Apelin/Elabela-APJ: a novel therapeutic target in the cardiovascular system
Introduction
Since the discovery of APJ receptor in 1993, increasing evidence showed that APJ is essential in embryonic and adult mammalian cardiovascular systems. Apelin induces extensive physiological functions, including vasodilation, energy metabolism, increasing myocardial contractility and angiogenesis via its receptor APJ (1-3). Although the physiological roles of the apelin-APJ system have been revealed by gene knockout mice, there are differences in phenotypes between apelin and APJ knockout mice. Subsequently, the discovery of Elabela (ELA)/Toddler, a new endogenous ligand of APJ, reasonably explained this phenotypic difference (4,5). In this review, we discussed recent progression of roles of apelin/ELA-APJ system in cardiovascular physiology and pathophysiology, as well as underlying mechanisms in development and cardiovascular disease. It is clarified that the system of apelin/ELA-APJ can be an important target for cardiovascular diseases treatment.
Apelin, ELA and APJ: structure and function
APJ is a member of the G protein-coupled receptor (GPCR) family, which is similar to angiotensin II type 1 receptor (AT1R) in distribution pattern and amino acid composition (6), but has no affinity with angiotensin II. APJ is widespread expression in eukaryotes and expresses in human embryonic and adult tissues including heart, vascular system (especially vessel endothelium), lung, adipose tissue, gastrointestinal tract, central nervous system, breast and placenta (7-11). Immunohistochemistry showed that APJ locates in vascular endothelial cells, smooth muscle cells (SMCs), and cardiomyocytes. APJ is activated by apelin isoforms and ELA families to induce the cardiac and vascular effect (12). Recently, Ma has reported the human complex of apelin-17-APJ crystal structure and it reveals that the apelin-17 adopts a lactam constrained curved two-site ligand binding mode. Structural comparisons with others suggested that peptides with high conformational flexibility may regulate receptors in a similar way (13). In terms of function, human genetic studies have shown that polymorphism of the APLNR gene is related to the occurrence of coronary artery disease (CAD) and hypertension (14,15).
In 1998, Tatemoto et al. extracted a polypeptide from the bovine stomach and identified it as the endogenous ligand of APLNR (16). The apelin gene is located on the X chromosome and encodes the precursor apelin. The precursor apelin contains 77 amino acids and is cut by endopeptidase to produce mature apelin, including apelin-36, 17, 13, 12 and (Pyr1)-apelin-13. However, the process of converting precursor apelin into mature subtypes by protease is not clear. Apelin distributed in blood circulation in a paracrine/autocrine manner, thus giving full play to endocrine function. Generally, short apelin isoforms seem to be dominant in circulation (1,17). The highest concentration of apelin-17 and (pyr) apelin-13 was found in the plasma of human and rat (17,18). Apelin activates APJ to produce multiple cardiovascular effects including angiogenesis, vasodilation, cardiac contractility due to the widespread distribution of apelin in the heart and blood vessels.
Apelin ever had been thought to be the only endogenous ligand of the APJ. Functional differences between apelin and APJ are now revealed by further research. Therefore, other ligands may exist for APJ. Chng et al. firstly identified a new APJ endogenous ligand in zebrafish embryos called ELA (4). A few months later, another group published the same peptide structure called “Toddler” (19). The elabela gene encodes precursor proteins containing 54 amino acids and is processed into the following subtypes: ELA-32, ELA-22, ELA-21 and ELA-11. ELA is highly expressed in embryos, but only exists in a few number of adult tissues, for example, heart and blood vessels (20-22) (Table 1). ELA circulates in the blood of humans and mice (22,23). ELA regulates embryonic cardiovascular development, angiogenesis, and self-renewal of embryonic stem cells. However, further work is needed to reveal the mechanisms.
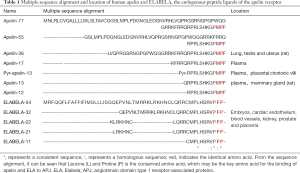
Full table
Apelin/ELA-APJ system regulates fetal cardiovascular development
ELA is important for the formation of the embryonic cardiovascular system and early placental development, and apelin regulates fetal neovascularization and energy homeostasis in the late pregnancy.
ELA-APJ participates in fetal heart development
APJ is essential for mouse embryos cardiovascular development. It is reported that APJ-knockout mice show part of embryonic fatal effect, and over fifty percent embryos die at 10. Five days of pregnancy. In addition, the great mass of survived embryos also have structural and functional abnormalities in the heart and blood vessels in adulthood, including insufficient vascular maturation and abnormal ventricular wall formation (24,25). Although Aplnr-deficient animals show embryonic fatal effect and cardiovascular defects, apelin knock out animals can survive and reproduce without serious heart defects (26-28). These studies show that in the early embryonic stage, the regulation of APJ on cardiac development is apelin-independent which maybe depend on ELA.
The vertebrate heart is produced during the gastrulation, and because the cardiac precursor converges from the mesoderm territory of the lateral plate to the midline of the embryo and extends to the nose to form a bilateral heart field. The low penetrance embryonic lethality caused by apela-deficient has many resemblances to those caused by Aplnr-deficient animals (29). ELA and APJ have very similar spatio-temporal expression patterns during embryonic development. On one hand, they were detected in the early stages of gastrulation, while apelin expression was only detected at the end stage. This is also a reasonable explanation for the effect of APJ on cardiac development independent of apelin. On the other hand, ELA knockout in spermatogonia impair cell movement during gastrulation and endoderm differentiation during embryogenesis (30,31). The differentiation of endoderm precursors is much important to guide the overlying cardiac progenitor cells towards the heart forming area (4). Myocardial progenitor cells migrate to the anterior lateral plate mesoderm (ALPM) and are exposed to the signals needed for cardiac development. Paskaradevan et al. demonstrated that the Aplnr-deficient resulted in myocardial progenitor cells cannot move to the cardiogenic area during gastrulation (28). In general, these results suggest that ELA activates APJ to promote endoderm differentiation and myocardial progenitor cell migration, then regulates fetal heart development.
Apelin/ELA-APJ regulates fetal vascular development
Vasculogenesis is the process of forming new primitive vascular network from hemangioblasts directly, while angiogenesis is the process of budding from existing blood vessels to form new blood vessel segments. The ELA-APJ is related to fetal angiogenesis. Using homologous recombination of ELA knockout mice, it was found that Apela-deficiency could inhibit embryonic vascular remodeling and inhibit the formation of organized yolk vessels, dorsal aorta, outflow tract, and interbody vessels. In addition, ELA knockout of spermatogonia boosts up the endothelial cell-specific molecule 1 (ESM1) expression in embryos too, which leads to angiogenic defects. Angioblasts migrate to the midline is a necessary condition for large axial vascular formation in the embryonic stage of vertebrates. They are the basis for the development and maturation of the cardiovascular system. ELA stimulating APJ further guides the migration of angiogenic cells to the midline. The mechanism may be that the ELA fragment-APJ complex induces receptor internalization by activating Gαi1 and β-inhibitor protein 2 signaling pathway (32). Internalized APJ inhibits the accumulation of cAMP, activates the phosphorylation of extracellular signal-regulated kinase (ERK)1/2, and inhibits intracellular calcium mobilization (20), and eventually induce vascular development.
