Ligustrazine nanoparticles nano spray’s activation on Nrf2/ARE pathway in oxidative stress injury in rats with postoperative abdominal adhesion
Introduction
Postoperative abdominal adhesion refers to the pathological connection between the omentum, abdominal organs and abdominal wall after surgery, which is a common postoperative complication with an incidence of more than 90% after open surgeries according to report (1-3). The main clinical manifestations of postoperative abdominal adhesion include intestinal obstruction, chronic abdominal pain and female infertility (4,5), which will make serious adverse effects on the second surgery (6). Although postoperative abdominal adhesion has been extensively studied, there has been neither effective treatments and interventions so far nor relevant authoritative treatment guidelines and definitive treatments, and the current treatment methods remain a great controversy (2). Therefore, it is always a hot spot in abdominal surgery research. The minimally invasive techniques such as laparoscopy and hysteroscopy are widely used in clinic and have greatly reduced the trauma caused by abdominal surgery. However, they cannot completely prevent postoperative abdominal adhesions (7).
The formation and mechanism of postoperative abdominal adhesions are so complex, that different drugs and materials are used in different stages to prevent its progress (8), including lavage of abdominal cavity (9), nonsteroidal anti-inflammatory drugs (10), double-layer electrostatic film made by hyaluronic acid (11), calcium channel blockers (12), thermosensitive injectable hydrogel (13) and green tea extract (14). However, there remains controversy in the efficacy of those agents preventing adhesion (15). In addition, most devices are limited in situations with incomplete hemostasis or bowel resection and consecutive anastomosis (16-18). Therefore, further study is needed to find more effective agents that could be safely used or could prevent and treat post-surgical peritoneal adhesion. Ligustrazine is an alkaloid extracted from the root of Rhizoma chuanxiong, a traditional Chinese herb. It is one of the active constituents of Rhizoma chuanxiong. Several studies have demonstrated the biological and pharmacological effects of ligustrazine such as inhibiting oxidation, improving microcirculation, and decreasing platelet aggregation and inflammation (19-21). Zhang et al. (22) reported that after the existing adhesions being loosened by surgery, 6 patients with adhesive intestinal obstruction, were treated with ligustrazine injection to the abdominal cavity for prevention. They were followed up for 1–3 years and no adhesion was found. Zhang et al. (23) observed the clinical effect of 40 mg ligustrazine diluted with 250 mL saline on 70 patients with abdominal surgery by peritoneal perfusion. Results showed that the recovery time of bowel sounds and the first exhaust and defecation time in the ligustrazine treatment group was obviously shortened, compared with the control group, and there was a significant difference in the effective rate and the overall graded efficacy ratio between the two groups.
According to the clinical trials and our previous research on the effect of ligustrazine on postoperative abdominal adhesions, it is found that ligustrazine can effectively inhibit abnormal fibrosis so as to prevent postoperative abdominal adhesions (24). However, ligustrazine, as an alkaloid, is characterized by fast absorption, metabolism and short half-life (25), leading to low bioavailability, thus its clinical application is limited. Our research group developed ligustrazine nano preparations according to the metabolic characteristics of ligustrazine. The use of nanotechnology increases the solubility of drug particles so as to improve bioavailability (26), and cover to isolate with the aid of polylactic acid (27). Ligustrazine nanoparticles nano spray (LNNS) is easy to use and characterized by targeted administration, high bioavailability, good biocompatibility and low cytotoxicity whereby effectively compensates for the short-term metabolism of ligustrazine. It is found that LNNS can inhibit TGF-β1/Smad signaling pathway so as to reduce peripheral blood TNF-α and IL-1β levels, lessens the proliferation of collagen I of adhesion tissue and increases levels of TGF-β1, FN, CTGF in order to balance t-PA and PAI-1 to further reduce the extent of peritoneal adhesions in rats (28,29).
Recent literature studies have shown that oxidative stress is an important pathological mechanism for postoperative abdominal adhesions (30). Reactive oxygen is a chemically reactive factor containing oxygen, a by-product of oxygen metabolism. The surgical trauma generated and foreign body invasion can cause a large amount of active oxygen produced by the body in a short time. When the accumulation of active oxygen exceeds the body’s ability to remove, it can lead to peroxidative damage to tissues and organs (31). Oxidative stress is closely related to pathological changes, inflammatory reaction, and immune and fibrinolysis system imbalance. Therefore, eliminating reactive oxygen species (ROS) is an important way to regulate antioxidant capacity of the body so as to prevent postoperative abdominal adhesions (30).
Based on the beneficial effects of ligustrazine described above, this study aimed to explore the effects of LNNS on inflammation and oxidative stress which are the two major pathological mechanisms involved in post-surgical peritoneal adhesion.
Methods
Drugs and chemicals
Ligustrazine (purity higher than or equal to 98%, No. JD-45630) was purchased from Shanghai Jingdu Biotechnology Co., Ltd. TNF-α and IL-1β rat ELISA kits were prepared from Nanjing Jiancheng Bioengineering, China. Sodium hyaluronate gel was purchased from Shandong Bausch & Lomb Pharmaceutical Co., Ltd.; while rabbit polyclonal NRF2 antibody (Abcam, USA), rabbit polyclonal to HO-1 antibody (Abcam, USA), rabbit polyclonal NQO1 antibody (Abcam, USA), TRIzon, Reagent, monoclonal Anti-β-Actin antibody, goat anti-mouse antibody, high-sensitivity chemiluminescence detection kit and the reverse transcription kit were all purchased from Thermo; the QPCR premix was purchased from Vazyme.
