH, Huang ZQ, et al. Protective effects of lidocaine injected into the hepatoduodenal ligament on warm ischemia-reperfusion injury to the rat liver. Chin Med J (Engl) 2004;117:275-9. [PubMed]
- Das KC, Misra HP. Prevention of reperfusion lung injury by lidocaine in isolated rat lung ventilated with higher oxygen levels. J Postgrad Med 2003;49:17-20. [Crossref] [PubMed]
- Szydlowska K, Tymianski M. Calcium, ischemia and excitotoxicity. Cell Calcium 2010;47:122-9. [Crossref] [PubMed]
- Pavlikova M, Kovalska M, Tatarkova Z, et al. Response of secretory pathways Ca(2+) ATPase gene expression to hyperhomocysteinemia and/or ischemic preconditioning in rat cerebral cortex and hippocampus. Gen Physiol Biophys 2011;30:S61-9. [Crossref] [PubMed]
- Zhang H, Li L, Sun Y, et al. Electroacupuncture could regulate the NF-κB signaling pathway to ameliorate theinflammatory injury in focal cerebral ischemia/reperfusion model rats. J Neurochem 2016;137:713-25.
- Blondeau N, Widmann C, Lazdunski M, et al. Activation of the nuclear factor-kappaB is a key event in brain tolerance. J Neurosci 2001;1:4668-477. [Crossref] [PubMed]
- Zhou J, Du T, Li B, et al. Crosstalk between MAPK/ERK and PI3K/AKT signal pathways during brain ischemia/reperfusion. ASN Neuro 2015;7:7. [Crossref] [PubMed]
- Gong L, Tang Y, An R, et al. RTN1-C mediates cerebral ischemia/reperfusion injury via ER stress and mitochondria-associated apoptosis pathways. Cell Death Dis 2017;8:e3080. [Crossref] [PubMed]
- Zhao H, Chen Z, Xie LJ, et al. Suppression of TLR4/NF-κB Signaling Pathway Improves Cerebral Ischemia-Reperfusion Injury in Rats. Mol Neurobiol 2018;55:4311-9. [PubMed]
- Zhou X, Zhang F, Hu X, et al. Inhibition of inflammation by astaxanthin alleviates cognition deficits in diabetic mice. Physiol Behav 2015;151:412-20. [Crossref] [PubMed]
- Guo W, Feng G, Miao Y, et al. Rapamycin alleviates brain edema after focal cerebral ischemia reperfusion in rats. Immunopharmacol Immunotoxicol 2014;36:211-23. [Crossref] [PubMed]
- Bederson JB, Pitts LH, Tsuji M, et al. Rat middle cerebral artery occlusion: evaluation of the model and development of a neurologic examination. Stroke 1986;17:472-6. [Crossref] [PubMed]
- Wang M, Qi DS, Zhou C, et al. Ischemic preconditioning protects the brain against injury via inhibiting CaMKII-nNOS signaling pathway. Brain Res 2016;1634:140-9. [Crossref] [PubMed]
- Cassutto BH, Gfeller RW. Use of intravenous lidocaine to prevent reperfusion injury and subsequent multiple organ dysfunction syndrome. J Vet Emerg Crit Care 2003;13:137-48. [Crossref]
- Leão AH, Medeiros AM, Apolinário GK, et al. Hippocampal-dependent memory in the plus-maze discriminative avoidance task: The role of spatial cues and CA1 activity. Behav Brain Res 2016;304:24-33. [Crossref] [PubMed]
- Bae EJ, Chen BH, Yan BC, et al. Delayed hippocampal neuronal death in young gerbil following transient global cerebral ischemia is related to higher and longer-term expression of p63 in the ischemic hippocampus. Neural Regen Res 2015;10:944-50. [Crossref] [PubMed]
- Jung YS, Oh AY, Park HP, et al. Postischemic administration of pravastatin reduces neuronal injury by inhibiting Bax protein expression after transient forebrain ischemia in rats. Neurosci Lett 2015;594:87-92. [Crossref] [PubMed]
- Cory S, Adams JM. The Bcl-2 family: regulators of the cellular life-or-death switch. Nat Rev Cancer 2002;2:647-56. [Crossref] [PubMed]
- Siddiqui WA, Ahad A, Ahsan H. The mystery of BCL2 family: Bcl-2 proteins and apoptosis: an update. Arch Toxicol 2015;89:289-317. [Crossref] [PubMed]
- Singla N, Dhawan DK. Zinc down regulates Apaf-1-dependent Bax/Bcl-2 mediated caspases activation during aluminium induced neurotoxicity. Biometals 2015;28:61-73. [Crossref] [PubMed]
- Rodrigo J, Fernández AP, Serrano J, et al. The role of free radicals in cerebral hypoxia and ischemia. Free Radic Biol Med 2005;39:26-50. [Crossref] [PubMed]
- Floyd RA. Role of oxygen free radicals in carcinogenesis and brain ischemia. FASEB J 1990;4:2587-97. [Crossref] [PubMed]
- Qin F, Liu YX, Zhao HW, et al. Chinese medicinal formula Guan-Xin-Er-Hao protects the heart against oxidative stress induced by acute ischemic myocardial injury in rats. Phytomedicine 2009;16:215-21. [Crossref] [PubMed]
- Tu Q, Wang R, Ding B, Zhong W, Cao H. Protective and anti-oxidant effect of Danshen polysaccharides on cerebral ischemia/reperfusion injury in rats. Int J Biol Macromol 2013;60:268-71. [Crossref] [PubMed]
- Niizuma K, Yoshioka H, Chen H, et al. Mitochondrial and apoptotic neuronal death signaling pathways in cerebral ischemia. Biochim Biophys Acta 2010;1802:92-9.
- Speyer CL, Ward PA. Role of endothelial chemokines and their receptors during inflammation. J Invest Surg 2011;24:18-27. [Crossref] [PubMed]
- Duckworth EA, Butler T, Collier L, et al. NF-kappaB protects neurons from ischemic injury after middle cerebral artery occlusion in mice. Brain Res 2006;1088:167-75. [Crossref] [PubMed]
- Piao CS, Kim JB, Han PL, et al. Administration of the p38 MAPK inhibitor SB203580 affords brain protection with a wide therapeutic window against focal ischemic insult. J Neurosci Res 2003;73:537-44. [Crossref] [PubMed]
- Harari OA, Liao JK. NF-κB and innate immunity in ischemic stroke. Ann N Y Acad Sci 2010;1207:32-40. [Crossref] [PubMed]
- Duch A, de Nadal E, Posas F. The p38 and Hog1 SAPKs control cell cycle progression in response to environmental stresses. FEBS Lett 2012;586:2925-31. [Crossref] [PubMed]
- Wang W, Tang L, Li Y, et al. Biochanin A protects against focal cerebral ischemia/reperfusion in rats via inhibition of p38-mediated inflammatory responses. J Neurol Sci 2015;348:121-5. [Crossref] [PubMed]
- Feng G, Liu S, Wang GL, et al. Lidocaine attenuates lipopolysaccharide-induced acute lung injury through inhibiting NF-kappaB activation. Pharmacology 2008;81:32-40. [Crossref] [PubMed]
- Yuan T, Li Z, Li X, et al. Lidocaine attenuates lipopolysaccharide-induced inflammatory responses in microglia. J Surg Res 2014;192:150-62. [Crossref] [PubMed]