It is known that ELA activates APJ to promote fetal vasculogenesis. APLNR is not expressed in human embryonic stem cells (hESCs). ELA can cause hESCs differentiate towards endoderm by enhancing the transforming growth factor-β (TGF-β) pathway (33). TGF-β is very important to endothelial and SMC proliferation, differentiation, migration, tube formation, and sprout. TGF-β binds with TGF-β receptor II (TβRII) to promote the phosphorylation of Smad2 and Smad3. And Smads induce endothelin and vascular endothelial growth factor (VEGF) expression in cooperation with other transcription factors, thus promoting vasculogenesis (34) (Figure 1). Therefore, ELA has been proposed to be an endogenous secreted growth factor for hESCs and human embryos that activate the TGF-β pathway to promote vasculogenesis.
The apelin-APJ axis is mainly responsible for the regulation of blood vessel diameter in the process of embryonic angiogenesis. Apelin is highly expressed in vascular endothelial cells of frog embryos. Cox et al. (35) showed that apelin increased the angiogenic branches of frog embryos and chicken chorioallantoic membrane systems. In addition, APJ is expressed on endothelial cells of the new blood vessels sprouted from the dorsal aorta in early embryogenesis. During embryogenesis apelin knock out mice exerted narrow blood vessels in intersomitic vessels. Apelin promoted endothelial cell proliferation mediated by VEGF and enhanced cell-to-cell aggregation (36). Due to the deficiency of vascular smooth muscle cells (VSMCs), the survived APJ-knockout embryos proved incomplete vascular maturation. This phenomenon may involve a new molecular mechanism. Apelin-APJ activates Gα13which cascade induces phosphorylation and cytoplasmic translocation of histone deacetylase (HDAC)4 and HDAC5, then leads to activation of cardiomyocyte enhancer factor 2 (MEF2) and promote fetal vascular development (37). After APJ knockout in mice, the endocardial HDAC4 and HDAC5 nuclei translocation was increased and the expression of MEF2 transcription factor Krüppel-like factor 2 (KLF2) was inhibited. However, as an epigenetic regulator, HDACs promote chromatin density and inhibits gene transcription by removing acetyl groups, and it contradicts MEF2 activation (Figure 1). HDACs up-regulate the expression of HIF-1α and VEGF and promote angiogenesis by inhibiting the expression of p53 and VHL (38). We think there are two possible reasons: (I) apelin-APJ caused HDAC4 and HDAC5 to transfer to cytoplasm and lost the transcriptional inhibition in the nucleus, and then played an “angiogenesis-like” role; (II) HDAC4 and HDAC5 activate MEF2 by inhibiting certain signal transcription.
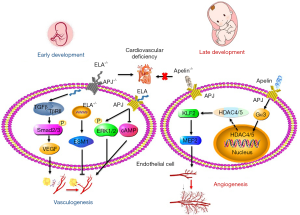
Apelin/ELA-APJ system and adult cardiovascular development
Up to now, the main studies of the apelin/ELA-APJ system are still in adult system, and the cardiovascular effects include VSMC proliferation, vasodilation, and positive myodynamia.
Apelin-APJ promotes VSMCs proliferation
At present, no report shows that ELA is associated with VSMC function. Our team has been devoted to studying apelin in VSMC proliferation. We firstly reported that apelin-13 remarkably promoted VSMC proliferation which accelerated the cell cycle process of apelin and promoted the transition of G0/G1 to S phase during mitosis. Apelin-p-ERK1/2-cyclin D1 was involved in the regulation of VSMC proliferation (39). PI3K/Akt is involved in cell cycle regulation. In 2010, Liu et al. further found that apelin-13 binded with APJ to stimulate PI3K phosphorylation and activated protein kinase Akt and its downstream signal molecule ERK1/2, then promoted the synthesis of cyclin D1 and eventually induced the proliferation of VSMC (40). Apelin-13 also upregulated early growth response factor-1 (Egr-1) through ERK signaling pathway to induce proliferation and migration of VSMC (41).
In 2011, Li et al. found that apelin-13 stimulated the expression of NADPH oxygenase 4 (NOX4) and promoted the production of reactive oxygen species (ROS). After interfering with NOX4 by RNA, the production of ROS and the proliferation of VSMC induced by apelin-13 were blocked, and the phosphorylation of ERK was reversed, which indicating that NOX4is an upstream signal molecule of ERK and participated in the promotion of VSMC proliferation by apelin-13 (42). In 2013, Li et al. further researched the downstream signal molecules of ERK. They found that Jagged-1/Notch3 between ERK and cyclin D1 was involved in the promotion of VSMC proliferation by apelin-13 (43). In a nutshell, apelin could promote the proliferation of VSMC, and the mechanism was that apelin activated PI3K/Akt and 14-3-3, which accelerated the transformation from G1 to S phase during mitosis and transmitted the signal from ERK to cyclin D1, then promoted VSMC proliferation. Mitophagy induced by overexpressing PINK1/Parkin promoted apelin-13-induced human aortic proliferation of VSMC by activating p-AMPKα and worsens atherosclerotic lesion progression (44). From the above, apelin-APJ facilitates proliferation of VSMC by accelerating the cell cycle process and PINK1/Parkin-mediated mitophagy (Figure 2).
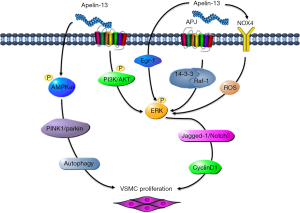
Apelin/ELA-APJ dilates blood vessels in endothelium-dependent and central nervous system-dependent manners
Previous studies linked the cardiovascular effects of apelin and ELA to vasodilation (45,46). Recent clinical studies have shown that apelin dilates the peripheral and coronary arteries in healthy people and heart failure (HF) patients and causes an increase in cardiac output (47,48). Apelin-13 induced vasodilation in intact endothelial vessels. The maximum relaxation was 79% at 10−6 M, but it reduced from 48% to 31% by removing of endothelium which indicating that apelin-13 induces vasodilation in an endothelium-dependent manner (20). The mechanism is that apelin is produced and released in vascular endothelial cells then binds to and activates adjacent APJ. Apelin-APJ activates endothelial nitric oxide synthase (eNOS) by activating L-arginine (L-Arg). Apelin-APJ stimulates the production and release of NO which increases the level of cyclic guanosine monophosphate (cGMP) in VSMC cells and realizes the vasodilation effect. Apelin is a powerful endothelial-dependent vasodilator that acts through a mechanism of prostanoid-dependence. Moreover, endothelial removal revealed direct vasoconstriction in both arteries and veins (49). After endothelial cell injury, the production of NOS is inhibited, which is the main reason why apelin constricts blood vessels and temporarily increases blood pressure during endothelial cell injury, which also involves the activation of protein kinase C (PKC) and the phosphorylation of myosin light chain (MLC) (50). This suggested that apelin dilated blood vessels in an endothelium-dependent manner.