Animals
A total of 60 healthy 8-week-old male Sprague Dawley (SD) rats weighting of 250±25 g were purchased from Shanghai Jiesijie Experimental Animal Co., Ltd. They were acclimatized and housed in separated standard cages. The animal room was well ventilated with temperature of 21±2 °C and 60%±3% humidity, and was equipped with 12 h natural light-dark cycle. Rats had free access to standard tap water and laboratory pellet chow. Good hygiene was maintained by frequent cleaning and removal of feces and spilled feeds from cages daily. All animals received humane care in compliance with the guidelines of the China Council on Animal Care and Use. All animal procedures performed in this study were reviewed, approved, and supervised by the Institutional Animal Care and Use Committee of the Ethics Committee of Nanjing University of Chinese Medicine, China.
Preparation of LNNS
LNNS was defined using the method which previously described (28,29). In briefly, the ligustrazine and polylactic acid were dissolved in an acetone solution at a feed ratio of 1:4, 20 g/L as with the concentration of lactic acid in the acetone solution. This solution was used as the oil phase. While 0.25% poloxamer solution of 4 times the volume of the oil phase was used as the aqueous phase. The oil phase was injected into the aqueous phase at high-speed magnetic stirrer, and then kept stirred at constant temperature of 30 °C for 70 min to evaporate the acetone. After that 1 g/L of a ligustrazine nanoparticle solution with an average particle diameter of about 200 nm was obtained. Afterwards, the solution is filled, sealed, and radiated for sterilization to make a ligustrazine nanoparticle spray. The encapsulation efficiency of the prepared ligustrazine polylactic acid nanoparticles was stable. The nanoparticles observed under transmission electron microscopy were spherical-like matter whose surface was smooth and non-adhesive and particle size distribution was narrow with the average diameter of about 210 nm and the average diameter distribution of around 0.09 (32).
Model establishment
Rats were randomly divided into sham operation (sham) group, control group, sodium hyaluronate (HA) group and low dose LNNS (L-LNNS) group, medium dose LNNS (M-LNNS) group, and high dose LNNS (H-LNNS) group according to random number table, each with 10 rats. According to the previous study, the experimental abdominal adhesion model was established by needle files (29,33,34). The rats were fasted for 12 h but given free access to drinking water before the model established, and then they were intraperitoneally injected with 10% chloral hydrate (3.5 mL/kg). The rats were fixed in supine position, shaved and sterilized. Afterwards, 1.5 cm front median incision was made in the lower abdomen layer by layer. The assorted file repeatedly rubbed the serosal layer on the right ileocecal area until the surface appears spotted hemorrhage to form a 2.0 cm × 1.5 cm wound that was brought into the abdominal cavity after exposure in air for 5 min. The 3-0 sterile silk thread was sutured. In the sham operation group, there was no modeling and the abdominal cavity of rats was only sutured after being opened. In the control group, the abdomen was sutured after modeling, but no drug was given. While, in the low, medium and high dose groups, 2.5, 5, 10 mL/kg LNNS were applied to the wounds respectively after modeling. Besides, 1% sodium hyaluronate gel was used in the sodium hyaluronate groups (0.5 mL/kg) before closing the abdomen of models. After the model was established, the rats in each group were fasted for 24 h and then they were fed for 7 days, during which, their feeding, activity and wound healing were observed.
Macroscopic evaluation of adhesion score
Incision healing, abscess, general condition (body weight), incision and abdominal infection were observed after the operation, and animal’s mortality was recorded. Rats were fasted on the 8th day after surgery before euthanized by cervical dislocation. Next, “U” type incision was made under the xiphoid into the abdomen. The adhesion scores were evaluated according to a modified classification of Phillips, Bhatia grading standards from reported literatures (35,36) (Table 1) The levels of adhesion were scored as follows: 0= no adhesion; 1= single band of adhesions between viscera or from one viscus to the abdominal wall, spontaneously separating adhesion; 2= two bands, either between viscera or from viscera to the abdominal wall, separation of adhesion with traction; 3= more than two bands between viscera or viscera to the abdominal wall, or whole of intestines forming a mass, without being adherent to the abdominal wall, separation of adhesion with a blunt dissection; and 4= whole model area covered with adhesion, separation of adhesion with a sharp dissection. The scorers who have experience on peritoneal adhesions were arranged to different groups randomly.
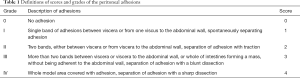
Full table
Histopathological evaluation
The tissue samples were dehydrated, embedded in paraffin, and stained with HE so as to observe inflammatory response and fibrosis. Fixed for 24 h, the injured cecum wall and the peritoneal adhesion tissue were embedded in paraffin. Then they were cut and divided into 5 µm paraffin sections. Histopathological changes in fibrosis and inflammation were observed under light microscope. According to the microscopic scoring standard (36) (Table 2), two pathological sections were evaluated by the researchers of the Department of Pathology, Nanjing University of Chinese Medicine. Six HE slices from each group were chosen. Five fields of each slice in view were randomly selected under light microscope. The images were taken with a color pathological image analysis system to calculate the degree of inflammation and fibrosis.

Full table
Masson’s trichrome stain for collagen deposition
Some paraffin sections were observed for by Masson’s trichrome stain, and the collagen fibers were stained blue. The staining was carried out according to the instruction of the Masson’s trichrome stain kit (Sigma-Aldrich, United States). The percentage of positive staining was assessed. Eight microscope fields were randomly selected by Image-Pro Plus 5.0 software (Leica, Germany) to measure the average collagen thickness in the adhesive tissues.
Detection of ROS, NO, SOD and MDA activity in peritoneal fluids
On the 8th day after operation, rats in each group were intraperitoneally injected with 0.5 mL normal saline. The peritoneal fluid was collected (abandoned if blood was contaminated) 0.5 h later. The fluid’s pH was adjusted to 3.9, and then centrifuged at 3,000 ×g for 5 min. The supernatant was taken and finally stored at 4 °C in refrigerator. The activity of ROS, nitric oxide (NO), superoxide dismutase (SOD) and malondialdehyde (MDA) in the sample was calculated following the kit instructions.
Enzyme-linked immunosorbent assays (ELISA)
Ten percent chloral hydrate (3.5 mL/kg) was intraperitoneally injected into rats in each group. Blood was collected from abdominal aorta and centrifuged at 3,000 ×g/min for 15 min at 4 °C. The serum was taken and stored in a refrigerator at −80 °C for testing. The concentrations of TNF-α and IL-1β in serum were evaluated using commercial ELISA kits (R&D Systems, USA) according to the manufacturer’s instructions. The optical density (OD) of samples were read on a microplate reader (Spectra Max190, Thermo Fisher, USA) with a wavelength of 450 nm. The OD value was plotted on the ordinate, and the concentration of the standard product was plotted on the abscissa to draw a graph. The corresponding concentration range was searched according to the OD value of the sample.
Immunohistochemistry
Tissue of each group were fixed with paraformaldehyde for 24 h and then they were embedded in paraffin. Immunohistochemical staining was used for the 4 µm sections, which were processed by conventional dewaxing, hydration, washing with double distilled water, antigen retrieval. They were then blocked at room temperature, followed by incubation of MCP-1 and MMP-9 primary antibodies overnight. The secondary anti-DAB was incubated at room temperature, counterstained with hematoxylin and differentiated with hydrochloric acid alcohol, dried, and coverslipped with mounting. The average OD of positive cells in each section was analyzed by an upright microscope, and the plasma membrane in brownish yellow was considered positive. Five intestine tissue sections with complete structure (10×10) were randomly selected for as application. The semi-quantitation of immunohistochemical staining was performed, and the intensity was scored as follows: 0, negative; 1, mild; 2, moderate; and 3, severe. The extent of staining was assessed based on the percentage of positive cells and was scored as follows: 0, negative; 1, 1–25%; 2, 26–50%; 3, 51–75%; and 4, 76–100%. The total staining scores (0–7) were determined by the score for staining intensity and extent for positive area in the adhesive tissue.
RT-PCR
Five hundred mg intestinal tissue of rat was taken on the ice. Five hundred mg of the colon 2 cm to the ileocecal area was cut out, in the event of adhesion absent. The tissue was then placed in a sterile cryotube kept in liquid nitrogen, and transferred to −80 °C for cryopreservation. Then, 100 mg of the tissue was taken from each group to extract total RNA by Trizon kit. The total RNA concentration in each sample was determined by Nanodrop 2000c with the value of A260/A208 between 1.8 and 2.0. The transcribed cDNA was subjected to a PCR with a reaction system of 20 µL [2× ChamQ SYBR qPCR Master Mix (High ROX Premixed) 10 µL, PCR Forward Primer (10 µmol/L) 0.4 µL, PCR Reverse Primer (10 µmol/L) 0.4 µL, cDNA template 0.5 µL (total 500 ng), ddH2O 8.7 µL]. The reaction conditions were set to 95 °C for 30s pre-denaturation, 95 °C for 10 s, 60 °C for 30s annealing (40 cycles). The reaction was designed with Primer Premier 5.0 software and synthesized by Nanjing Kingsray Biotechnology Co., Ltd. GAPDH was used as the internal reference primer. PCR primer sequences were as follows: GAPDH: forward 5'-ATCTCG CTC CTG GAA GAT GG-3', reverse 5'-CAA GTT CAACGG CAC AGT CA-3'; Nrf2: forward 5'-CCA TTT ACGGAG ACC CAC-3', reverse 5'-TCT GAG CGG CAA CTTTAT-3'; heme-oxygenase-1: forward 5'-ATG GAGCGC CCA CAG CTC GAC AGC A-3', reverse 5'-ACTGGGTTC TGC TTG TTT CG-3'; NQO1: forward 5'-GGGGACATGAACGTCATTCTCT-3', reverse 5'-AGTGGTGACTCCTCCCAGATGGTG-3'. The 2-ΔΔCt method was used to quantify the relative changes in mRNA.
Western blot analysis
One hundred mg tissue of the intestinal tube was taken from each group, and the relevant protein was extracted by homogenization with 1 mL of RIPA buffer. Qubit 2.0 was used to measure the protein content. The protein was separated by SDS-polyacrylamide gel electrophoresis, transferred to a PVDF membrane, and incubated with 5% skim milk powder PBST (PBS containing 0.05% Tween-20) for 1 h at 37 °C. Afterwards, the primary antibody was incubated overnight at 4 °C, and rinsed 3 times with PBST for 5 min each time. The secondary antibody was incubated for 1 h at room temperature and rinsed 3 times with PBST for 5 min each time. The signals were detected by ECL kit, imaging was made by chemiluminescence imager, and bands were analyzed by Image Lab 5.0.
Statistical analysis
The data was analyzed using SPSS 19.0 software. The measurement data were expressed as mean ± standard deviation, consistent with normality and homogeneity of variance. The one-way ANOVA compared the means among groups. LSD was used for comparison between groups. Kruskal-Wallis rank sum test for sample nonparametric rank sum test. P<0.05 was considered significant.
Results
General observation
One rat in the control group died of the intra-abdominal infection 5 days after scraping because the contents spilled out from intestines. Another rat in high dose LNNS group died of preoperative anesthesia. Abdominal incision of all the other rats healed well without swelling or incisional hernia. Rats in each group were in poor condition in general 24 hours after surgery. They were inactive, taking little food and water. While, two days later found the rats more active, taking food and water as normal. On the third day after surgery, the suture of the abdominal wound gradually fell off. The rats’ activity and diet recovered.
Macroscopic adhesion evaluation
As shown in Figure 1, compared with the sham operation group, abdominal adhesions of the control group were tight without cracks in general, only a few adhesions were thickened and expanded, but their toughness was increased, and some adhesions were occasionally associated with blood vessels. In the sodium hyaluronate group and LNNS groups, adhesions were reduced and loosened at different degrees, and no angiogenesis was observed. In particular, the high dose group showed a loose film-like adhesion in the cecal area of the filing part. Abdominal adhesion grades of the groups were shown in Figure 1. Compared with the sham group, the abdominal adhesion grade of the control group was significantly higher than that of the sham operation group, and the difference between the groups was statistically significant (P<0.01). Compared with the control group, the intraperitoneal adhesion grade of LNNS groups all decreased. Abdominal adhesion grade fell as dose of LNNS increased, with significant difference (P<0.05 or P<0.01).