ELA was found expression in heart, vascular endothelium and other peripheral organs in adult and showed cardiovascular profile through APJ as well as apelin. Animal studies have shown that ELA had more significantly systemic vasodilation reduction influence compared with apelin (32). A preliminary clinical study showed that ELA was negatively correlated with systolic and diastolic blood pressure and positively correlated with flow-mediated vasodilation (FMD). Stepwise multivariate linear analysis also found that plasma ELA level was closely related to endothelial function (51). Compared with apelin, ELA induces vasodilation after nitric oxide synthase inhibitor (flname) treatment (20). It indicated that ELA-mediated vasodilation does not require NO. However, how does ELA regulate vasodilation remained to be elucidated.
Apelin/ELA-APJ acts as a critical part in the positive inotropic effect
The high expression of apelin and ELA in the heart indicates that the apelin/ELA-APJ system may affect cardiac function. The infusion of apelin into an isolated perfused rat heart can induce a positive inotropic effect (52). In addition, exogenous apelin-12 exerts a selective positive inotropic action by increased [Ca(2+)](i) transients (53). In intact rat heart, the positive inotropic response of apelin was significantly decreased by selectively inhibiting the positive inotropic response of Na(+)/H(+) exchanger (NHE). Apelin promotes phospholipase C (PLC) to hydrolyze phosphatidylinositol to diacylglycerol and PKC activates NHE (Figure 3). In isolated cardiomyocytes, apelin significantly activated the sarcolemmal (NHE) and increased intracellular pH and myofilament sensitivity to Ca(2+) (54). Therefore, apelin plays a positive inotropic role by activating NHE.
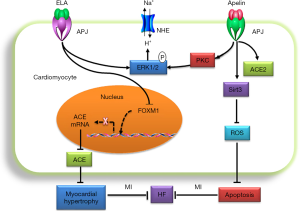
In mammalian hearts, ELA also showed positive inotropic function (55,56). In isolated rat hearts, nanomolar levels ELA increases cardiac contractility and expands coronary arteries by inducing ERK1/2 phosphorylation (5). Constant injection of ELA peptide greatly suppressed contractile damage due to pressure overload in mice. Mechanistically, ELA binds with APJ to downregulate transcription factors Forkhead Box M1 (FOXM1) transcription factor, then inhibits the expression of angiotensin-converting enzyme (ACE) instead of ACE2 in the stress heart, which is different from the influences of apelin. It is reported that activation of ERK1/2 by PKCδ leads to the human NHE2 transcriptional activity up-regulation in intestinal epithelial cells (57). Therefore, the different effects of ELA and apelin on the expression of ACE and ACE2 implicate fine-tuned mechanisms for a ligand-induced APJ activation and downstream signaling.
Apelin/ELA-APJ system is associated with cardiovascular diseases
ELA/ Apelin can be used to treat preeclampsia (PE)
PE is a severe pregnancy disease with an incidence of 5–7% worldwide (58). PE patients showed significant signs of hypertension after 20 weeks of gestation, usually accompanied by urinary protein and multiple organ dysfunction. Clinical symptoms of PE are connected to common endothelial dysfunctions like organ ischemia and vasoconstriction (59,60). ELA levels in pregnant women with late-onset PE were significantly lower than those in normal pregnancies. The mRNA and protein expressions of ELA and APJ in late-onset PE placental tissues are also decreased (61). The onset and cause of early and late eclampsia are different, but both are associated with placental function and perfusion. Early eclampsia is "exogenous" to the placenta, thus the cause of late-onset PE is ‘intrinsic’ to the growing and aging placenta (62). This may be the reason for the decreased expression of ELA and APJ in the placenta of late-onset PE. Ho et al. have also reported pregnant mice with ELA knockout showed PE like symptoms, including elevated blood pressure and proteinuria, that could be restored to normal by infusion of recombinant ELA (21). Furthermore, an in vitro study showed that the addition of ELA significantly increased the invasion ability and proliferation of trophoblast cells, which were inhibited by the APJ-specific antagonist ML221 (61). Therefore, ELA may protect against PE. Firstly, paracrine-ELA induces normal differentiation of endothelial tip cells and further maintains normal branching angiogenesis and an adequate labyrinth network formation. Secondly, ELA modulates cardiovascular function by stimulating vasodilatation to anti-hypertension.
Apelin can regulate placental vascular tension. It has been shown that the levels of Apelin and/or APJ mRNA and protein in the placenta of PE patients decreased (63,64), while the level of Apelin in maternal blood of PE increased (64-66). The mechanism is that PE-related maternal hypertension can induce the tissue and circulation level of apelin, which is beneficial to vasodilation to counteract maternal hypertension. The interesting thing is that chronic subcutaneous administration of apelin in rat PE model can reduce maternal hypertension and proteinuria and improve fetal growth. It suggested that the apelin/ELA-APJ axis may protect pregnant women against PE.
Exogenous apelin/ELA has antihypertensive effect
Apelin/ELA play role in antihypertension. Circulating ELA (51) and apelin (67) levels were decreased in patients with essential hypertension (EH) and general hypertension (GH), respectively. Intravenous injection of ELA-21 significantly decreased mean arterial pressure (MAP) in spontaneously hypertensive rats (SHRs) (68). Exogenous apelin could not only temporarily reduce the blood pressure of wild type mice, but also reduce the blood pressure of SHRs, but the roles of apelin were abolished in APJ-deficient mice. This apelin-induced response was inhibited by pretreatment with a nitric oxide synthase inhibitor, and apelin-induced phosphorylation of endothelial nitric-oxide synthase in endothelial cells from APJ-deficient mice disappeared (46). Apelin plays an antihypertensive role in both peripheral and central nervous system. In central nervous system, apelin activates APJ receptors in the hippocampal gyrus and hypothalamus which results in vasopressin release decrease and plasma adrenocorticotropin and adrenocorticotropin synthesis increase (69), then reduces blood pressure. In peripheral system, apelin regulate vascular tension and blood pressure in different ways. Apelin antagonizes the effect of Ang II on blood pressure (70,71). When Ang II amplifies vascular tension and aggravates hypertension, apelin acts as a vasodilator and antihypertension factor.