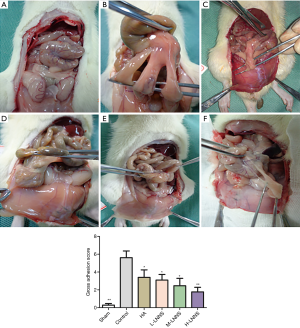
Histologic adhesion scores
It is shown in Figure 2 that no abnormal changes in the villus epithelium of the sham operation group were observed under the light microscope, and there were no hyperemia or inflammation in the mucosa, submucosa, muscle layer and serosal tissue. The blood vessels in the intestinal wall of the control group were highly dilated and edematous. Lymphocyte infiltration and fibroblast proliferation were observed in the serosa layer. Some microabscesses formed by small focal neutrophil accumulation were observed. Edema was observed in the sodium hyaluronate group, with infiltrating lymphocytes and other inflammatory cells as well as serosal fibrosis. The muscle layer structure of LNNS groups was intact, and the serosa layer was slightly thickened. In the low dose group intestinal mucosa, submucosal congestion and edema, inflammatory cell infiltration were found; while in the medium dose group, there were mild congestion, edema in the serosa layer and connective tissue hyperplasia of the serosal surface; fibroblasts and capillary proliferation were observed in the high dose group. Figure 2D,E,F demonstrated that the inflammation and fibrosis scores of the low, medium and high doses of LNNS groups were lower than those of the control group which was statistically significant (P<0.05). It is suggested that all the LNNS groups reduced the inflammation and fibrosis scores of the adhesions.
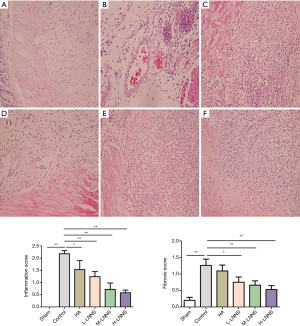
LNNS reduces collagen synthesis in adhesive tissue
Masson’s trichrome stain is a classic collagen fiber staining method in which the blue portion is considered to be a collagen fiber, while muscle fiber is shown in pale red. As shown in Figure 3, it was found that the sham operation group had little pale-blue collagen tissue; the subcutaneous connective tissue of the control group showed a deep blue broad distribution with the intestinal wall or pulp as well as tightly bound membrane, suggesting there were a large amount of collagen fibers. In the sodium hyaluronate group and the low, medium and high doses of LNNS groups, it showed a thin, light blue local distribution, which indicates a decrease in collagen fibrosis (P<0.05). The most obvious decrease of collagen thickness occurred in the high dose group.
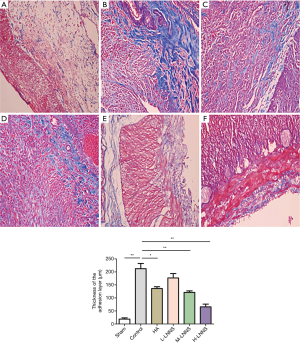
Effects of LNNS on the activity of ROS, SOD, MDA and NO in peritoneal fluid of rats
Compared with the sham operation group, levels of ROS, MDA and NO in the peritoneal fluid of the control group were significantly increased (P<0.01); while the expression of SOD decreased (P<0.01). SOD of LNNS groups increased, but their ROS, MDA and NO were significantly lower than those of the control group (P<0.05), as shown in Figure 4.
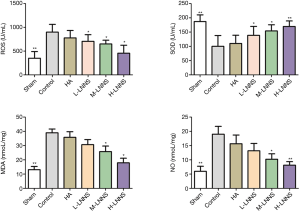
Effects of LNNS on serum TNF-α and IL-1β levels of rats
Compared with the sham operation group, the levels of TNF-α and IL-1β in the control group were dramatically increased (P<0.01). The expression of TNF-α and IL-1β declined after intervention with LNNS (P<0.01). Compared with the control group, its difference was significant, as shown in Figure 5, suggesting that LNNS reduced abdominal adhesion by lowering the level of inflammatory mediators.
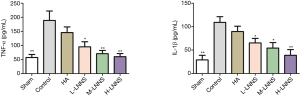
LNNS reduces the expression of MCP-1 and MMP-9 in adhesion tissues
The semiquantitative results of MCP-1 immunohistochemistry are shown in the Figure 6. Results showed that MCP-1 expression in the control group (4.48% vs. 1.58%) had significantly increased compared with the sham operation group, and the level of MCP-1 protein in the adhesion tissues of the high, middle and low doses of LNNS groups was significantly lower than that of the control group after 7 days (P<0.05). There was no significant difference between the high-dose group of LNNS group and the sham-operated group. The concentration of protein in LNNS groups decreased as the dose increased, while the protein expression level decreased in a dose-dependent manner. The semi-quantitative results of MMP-9 immunohistochemistry are shown in Figure 7. Compared with the control group, the MMP-9 protein level in the adhesion tissues of the high, medium and low doses of LNNS groups was significantly increased (P<0.05). The level of MMP-9 in the high dose group of LNNS group was superior to that of the sham operation group.