Apelin/ELA-APJ acts as a critical part in anti-HF
HF is the top reason for death around global countries because of its poor prognosis. Studies have demonstrated that apelin/APJ could be a target for the HF treatment. In the experimental model, such as decompensated HF induced by pressure overload, levels of apelin and APJ in circulation and myocardium were down-regulated in mice with decompensated HF (72,73). Apelin-deficiency increases cardiac dysfunction induced by pressure overload in mice (74). In humans, plasma apelin levels in HF patients remain unchanged or increased during the compensatory period, but are down-regulated during the compensatory period due to ischemic heart disease or idiopathic dilated cardiomyopathy (75,76). In contrast, heart resynchronization therapy can restore cardiac contractions and plasma apelin levels (77). This bipolar change indicates that apelin produced in the early stage of HF helps to maintain cardiac contractility and gradually maladapted, resulting in cardiac dysfunction in the later stage of HF. However, infusion of apelin into patients with HF can significantly increase cardiac output (48). Apelin also regulates HF caused by ventricular hypertrophy. Clinical data showed significantly lower serum apelin level in left ventricular hypertrophy (LVH) patient (78). Apelin-APJ signaling leads to increase Ca2+ sensitivity in the sarcomere without increasing Ca2+ transients, protect against the hypertrophy-HF transition (79). It suggests that apelin or APJ agonists can be used as therapeutic drugs in HF patients.
ELA, like apelin, antagonizes Ang II-induced cardiac damage and remodeling and exhibits potent cardioprotective effects in HF. Continuous infusion of ELA could significantly inhibit myocardial hypertrophy, fibrosis and contractility damage induced by pressure overload in mice. ELA administration decreased the mRNA expression of genes associated with HF and fibrosis. In APJ knockout mice, the cardioprotective effect of ELA was reduced, suggesting that ELA plays a cardiac protective role by activating APJ in the adult heart. In terms of mechanism, the ELA-APJ axis may protect HF caused by pressure overload by inhibiting the expression of FOXM1and ACE and pathogenic angiotensin II signal transduction (80). In summary, Apelin/ELA-APJ acts as a critical part in anti-HF
Apelin/ELA-APJ protects against myocardial infarction (MI)
Apelin is a protective factor for CAD such as MI. Good coronary artery collateral branches could reduce the area of MI. Studies have consistently shown that patients with decreased blood apelin levels have a higher incidence of adverse cardiac events after percutaneous coronary intervention (81). apelin knockout MI rats showed poor angiogenesis, remodeling, and functional repair after infarction, and induced accelerated death (82). In the MI rat model induced by coronary artery ligation, apelin-13 injection could promote myocardial angiogenesis, reduce MI size and fibrosis, and improve myocardial dysfunction (83,84), also remarkably decrease the mitochondrial damage and infarct size in I/R injured mice (85). Bone marrow cells (BMCs) stimulate myocardial angiogenesis and strengthen ischemic functional recovery through a paracrine mechanism (86). Apelin administration enhances the VEGF expression in bone marrow—derived mononuclear cells and promotes post-MI heart repair (84). Moreover, apelin treatment resulted in an important increase in expression of Sirtuin3 (Sirt3) and reduction of ROS formation. Apelin treatment further attenuated stress-induced apoptosis whereas knockout of Sirt3 abolished the anti-apoptotic effect of apelin in cultured BMCs (87,88). Moreover, apelin enhanced superoxide dismutase activity and phosphorylation of ERK1/2 and Akt, and inhibited oxidative stress and apoptosis of cardiomyocyte, thus recovered cardiac dysfunction caused by I/R injury (89). In conclusion, apelin-APJ may improve MI by inhibiting cardiomyocyte apoptosis.
The analysis of samples of infarcted mice heart showed that the expression of ELA and APJ receptor was induced in the hearts of mice with failure and was correlated with left ventricular ejection fraction (LVEF). ELA increased myocardial contractility and induced coronary artery dilatation and attended by an important increase in phosphorylation of ERK1/2. The inhibition of ERK1/2 significantly attenuated muscle strength induced by ELA (5). In addition, the Fc-ELA-21 fusion protein daily injection in MI rats for 4 weeks significantly mitigated heart dysfunction in regard to hemodynamics. In the cellular and tissue research, Fc-ELA-21 fusion protein treatment greatly promoted cardiomyocyte proliferation, increased angiogenesis, and reduced heart fibrosis & apoptosis near the infarct region (90). Consequently, ELA in the adult heart is upregulated by cardiac remodeling after infarction. ELA is concluded to increase cardiac contraction in ERK1/2 dependent way.
Apelin/ELA-APJ inhibits pulmonary arterial hypertension formation
Pulmonary arterial hypertension (PAH) is featured by endothelial injury, outer vascular modification, and vascular remodeling caused by irregular proliferation of pulmonary artery smooth muscle cells (PASMCs) in pulmonary vessels. Apelin is closely related to PAH, and plasma apelin level in PAH patients (91) and the expression of apelin in pulmonary vascular endothelial cells decreased (92,93). Compared with PAH induced by chronic hypoxia, PAH formed in apelin knockout mice was more serious. After blocking the apelin/APJ signal, the activation of AMPK, KLF2, and eNOS were inhibited and PAH deteriorated. Recent studies have shown that apelin/APJ can also target other genes involved in the pathological process of PAH, such as miR-424, miR-503, etc., and inhibit the activation of downstream signal FGF2 and block the formation of PAH with eNOS (93) (Figure 4). In addition to apelin, the expression of ELA decreased in cardiopulmonary tissue of PAH patients and rats. ELA treatment can significantly reduce right ventricular systolic blood pressure, improve right ventricular hypertrophy and pulmonary vascular remodeling in monocrotaline exposed rats (22). It has been shown that apelin/ELA can inhibit PAH formation and can be a potential target for PAH treatment.
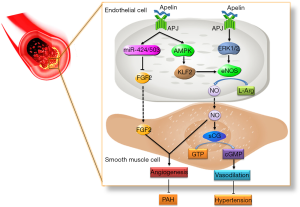
Other cardiovascular diseases related to apelin/ELA-APJ
Apelin/ELA-APJ system is also related to a variety of other cardiovascular diseases including sepsis-induced cardiac dysfunction and atherosclerosis. Apelin/ELA as an alternative to catecholamines is beneficial for patients in septic shock (94). In fatal sepsis-induced cardiac dysfunction by cecal ligation puncture, ELA or apelin-13 (39 and 15 µg/kg/hr, respectively) infusion can improve myocardial dysfunction and survival (95,96). Moreover, ELA is more superior than apelin-13 in improving fluid homeostasis and cardiovascular hemodynamic recovery, it possibly because full-length ELA uniquely regulates the release of vasopressin (96). Apelin is one of adipokines and associated with Type 1 diabetes mellitus (T1DM). Clinical research showed that serum apelin was significantly increased in T1DM patients. Carotid intima-media thickness (CIMT) has positive correlation with increased serum apelin level. Serum apelin may be a new marker of early atherosclerosis in children with T1DM (97). And, apelin-13 induced atherosclerotic plaque stimulates monocytes (MCs)-human umbilical vein endothelial cells (HUVECs) adhesion via ROS-autophagy pathway(98). Therefore, apelin/ELA-APJ system is a druggable target for sepsis-induced cardiac dysfunction and atherosclerosis.