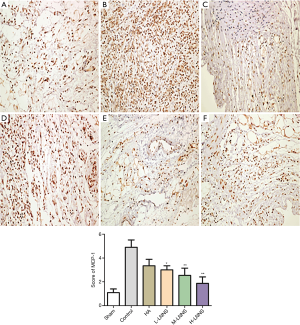
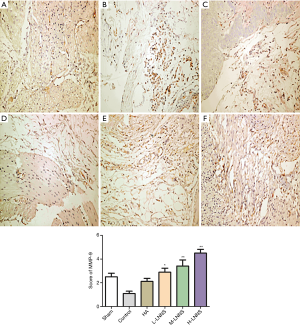
Comparison of mRNA expression levels of Nrf2, heme-oxygenase-1 and NQO1 protein between groups
Compared with the sham operation group, mRNA expression levels of Nrf2, heme-oxygenase-1 and NQO1 in the adhesion in control group increased (P<0.05); the LNNS groups found them grew further, compared with the control group (P<0.01, P<0.05), as shown in Figure 8. It was suggested that LNNS significantly activated the expression of Nrf2, heme-oxygenase-1 and NQO1 mRNA in rats with abdominal adhesion.
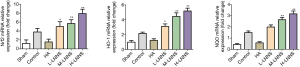
Comparison of the expression levels of Nrf2, heme-oxygenase-1 and NQO1 proteins between groups
Compared with the sham operation group, the expression of Nrf2, heme-oxygenase-1 and NQO1 proteins in the control group significantly went up (P<0.05, P<0.01), and they further increased in LNNS groups, compared with the control group (P<0.01) (Figure 9), suggesting that oxidative stress caused by surgical injury promoted the up-regulation of Nrf2, heme-oxygenase-1 and NQO1 proteins expression, thereby exerting antioxidant effects.
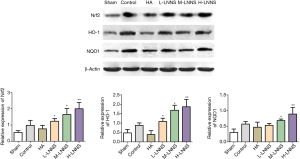
Discussion
Postoperative abdominal adhesion is a major problem after clinical laparotomy that needs to be resolved. The formation of complex and orderly abdominal adhesion involves different pathological processes such as post-traumatic immune activation, inflammatory response and fibrinolysis imbalance (37). Previous studies have shown that ligustrazine, a major active ingredient derived from Rhizoma chuanxiong, has a positive therapeutic effect on various postoperative tissue adhesions. The mechanism may be involved in anti-inflammatory response, inhibiting fibroblast nuclear division and proliferation, reducing fibroblast DNA as well as the synthesis of collagen (38). Our team initially confirmed the LNNS decreased TNF-α dose-dependently, and restored the balance between tPA and PAI-1. In addition, it was confirmed that ligustrazine had no obvious cytotoxicity to L929 cells (29).
In the present study, the rat model of abdominal adhesion was established by using needle files. The results showed that the gross abdominal adhesion index and microscopic inflammation and fibrosis scores of the control group increased. Compared with the control group, the middle and high dose groups of LNNS showed a decrease in the number of adhesions and looseness without angiogenesis, and a significant difference in the scores of abdominal adhesions and pathological sections of the inflammation and fibrosis scores (P<0.01) were found. Masson staining showed that in the medium and high dose groups of LNNS, the thickness of collagen in adhesion tissues was significantly reduced and the formation of abdominal adhesions was inhibited, which is consistent with the previous studies (29). Oxidative-antioxidant system in the body maintains a dynamic balance under physiological condition. When the body suffers from trauma (open surgery), a large number of ROS will be accumulated. Antioxidant factors are unable to eliminate excessive ROS so that oxidative stress occurs, which further damages organs and tissues (39). ROS is a chemically reactive factor containing oxygen, significant for cell signaling and homeostasis maintenance (40). Abnormally elevated ROS causes vascular cell damage, inflammatory cell recruitment, lipid peroxidation, etc. (41). ROS also mediates changes in vascular fibrosis and regulates collagen and fibronectin (30). It has been reported that ROS can induce fibroblast proliferation and extracellular matrix secretion to promote tissue fibrosis (42).
NO, as a signaling molecule, plays a critical role in neurotransmission, blood pressure regulation, inhibition of platelet aggregation and leukocyte adhesion, inhibition of vascular smooth muscle cell proliferation, and immune regulation (43,44). Both NO and ROS are important regulating factors in wound healing. Phospholipids on cell membranes can be oxidized as oxidative stress damage, thus a stable lipid peroxide MDA was formed. MDA content reflects the degree of lipid peroxidation in the body as well as ROS attacking on cells (45). SOD is an important defense against free radical damage in organisms. Widely distributed in various tissues of the body, SOD keeps the balance of free radicals in the body and scavengs superanionic free radicals to protect cells, so its vitality reflects the body’s ability to eliminate oxygen free radicals (46). A recent study showed that by taking Paeoniflorin for 7 days, ROS and SOD activity in the enterocoelia fluid of rats with postoperative abdominal adhesions was reduced and the expression of SOD in peritoneal adhesion specimens increased (47). Askari et al. (48) explained that the activity of antioxidant enzymes such as GSH and the levels of NO and MDA significantly decreased after administration of high, medium and low doses of propolis for 14 days, especially in the high dose group.
Results of this study showed that levels of ROS, MDA and NO in the peritoneal fluid of the control group were higher than those in the sham operation group, while the level of SOD decreased, suggesting that oxidative stress is closely related to the formation of postoperative abdominal adhesion. LNNS reduced the levels of ROS, MDA and NO in peritoneal fluid of rats with experimental abdominal adhesion, and increased the activity of SOD, suggesting that anti-oxidative stress may be caused by LNNS in the prevention and treatment of postoperative abdominal adhesions.
In the inflammatory phase of healing, once the inflammatory cells (white blood cells and macrophages) are activated, they will produce a large amount of peroxide for sterilization, regulation of inflammation and transfer of signals. Excessive peroxide may induce oxidative stress response, leading to delayed or excessive wound repair to form adhesions (49). The inflammatory response is the result of the interaction of various inflammatory cytokines, of which TNF-α and IL-1β are two important pro-inflammatory cytokines. TNF-α is a key mediator in the cytokine chain reaction of abdominal adhesion injury (10). It stimulates vascular endothelial cells and neutrophils to express surface adhesion molecules, promote aggregation, and aggravate the degree of injury (50). In addition, TNF-α stimulates the synthesis of inducible NO synthase to release a large amount of NO (51), which in turn reacts rapidly with superoxide anion to further produce more oxidative hydroxyl radicals. This will cause lipid peroxidation in cell membrane to worsen the damage. Besides, the expression of TNF-α and IL-1β in the serum of the control group was up-regulated 7 days after surgery, which was related to the inflammatory response induced by oxidative stress to trauma after surgery.