Potential drugs are developed based on apelin/ELA
At present, many peptides have been developed based on apelin/ELA. These peptides can significantly improve the symptoms of cardiovascular disease. We have listed the peptides and small molecules targeting apelin/APJ in a review paper (99). In recent years, apelin has been used for some new indications, and new peptides of ELA structural homologs are being developed. Apelin peptides have beneficial effects on the cardiovascular system. However, apelin peptides have extremely short biological half-lives. So, improved understanding of apelin peptide metabolism may lead to the discovery of biologically stable analogues with therapeutic potential. Wang et al. designed and synthesized active apelin analogues (pyr-apelin 12 and 16) which were resistant to ACE2-mediated degradation. This makes an important step toward the development of long-lasting apelin receptor agonists (100). ELA (19-32), a peptide composed of amino acids at position 19-32 of ELA, binds to APJ and activates the β-arrestin-2 and Gαi1 signaling pathways, and then induces the internalization of receptors, which function like endogenous ELA. In addition, both ELA and ELA (19-32) reduce arterial pressure and have a positive inotropic effect on the heart (32). Preventing APJ internalization may be a novel strategy for hypertension and HF treatment. Another fusion protein of Fc-ELA-21 had a half-life roughly about 44 h in circulation in mice after subcutaneous injection and retained its functionality in vitro. Fusion protein injected daily in MI rats for four weeks greatly mitigated heart dysfunction with respect to hemodynamics. Treatment with Fc-ELA-21 fusion protein greatly reduced apoptosis and heart fibrosis near the infarct area and promoted cardiomyocyte proliferation and improved angiogenesis in vitro and in vivo (90), which suggests that Fc-ELA-21 may be a possible peptide drug for HF. Biphenyl acid derivatives compound 15a was demonstrated to have comparable potency with apelin-13 in vitro. In vivo, compound 15a improved the cardiac output in male Sprague Dawley rats in a dose dependent manner and may be beneficial for HF patients (101). Although these peptides have not been applicated in clinic, their high activity and selectivity to APJ and their homology with apelin/ELA indicates that they can be used as selective drugs for cardiovascular diseases therapy.
Conclusions and future perspectives
In this review, ELA-APJ/apelin related cardiovascular function, and cardiovascular illness was discussed. This provided the theoretical basis for cardiovascular diseases occurrence and progression and new targets for cardiovascular diseases treatment. What’s more, the roles of apelin/ELA-APJ in cardiovascular system associated diseases such as cerebral artery disease, hemangioma, arterial-venous disorganization are also being studied (102). More importantly, as saw in Aplnr-deficient mice, the discovery of ELA provides a good explanation for the absence of any abnormalities in cardiac development in apelin knockout mice. This may be helpful for us to understand the occurrence of some cardiovascular diseases from the source. As mentioned, apelin-APJ-related cardiovascular diseases have been studied comprehensively, but ELA-related ones are still rare. Therefore, systematic and comprehensive biological studies of ELA are still necessary to be performed. We should reveal how does ELA and apelin contribute and cross-regulate to the pathogenesis of heart disease in the future. In summary, this expanding knowledge of apelin/ELA-APJ systems will further encourage the therapeutic use of apelin, ELA and their derivatives.
Acknowledgments
Funding: This work was supported by grants from the National Natural Science Foundation of China (grant number: 81603192) and Science and Education Joint Project of Hunan Natural Science Foundation (grant number: 2018JJ5039).
Footnote
Conflicts of Interest: The authors have no conflicts of interest to declare.
Ethical Statement: The authors are accountable for all aspects of the work in ensuring that questions related to the accuracy or integrity of any part of the work are appropriately investigated and resolved.
Open Access Statement: This is an Open Access article distributed in accordance with the Creative Commons Attribution-NonCommercial-NoDerivs 4.0 International License (CC BY-NC-ND 4.0), which permits the non-commercial replication and distribution of the article with the strict proviso that no changes or edits are made and the original work is properly cited (including links to both the formal publication through the relevant DOI and the license). See: https://creativecommons.org/licenses/by-nc-nd/4.0/.
References
- De Mota N, Reaux-Le Goazigo A, El Messari S, et al. Apelin, a potent diuretic neuropeptide counteracting vasopressin actions through inhibition of vasopressin neuron activity and vasopressin release. Proc Natl Acad Sci U S A 2004;101:10464-9. [Crossref] [PubMed]
- Kasai A, Shintani N, Oda M, et al. Apelin is a novel angiogenic factor in retinal endothelial cells. Biochem Biophys Res Commun 2004;325:395-400. [Crossref] [PubMed]
- Dray C, Knauf C, Daviaud D, et al. Apelin stimulates glucose utilization in normal and obese insulin-resistant mice. Cell Metab 2008;8:437-45. [Crossref] [PubMed]
- Chng SC, Ho L, Tian J, et al. ELABELA: a hormone essential for heart development signals via the apelin receptor. Dev Cell 2013;27:672-80. [Crossref] [PubMed]
- Perjes A, Kilpio T, Ulvila J, et al. Characterization of apela, a novel endogenous ligand of apelin receptor, in the adult heart. Basic Res Cardiol 2016;111:2. [Crossref] [PubMed]
- O'Dowd BF, Heiber M, Chan A, et al. A human gene that shows identity with the gene encoding the angiotensin receptor is located on chromosome 11. Gene 1993;136:355-60. [Crossref] [PubMed]
- Hosoya M, Kawamata Y, Fukusumi S, et al. Molecular and functional characteristics of APJ. Tissue distribution of mRNA and interaction with the endogenous ligand apelin. J Biol Chem 2000;275:21061-7. [Crossref] [PubMed]