MCP-1 is considered to be a highly potent chemotactic factor that plays an important role in inducing fibroblast proliferation and promoting extracellular matrix deposition (52), accelerating the progression of abdominal adhesions (53). Mononuclear/macrophage chemokines are secreted in a large number of major cell groups such as peritoneal fibroblasts and peritoneal mesothelial cells. Once the body has an inflammatory reaction, the secretion of monocyte/macrophage chemokines rapidly increases to bind with receptor CCR2, thus inflammatory cytokines are activated (54). Abdominal adhesions are characterized by adhesion tissue formation and excessive deposition of extracellular matrix. The remodeling and alteration of extracellular matrix is the basic condition for the occurrence and development of abdominal adhesion. The synthesis and degradation of extracellular matrix are affected by many factors, among which hydrolysis of matrix metalloproteinase and the synergistic effect of inhibitor are the key ones, therefore MMP’s role in adhesion tissue attracts increasing attention. MMP-9 (gelatinase B) is one of the major members of the matrix metalloproteinase family, and its main function is to degrade the extracellular matrix and the basement membrane component of type IV collagen (55).
The present study found, compared with the control group, the low, medium and high doses of LNNS significantly reduced MCP-1 protein and increased MMP-9 protein expression in adhesion tissues. It is consisted with the findings of Wei et al. that immunohistochemistry showed a significant increase in the protein content of MMP-9 in adhesion tissues and a drop of the protein content of α-SMA after 7 days of emodin administration (56).
Studies have shown that oxidative stress regulation is considered to be an important intervention target for abdominal adhesions, and the Nrf2-ARE signaling pathway is one of the crucial stress pathways to regulate endogenous defense systems (57,58). The nuclear factor-E2-related factor (2NF-E2)-antioxidant response element (ARE) pathway is the most significant endogenous antioxidant stress pathway discovered so far. Its role in regulating the body's antioxidant capacity has been initially confirmed in various diseases such as digestive system, circulatory system and immune system (59,60). Nrf2 is an important transcription factor that promotes the reduction of cellular ROS and protects against harmful external stimuli (61). Under physiological conditions, Kelch-like ECH-associated protein 1 (Keap1) in the cytoplasm is linked to Nrf2 to inactivate it. Once Nrf2 is activated, it will separate from Keap1 and transfer into the nucleus to recognize and bind a series of ARE that can initiate the expression of the corresponding downstream phase II detoxification enzyme gene, and the expression of such genes can induce the expression of some important antioxidant substances (such as SOD, CAT, etc.) in the cell, which in turn increases clearance and metabolism of exogenous toxic substances and free radicals (59). As an important downstream target gene driven by Nrf2, HO-1, NAD(P) and Nqo1 are representative components of antioxidant stress injury (62). HO-1 and NQO1 are the two major antioxidant enzymes of downstream in this pathway. HO-1 is a rate-limiting enzyme that catalyzes the production of bilirubin, Fe2+ and carbon monoxide, while biliverdin can be further formed into bilirubin by reductase. Bilirubin has a broad spectrum of antioxidant against a variety of harmful stimuli such as hypoxia, inflammation and other damage (63). The Nrf2-ARE pathway is the major endogenous antioxidant stress pathway and an important factor for the imbalance of inflammation-coagulation-fibrosis and the formation of adhesions (30). Therefore, study of the oxidative stress pathway surrounding Nrf2 and the regulation of downstream factors is conducive to the prevention and treatment of abdominal adhesions.
The results of this study showed that the expression of Nrf2, heme-oxygenase-1, NQO1 protein and mRNA in postoperative abdominal adhesion rats were higher than those in the sham-operated group, suggesting that the surgical damage can destroy the homeostasis of the organism and it may induce compensatory protection. It then activates the Nrf2-ARE signaling pathway under stress, and promotes the up-regulation of Nrf2 and heme-oxygenase-1 to protect against damage caused by harmful stimuli. Compared with the control group, the expression levels of Nrf2, heme-oxygenase-1, NQO1 protein and mRNA further increased, suggesting that Nrf2, heme oxygenase 1 and NQO1 were further increased in the postoperative abdominal adhesion rats intervened with LNNS in this experiment. They exerted antioxidant and cytoprotective effects.
In summary, activation of Nrf2-ARE signaling pathway promotes dissociation of Nrf2 from Keap1 to bind into nucleus and combine with ARE. It then up-regulates the expression of HO-1 and NQO1, and induces the expression of antioxidant protein to reduce lipid peroxidation damage in vivo. Therefore, attenuating the inflammatory response may be an effective target and pathway for the anti-adhesion effect of LNNS. The regulation of Nrf2-ARE signaling pathway may provide new attempts and considerations for the prevention and treatment of postoperative abdominal adhesions.
However, shortcomings are inevitable. The main adverse drug reactions (ADR) of ligustrazine injection in clinic is rash (64). Besides, gastrointestinal ADR (30% of total ADR) including nausea, vomiting, abdominal distension, stomach discomfort should not be ignored (65-67). Its ADR might be the results from plasma protein amino compounds that synthesizes larger molecules (67). The primary problem is a lack of evaluation of the side effects of LNNS. In spite of its advantages of low cytotoxicity and biocompatibility, whether LNNS will cause stress stimulation after spraying directly on the wound has not been studied. So, while paying attention to the application of LNNS, we should pay more attention to the biosafety of the dosage, form and application method. Its clinical transformation requires further experimental and clinical research.
Conclusions
This present results indicated that LNNS can attenuate the extent and severity of peritoneal adhesion, either through reduction of inflammatory factors or modulation of antioxidants status. Therefore, LNNS can be considered as a great potential agent against post-surgical peritoneal adhesion.
Acknowledgments
Funding: This study was financially supported by the National Natural Science Foundation of China (No. 81673982, 81804098), China Postdoctoral Science Foundation (No. 2019T120453), Jiangsu Natural Science Foundation (BK20180219), Jiangsu Youth Medical Talents Project (QNRC2016255) and Suzhou Hospital of Traditional Chinese Medicine Youth issues (YQN2016003).
Footnote
Conflicts of Interest: The authors have no conflicts of interest to declare.
Ethical Statement: The authors are accountable for all aspects of the work in ensuring that questions related to the accuracy or integrity of any part of the work are appropriately investigated and resolved. All animal procedures performed in this study were reviewed, approved, and supervised by the Institutional Animal Care and Use Committee of the Ethics Committee of Nanjing University of Chinese Medicine, China (No. 20170366).
References
- Moris D, Chakedis J, Rahnemai-Azar AA, et al. Postoperative Abdominal Adhesions: Clinical Significance and Advances in Prevention and Management. J Gastrointest Surg 2017;21:1713-22. [Crossref] [PubMed]
- Arung W, Meurisse M, Detry O. Pathophysiology and prevention of postoperative peritoneal adhesions. World J Gastroenterol 2011;17:4545-53. [Crossref] [PubMed]
- Brüggmann D, Tchartchian G, Wallwiener M, et al. Intra-abdominal adhesions: definition, origin, significance in surgical practice, and treatment options. Dtsch Arztebl Int 2010;107:769-75. [PubMed]
- Sisodia V, Sahu SK, Kumar S. Clinical Profile of Patients with Postoperative Adhesive Intestinal Obstruction and its Association with Intraoperative Peritoneal Adhesion Index. Chirurgia (Bucur) 2016;111:251-8. [PubMed]
- Koninckx PR, Gomel V, Ussia A, et al. Role of the peritoneal cavity in the prevention of postoperative adhesions, pain, and fatigue. Fertil Steril 2016;106:998-1010. [Crossref] [PubMed]
- Hamming JF, Bonsing BA. Adhesiolysis during abdominal surgery: substantial risks. Ned Tijdschr Geneeskd 2013;157:A5928. [PubMed]
- Ten Broek RPG, Stommel MWJ, Strik C, et al. Benefits and harms of adhesion barriers for abdominal surgery: a systematic review and meta-analysis. Lancet 2014;383:48-59. [Crossref] [PubMed]
- Diamond MP. Reduction of postoperative adhesion development. Fertil Steril 2016;106:994-7.e1. [Crossref] [PubMed]
- Catena F, Ansaloni L, Di Saverio S, et al. P.O.P.A. study: prevention of postoperative abdominal adhesions by icodextrin 4% solution after laparotomy for adhesive small bowel obstruction. A prospective randomized controlled trial. J Gastrointest Surg 2012;16:382-8. [Crossref] [PubMed]
- Bayhan Z, Zeren S, Kocak FE, et al. Antiadhesive and anti-inflammatory effects of pirfenidone in postoperative intra-abdominal adhesion in an experimental rat model. J Surg Res 2016;201:348-55. [Crossref] [PubMed]
- Jiang S, Wang W, Yan H, et al. Prevention of intra-abdominal adhesion by bi-layer electrospun membrane. Int J Mol Sci 2013;14:11861-70. [Crossref] [PubMed]
- Steinleitner A, Lambert H, Kazensky C, et al. Reduction of primary postoperative adhesion formation under calcium channel blockade in the rabbit. J Surg Res 1990;48:42-5. [Crossref] [PubMed]
- Chen CH, Kuo CY, Chen SH, et al. Thermosensitive Injectable Hydrogel for Simultaneous Intraperitoneal Delivery of Doxorubicin and Prevention of Peritoneal Adhesion. Int J Mol Sci 2018. [Crossref] [PubMed]
- Parsaei P, Karimi M, Asadi SY, et al. Bioactive components and preventive effect of green tea (Camellia sinensis) extract on post-laparotomy intra-abdominal adhesion in rats. Int J Surg 2013;11:811-5. [Crossref] [PubMed]
- Ahmad G, O'Flynn H, Hindocha A, et al. Barrier agents for adhesion prevention after gynaecological surgery. Cochrane Database Syst Rev 2015.CD000475. [PubMed]
- Klink CD, Schickhaus P, Binnebösel M, et al. Influence of 4% icodextrin solution on peritoneal tissue response and adhesion formation. BMC Surg 2013;13:34. [Crossref] [PubMed]
- Park KS, Lee KE. Antiadhesive effect and safety of oxidized regenerated cellulose after thyroidectomy: a prospective, randomized controlled study. J Korean Surg Soc 2013;84:321-9. [Crossref] [PubMed]
- Oner G, Ulug P. A systemic review of randomized controlled studies about prevention with pharmacologic agents of adhesion formation in the rat uterine horn model. Arch Med Sci 2015;11:274-81. [Crossref] [PubMed]
- Liu XM, Wang YB, Wu Q, et al. Effects of Ligustrazine on Airway Inflammation in A Mouse Model of Neutrophilic Asthma. Chin J Integr Med 2018;24:353-8. [Crossref] [PubMed]
- Zhao Y, Liu Y, Chen K. Mechanisms and Clinical Application of Tetramethylpyrazine (an Interesting Natural Compound Isolated from Ligusticum Wallichii): Current Status and Perspective. Oxid Med Cell Longev 2016;2016:2124638. [Crossref] [PubMed]
- Zou J, Gao P, Hao X, et al. Recent progress in the structural modification and pharmacological activities of ligustrazine derivatives. Eur J Med Chem 2018;147:150-62. [Crossref] [PubMed]
- Zhang CG, Yang GQ. Intraperitoneal Perfusion of Ligustrazin in the treatment of intestinal Adhesion: a report of 6 cases. J Pract Med 2000;16:319.