- O'Carroll AM, Selby TL, Palkovits M, et al. Distribution of mRNA encoding B78/apj, the rat homologue of the human APJ receptor, and its endogenous ligand apelin in brain and peripheral tissues. Biochim Biophys Acta 2000;1492:72-80. [Crossref] [PubMed]
- Medhurst AD, Jennings CA, Robbins MJ, et al. Pharmacological and immunohistochemical characterization of the APJ receptor and its endogenous ligand apelin. J Neurochem 2003;84:1162-72. [Crossref] [PubMed]
- Pope GR, Roberts EM, Lolait SJ, et al. Central and peripheral apelin receptor distribution in the mouse: species differences with rat. Peptides 2012;33:139-48. [Crossref] [PubMed]
- Mayeur S, Wattez JS, Lukaszewski MA, et al. Apelin Controls Fetal and Neonatal Glucose Homeostasis and Is Altered by Maternal Undernutrition. Diabetes 2016;65:554-60. [Crossref] [PubMed]
- Kleinz MJ, Skepper JN, Davenport AP. Immunocytochemical localisation of the apelin receptor, APJ, to human cardiomyocytes, vascular smooth muscle and endothelial cells. Regul Pept 2005;126:233-40. [Crossref] [PubMed]
- Ma Y, Yue Y, Ma Y, et al. Structural Basis for Apelin Control of the Human Apelin Receptor. Structure 2017;25:858-66.e4. [Crossref] [PubMed]
- Falcone C, Bozzini S, Schirinzi S, et al. APJ polymorphisms in coronary artery disease patients with and without hypertension. Mol Med Rep 2012;5:321-5. [PubMed]
- Kadoglou NP, Lampropoulos S, Kapelouzou A, et al. Serum levels of apelin and ghrelin in patients with acute coronary syndromes and established coronary artery disease--KOZANI STUDY. Transl Res 2010;155:238-46. [Crossref] [PubMed]
- Tatemoto K, Hosoya M, Habata Y, et al. Isolation and characterization of a novel endogenous peptide ligand for the human APJ receptor. Biochem Biophys Res Commun 1998;251:471-6. [Crossref] [PubMed]
- Zhen EY, Higgs RE, Gutierrez JA. Pyroglutamyl apelin-13 identified as the major apelin isoform in human plasma. Anal Biochem 2013;442:1-9. [Crossref] [PubMed]
- Azizi M, Iturrioz X, Blanchard A, et al. Reciprocal regulation of plasma apelin and vasopressin by osmotic stimuli. J Am Soc Nephrol 2008;19:1015-24. [Crossref] [PubMed]
- Pauli A, Norris ML, Valen E, et al. Toddler: an embryonic signal that promotes cell movement via Apelin receptors. Science 2014;343:1248636. [Crossref] [PubMed]
- Wang Z, Yu D, Wang M, et al. Elabela-apelin receptor signaling pathway is functional in mammalian systems. Sci Rep 2015;5:8170. [Crossref] [PubMed]
- Ho L, van Dijk M, Chye STJ, et al. ELABELA deficiency promotes preeclampsia and cardiovascular malformations in mice. Science 2017;357:707-13. [Crossref] [PubMed]
- Yang P, Read C, Kuc RE, et al. Elabela/Toddler Is an Endogenous Agonist of the Apelin APJ Receptor in the Adult Cardiovascular System, and Exogenous Administration of the Peptide Compensates for the Downregulation of Its Expression in Pulmonary Arterial Hypertension. Circulation 2017;135:1160-73. [Crossref] [PubMed]
- Panaitescu B, Romero R, Gomez-Lopez N, et al. ELABELA plasma concentrations are increased in women with late-onset preeclampsia. J Matern Fetal Neonatal Med 2020;33:5-15. [Crossref] [PubMed]
- Charo DN, Ho M, Fajardo G, et al. Endogenous regulation of cardiovascular function by apelin-APJ. Am J Physiol Heart Circ Physiol 2009;297:H1904-13. [Crossref] [PubMed]
- Kang Y, Kim J, Anderson JP, et al. Apelin-APJ signaling is a critical regulator of endothelial MEF2 activation in cardiovascular development. Circ Res 2013;113:22-31. [Crossref] [PubMed]
- Kasai A, Shintani N, Kato H, et al. Retardation of retinal vascular development in apelin-deficient mice. Arterioscler Thromb Vasc Biol 2008;28:1717-22. [Crossref] [PubMed]
- Zeng XX, Wilm TP, Sepich DS, et al. Apelin and its receptor control heart field formation during zebrafish gastrulation. Dev Cell 2007;12:391-402. [Crossref] [PubMed]
- Paskaradevan S, Scott IC. The Aplnr GPCR regulates myocardial progenitor development via a novel cell-non-autonomous, Galpha(i/o) protein-independent pathway. Biol Open 2012;1:275-85. [Crossref] [PubMed]
- Freyer L, Hsu CW, Nowotschin S, et al. Loss of Apela Peptide in Mice Causes Low Penetrance Embryonic Lethality and Defects in Early Mesodermal Derivatives. Cell Rep 2017;20:2116-30. [Crossref] [PubMed]
- Norris ML, Pauli A, Gagnon JA, et al. Toddler signaling regulates mesodermal cell migration downstream of Nodal signaling. Elife 2017.6. [PubMed]
- Sharma B, Ho L, Ford GH, et al. Alternative Progenitor Cells Compensate to Rebuild the Coronary Vasculature in Elabela- and Apj-Deficient Hearts. Dev Cell 2017;42:655-66.e3. [Crossref] [PubMed]
- Murza A, Sainsily X, Coquerel D, et al. Discovery and Structure-Activity Relationship of a Bioactive Fragment of ELABELA that Modulates Vascular and Cardiac Functions. J Med Chem 2016;59:2962-72. [Crossref] [PubMed]
- Ho L, Tan SY, Wee S, et al. ELABELA Is an Endogenous Growth Factor that Sustains hESC Self-Renewal via the PI3K/AKT Pathway. Cell Stem Cell 2015;17:435-47. [Crossref] [PubMed]
- Goumans MJ, Ten Dijke P. TGF-beta Signaling in Control of Cardiovascular Function. Cold Spring Harb Perspect Biol 2018.10. [PubMed]
- Cox CM, D'Agostino SL, Miller MK, et al. Apelin, the ligand for the endothelial G-protein-coupled receptor, APJ, is a potent angiogenic factor required for normal vascular development of the frog embryo. Dev Biol 2006;296:177-89. [Crossref] [PubMed]
- Kidoya H, Ueno M, Yamada Y, et al. Spatial and temporal role of the apelin/APJ system in the caliber size regulation of blood vessels during angiogenesis. EMBO J 2008;27:522-34. [Crossref] [PubMed]
- Kang Y, Kim J, Anderson JP, et al. Apelin-APJ Signaling Is a Critical Regulator of Endothelial MEF2 Activation in Cardiovascular Development. Circulation Research 2013;113:22-31. [Crossref] [PubMed]
- Qian DZ, Kachhap SK, Collis SJ, et al. Class II histone deacetylases are associated with VHL-independent regulation of hypoxia-inducible factor 1 alpha. Cancer Res 2006;66:8814-21. [Crossref] [PubMed]
- Li F, Li L, Qin X, et al. Apelin-induced vascular smooth muscle cell proliferation: the regulation of cyclin D1. Front Biosci 2008;13:3786-92. [Crossref] [PubMed]
- Liu C, Su T, Li F, et al. PI3K/Akt signaling transduction pathway is involved in rat vascular smooth muscle cell proliferation induced by apelin-13. Acta Biochim Biophys Sin (Shanghai) 2010;42:396-402. [Crossref] [PubMed]
- Liu QF, Yu HW, Sun LL, et al. Apelin-13 upregulates Egr-1 expression in rat vascular smooth muscle cells through the PI3K/Akt and PKC signaling pathways. Biochem Biophys Res Commun 2015;468:617-21. [Crossref] [PubMed]
- Li L, Li F, Li F, et al. NOX4-Derived Reactive Oxygen Species Drive Apelin-13-Induced Vascular Smooth Muscle Cell Proliferation via the ERK Pathway. International Journal of Peptide Research & Therapeutics 2011;17:307-15. [Crossref]
- Li L, Li L, Xie F, et al. Jagged-1/Notch3 signaling transduction pathway is involved in apelin-13-induced vascular smooth muscle cells proliferation. Acta Biochim Biophys Sin (Shanghai) 2013;45:875-81. [Crossref] [PubMed]
- He L, Zhou Q, Huang Z, et al. PINK1/Parkin-mediated mitophagy promotes apelin-13-induced vascular smooth muscle cell proliferation by AMPKalpha and exacerbates atherosclerotic lesions. J Cell Physiol 2019;234:8668-82. [Crossref] [PubMed]
- Lee DK, Cheng R, Nguyen T, et al. Characterization of apelin, the ligand for the APJ receptor. J Neurochem 2000;74:34-41. [Crossref] [PubMed]
- Ishida J, Hashimoto T, Hashimoto Y, et al. Regulatory roles for APJ, a seven-transmembrane receptor related to angiotensin-type 1 receptor in blood pressure in vivo. J Biol Chem 2004;279:26274-9. [Crossref] [PubMed]
- Barnes GD, Alam S, Carter G, et al. Sustained cardiovascular actions of APJ agonism during renin-angiotensin system activation and in patients with heart failure. Circ Heart Fail 2013;6:482-91. [Crossref] [PubMed]
- Japp AG, Cruden NL, Barnes G, et al. Acute cardiovascular effects of apelin in humans: potential role in patients with chronic heart failure. Circulation 2010;121:1818-27. [Crossref] [PubMed]
- Maguire JJ, Kleinz MJ, Pitkin SL, et al. [Pyr1]apelin-13 identified as the predominant apelin isoform in the human heart: vasoactive mechanisms and inotropic action in disease. Hypertension 2009;54:598-604. [Crossref] [PubMed]
- Japp AG, Cruden NL, Amer DA, et al. Vascular effects of apelin in vivo in man. J Am Coll Cardiol 2008;52:908-13. [Crossref] [PubMed]
- Li Y, Yang X, Ouyang S, et al. Declined circulating Elabela levels in patients with essential hypertension and its association with impaired vascular function: A preliminary study. Clin Exp Hypertens 2020;42:239-43. [Crossref] [PubMed]
- Szokodi I, Tavi P, Foldes G, et al. Apelin, the novel endogenous ligand of the orphan receptor APJ, regulates cardiac contractility. Circ Res 2002;91:434-40. [Crossref] [PubMed]
- Dai T, Ramirez-Correa G, Gao WD. Apelin increases contractility in failing cardiac muscle. Eur J Pharmacol 2006;553:222-8. [Crossref] [PubMed]
- Farkasfalvi K, Stagg MA, Coppen SR, et al. Direct effects of apelin on cardiomyocyte contractility and electrophysiology. Biochem Biophys Res Commun 2007;357:889-95. [Crossref] [PubMed]
- Yang P, Maguire JJ, Davenport AP. Apelin, Elabela/Toddler, and biased agonists as novel therapeutic agents in the cardiovascular system. Trends Pharmacol Sci 2015;36:560-7. [Crossref] [PubMed]
- Kuba K, Sato T, Imai Y, et al. Apelin and Elabela/Toddler; double ligands for APJ/Apelin receptor in heart development, physiology, and pathology. Peptides 2019;111:62-70. [Crossref] [PubMed]
- Muthusamy S, Shukla S, Amin MR, et al. PKCdelta-dependent activation of ERK1/2 leads to upregulation of the human NHE2 transcriptional activity in intestinal epithelial cell line C2BBe1. Am J Physiol Gastrointest Liver Physiol 2012;302:G317-25. [Crossref] [PubMed]
- Mol BWJ, Roberts CT, Thangaratinam S, et al. Pre-eclampsia. Lancet 2016;387:999-1011. [Crossref] [PubMed]
- Mor A, Fuchs Y, Zafra K, et al. Acute presentation of gestational diabetes insipidus with pre-eclampsia complicated by cerebral vasoconstriction: a case report and review of the published work. J Obstet Gynaecol Res 2015;41:1269-72. [Crossref] [PubMed]
- Bhorat I. An integrated model of materno-foetal cardiac dysfunction in severe pre-eclampsia. Cardiovasc J Afr 2019;30:181-83. [Crossref] [PubMed]
- Zhou L, Sun H, Cheng R, et al. ELABELA, as a potential diagnostic biomarker of preeclampsia, regulates abnormally shallow placentation via APJ. Am J Physiol Endocrinol Metab 2019;316:E773-E781. [Crossref] [PubMed]
- Staff AC, Redman CWG. The Differences Between Early- and Late-Onset Pre-eclampsia. In: Saito S, editor. Preeclampsia: Basic, Genomic, and Clinical. Singapore: Springer Singapore, 2018:157-72.
- Furuya M, Okuda M, Usui H, et al. Expression of angiotensin II receptor-like 1 in the placentas of pregnancy-induced hypertension. Int J Gynecol Pathol 2012;31:227-35. [Crossref] [PubMed]
- Inuzuka H, Nishizawa H, Inagaki A, et al. Decreased expression of apelin in placentas from severe pre-eclampsia patients. Hypertens Pregnancy 2013;32:410-21. [Crossref] [PubMed]
- Kucur M, Tuten A, Oncul M, et al. Maternal serum apelin and YKL-40 levels in early and late-onset pre-eclampsia. Hypertens Pregnancy 2014;33:467-75. [Crossref] [PubMed]
- Simsek Y, Celik O, Yilmaz E, et al. Serum levels of apelin, salusin-alpha and salusin-beta in normal pregnancy and preeclampsia. J Matern Fetal Neonatal Med 2012;25:1705-8. [Crossref] [PubMed]
- Xie H, Luo G, Zheng Y, et al. Lowered circulating apelin is significantly associated with an increased risk for hypertension: A meta-analysis. Clin Exp Hypertens 2017;39:435-40. [Crossref] [PubMed]
- Geng Z, Ye C, Tong Y, et al. Exacerbated pressor and sympathoexcitatory effects of central Elabela in spontaneously hypertensive rats. Am J Physiol Heart Circ Physiol 2020;318:H124-34. [Crossref] [PubMed]
- Newson MJ, Roberts EM, Pope GR, et al. The effects of apelin on hypothalamic-pituitary-adrenal axis neuroendocrine function are mediated through corticotrophin-releasing factor- and vasopressin-dependent mechanisms. J Endocrinol 2009;202:123-9. [Crossref] [PubMed]
- Yamamoto K, Ohishi M, Katsuya T, et al. Deletion of angiotensin-converting enzyme 2 accelerates pressure overload-induced cardiac dysfunction by increasing local angiotensin II. Hypertension 2006;47:718-26. [Crossref] [PubMed]
- Sun X, Iida S, Yoshikawa A, et al. Non-activated APJ suppresses the angiotensin II type 1 receptor, whereas apelin-activated APJ acts conversely. Hypertens Res 2011;34:701-6. [Crossref] [PubMed]
- Fukushima H, Kobayashi N, Takeshima H, et al. Effects of olmesartan on Apelin/APJ and Akt/endothelial nitric oxide synthase pathway in Dahl rats with end-stage heart failure. J Cardiovasc Pharmacol 2010;55:83-8. [Crossref] [PubMed]
- Pang H, Han B, Yu T, et al. Effect of apelin on the cardiac hemodynamics in hypertensive rats with heart failure. Int J Mol Med 2014;34:756-64. [Crossref] [PubMed]
- Kuba K, Zhang L, Imai Y, et al. Impaired heart contractility in Apelin gene-deficient mice associated with aging and pressure overload. Circ Res 2007;101:e32-42. [Crossref] [PubMed]
- Chong KS, Gardner RS, Morton JJ, et al. Plasma concentrations of the novel peptide apelin are decreased in patients with chronic heart failure. Eur J Heart Fail 2006;8:355-60. [Crossref] [PubMed]
- Miettinen KH, Magga J, Vuolteenaho O, et al. Utility of plasma apelin and other indices of cardiac dysfunction in the clinical assessment of patients with dilated cardiomyopathy. Regul Pept 2007;140:178-84. [Crossref] [PubMed]
- Francia P, Salvati A, Balla C, et al. Cardiac resynchronization therapy increases plasma levels of the endogenous inotrope apelin. Eur J Heart Fail 2007;9:306-9. [Crossref] [PubMed]
- Ye L, Ding F, Zhang L, et al. Serum apelin is associated with left ventricular hypertrophy in untreated hypertension patients. J Transl Med 2015;13:290. [Crossref] [PubMed]
- Parikh VN, Liu J, Shang C, et al. Apelin and APJ orchestrate complex tissue-specific control of cardiomyocyte hypertrophy and contractility in the hypertrophy-heart failure transition. Am J Physiol Heart Circ Physiol 2018;315:H348-56. [Crossref] [PubMed]
- Sato T, Sato C, Kadowaki A, et al. ELABELA-APJ axis protects from pressure overload heart failure and angiotensin II-induced cardiac damage. Cardiovasc Res 2017;113:760-9. [Crossref] [PubMed]
- Liu HT, Chen M, Yu J, et al. Serum apelin level predicts the major adverse cardiac events in patients with ST elevation myocardial infarction receiving percutaneous coronary intervention. Medicine (Baltimore) 2015;94:e449. [Crossref] [PubMed]
- Kidoya H, Takakura N. Biology of the apelin-APJ axis in vascular formation. J Biochem 2012;152:125-31. [Crossref] [PubMed]
- Zhang BH, Guo CX, Wang HX, et al. Cardioprotective effects of adipokine apelin on myocardial infarction. Heart Vessels 2014;29:679-89. [Crossref] [PubMed]
- Li L, Zeng H, Chen JX. Apelin-13 increases myocardial progenitor cells and improves repair postmyocardial infarction. Am J Physiol Heart Circ Physiol 2012;303:H605-18. [Crossref] [PubMed]
- Boal F, Timotin A, Roumegoux J, et al. Apelin-13 administration protects against ischaemia/reperfusion-mediated apoptosis through the FoxO1 pathway in high-fat diet-induced obesity. Br J Pharmacol 2016;173:1850-63. [Crossref] [PubMed]
- Crisostomo PR, Abarbanell AM, Wang M, et al. Embryonic stem cells attenuate myocardial dysfunction and inflammation after surgical global ischemia via paracrine actions. Am J Physiol Heart Circ Physiol 2008;295:H1726-35. [Crossref] [PubMed]
- Li L, Zeng H, Hou X, et al. Myocardial injection of apelin-overexpressing bone marrow cells improves cardiac repair via upregulation of Sirt3 after myocardial infarction. PLoS One 2013;8:e71041. [Crossref] [PubMed]
- Zeng H, He X, Hou X, et al. Apelin gene therapy increases myocardial vascular density and ameliorates diabetic cardiomyopathy via upregulation of sirtuin 3. Am J Physiol Heart Circ Physiol 2014;306:H585-97. [Crossref] [PubMed]
- Zeng XJ, Zhang LK, Wang HX, et al. Apelin protects heart against ischemia/reperfusion injury in rat. Peptides 2009;30:1144-52. [Crossref] [PubMed]
- Xi Y, Yu D, Yang R, et al. Recombinant Fc-Elabela fusion protein has extended plasma half-life andmitigates post-infarct heart dysfunction in rats. Int J Cardiol 2019;292:180-7. [Crossref] [PubMed]
- Chandra SM, Razavi H, Kim J, et al. Disruption of the apelin-APJ system worsens hypoxia-induced pulmonary hypertension. Arterioscler Thromb Vasc Biol 2011;31:814-20. [Crossref] [PubMed]
- Alastalo TP, Li M, Perez Vde J, et al. Disruption of PPARgamma/beta-catenin-mediated regulation of apelin impairs BMP-induced mouse and human pulmonary arterial EC survival. J Clin Invest 2011;121:3735-46. [Crossref] [PubMed]
- Kim J, Kang Y, Kojima Y, et al. An endothelial apelin-FGF link mediated by miR-424 and miR-503 is disrupted in pulmonary arterial hypertension. Nat Med 2013;19:74-82. [Crossref] [PubMed]
- Coquerel D, Sainsily X, Dumont L, et al. The apelinergic system as an alternative to catecholamines in low-output septic shock. Crit Care 2018;22:10. [Crossref] [PubMed]
- Chagnon F, Coquerel D, Salvail D, et al. Apelin Compared With Dobutamine Exerts Cardioprotection and Extends Survival in a Rat Model of Endotoxin-Induced Myocardial Dysfunction. Crit Care Med 2017;45:e391-8. [Crossref] [PubMed]
- Coquerel D, Chagnon F, Sainsily X, et al. ELABELA Improves Cardio-Renal Outcome in Fatal Experimental Septic Shock. Crit Care Med 2017;45:e1139-48. [Crossref] [PubMed]
- Sabry RN, El Wakeel MA, El-Kassas GM, et al. Serum Apelin: A New Marker of Early Atherosclerosis in Children with Type 1 Diabetes Mellitus. Open Access Maced J Med Sci 2018;6:613-7. [Crossref] [PubMed]
- Liu M, Li H, Zhou Q, et al. ROS-Autophagy pathway mediates monocytes-human umbilical vein endothelial cells adhesion induced by apelin-13. J Cell Physiol 2018;233:6839-50. [Crossref] [PubMed]
- Cao J, Li H, Chen L. Targeting drugs to APJ receptor: the prospect of treatment of hypertension and other cardiovascular diseases. Curr Drug Targets 2015;16:148-55. [Crossref] [PubMed]
- Wang W, McKinnie SM, Farhan M, et al. Angiotensin-Converting Enzyme 2 Metabolizes and Partially Inactivates Pyr-Apelin-13 and Apelin-17: Physiological Effects in the Cardiovascular System. Hypertension 2016;68:365-77. [Crossref] [PubMed]
- Su S, Clarke A, Han Y, et al. Biphenyl Acid Derivatives as APJ Receptor Agonists. J Med Chem 2019;62:10456-65. [Crossref] [PubMed]
- Kidoya H, Naito H, Muramatsu F, et al. APJ Regulates Parallel Alignment of Arteries and Veins in the Skin. Dev Cell 2015;33:247-59. [Crossref] [PubMed]