- Zhang JY, Liao KS, Wu QF, et al. Experimental and clinical study of Ligustrazine in preventing postoperative intestinal adhesion. J Hubei Institute Natlit 2006;23:1-3.
- Wang XW, Lu TL, Lu JF, et al. Experimental research on the preventive effect of Ligustrazine nanoparticles on postoperative peritoneal adhesion rats. J Nangjing Univ Tradit Chin Med 2011;27:148-50.
- Yan YL, Zhou LL. Research survey on Ligustrazine. Lishizhen Med Mater Med Res 2008;19:1343-5.
- Zeng L, Mao CQ, Lu TL, et al. Preparation technology and quality assay of ligustrazine poly lactic acid nanoparticles. Chin Tradit Patent Med 2013;35:261-3.
- Li ZY, Mao CQ, Zeng L, et al. Determination of entrapment efficiency of tetramethylpyrazine polylactic aci nanoparticles. J Nangjing Univ Tradit Chin Med 2011;27:77-9.
- Yan S, Yang L, Yue YZ, et al. Effect of ligustrazine nanoparticles nano spray on transforming growth factor-β/Smad signal pathway of rat peritoneal mesothelial cells induced by tumor necrosis factor-α. Chin J Integr Med 2016;22:629-34. [Crossref] [PubMed]
- Yan S, Yue YZ, Zeng L, et al. Effect of intra-abdominal administration of ligustrazine nanoparticles nano spray on postoperative peritoneal adhesion in rat model. J Obstet Gynaecol Res 2015;41:1942-50. [Crossref] [PubMed]
- Awonuga AO, Belotte J, Abuanzeh S, et al. Advances in the Pathogenesis of Adhesion Development: The Role of Oxidative Stress. Reprod Sci 2014;21:823-36. [Crossref] [PubMed]
- Lugrin J, Rosenblatt-Velin N, Parapanov R, et al. The role of oxidative stress during inflammatory processes. Biol Chem 2014;395:203-30. [Crossref] [PubMed]
- Yan Z, Wenlin L, Li Z, et al. Optimal dose of Ligustrazine nanoparticles in a rat model of experimental peritoneal adhesion. Chinese Journal of Tissue Engineering Research 2014;18:5799-804.
- Zhang H, Song Y, Li Z, et al. Evaluation of ligustrazine on the prevention of experimentally induced abdominal adhesions in rats. Int J Surg 2015;21:115-21. [Crossref] [PubMed]
- Yue Y, Yan S, Li H, et al. The role of oral fluvastatin on postoperative peritoneal adhesion formation in an experimental rat model. Acta Chir Belg 2018;118:372-9. [Crossref] [PubMed]
- Phillips RK, Dudley HA. The effect of tetracycline lavage and trauma on visceral and parietal peritoneal ultrastructure and adhesion formation. Br J Surg 1984;71:537-9. [Crossref] [PubMed]
- Bhatia DS, Allen JE. The prevention of experimentally induced postoperative adhesions. Am Surg 1997;63:775-7. [PubMed]
- Hong G, Vilz TO, Kalff JC, et al. Peritoneal adhesion formation. Chirurg 2015;86:175-80. [Crossref] [PubMed]
- Yan S, Li WL, Zeng L. Review on Anti-adhesion Activity of Tetramethylpyrazine. Nat Prod Res Dev 2015;27:367-71.
- Mahdy T, Mohamed G, Elhawary A. Effect of methylene blue on intraabdominal adhesion formation in rats. Int J Surg 2008;6:452-5. [Crossref] [PubMed]
- Brierley DJ, Martin SA. Oxidative stress and the DNA mismatch repair pathway. Antioxid Redox Signal 2013;18:2420-8. [Crossref] [PubMed]
- Shin HS, Ko J, Kim DA, et al. Metformin ameliorates the Phenotype Transition of Peritoneal Mesothelial Cells and Peritoneal Fibrosis via a modulation of Oxidative Stress. Sci Rep 2017;7:5690. [Crossref] [PubMed]
- Tao YE, Wen Z, Song Y, et al. Paeoniflorin attenuates hepatic ischemia/reperfusion injury via anti-oxidative, anti-inflammatory and anti-apoptotic pathways. Exp Ther Med 2016;11:263-8. [Crossref] [PubMed]
- Ahmad A, Dempsey SK, Daneva Z, et al. Role of Nitric Oxide in the Cardiovascular and Renal Systems. Int J Mol Sci 2018;19. [Crossref] [PubMed]
- Vanhoutte PM. Nitric Oxide: From Good to Bad. Ann Vasc Dis 2018;11:41-51. [Crossref] [PubMed]
- Mao S, Zhang A, Huang S. Serum levels of malondialdehyde, vitamin C and E in idiopathic nephrotic syndrome: a meta-analysis. Ren Fail 2014;36:994-9. [Crossref] [PubMed]
- Bresciani G, da Cruz IB, González-Gallego J. Manganese superoxide dismutase and oxidative stress modulation. Adv Clin Chem 2015;68:87-130. [Crossref] [PubMed]
- Gao Q, Wei G, Wu Y, et al. Paeoniflorin prevents postoperative peritoneal adhesion formation in an experimental rat model. Oncotarget 2017;8:93899-911. [Crossref] [PubMed]
- Askari VR, Rahimi VB, Zamani P, et al. Evaluation of the effects of Iranian propolis on the severity of post operational-induced peritoneal adhesion in rats. Biomed Pharmacother 2018;99:346-53. [Crossref] [PubMed]
- Sies H. Oxidative stress: a concept in redox biology and medicine. Redox Biol 2015;4:180-3. [Crossref] [PubMed]
- Beltran SR, Svoboda KK, Kerns DG, et al. Anti-inflammatory protein tumor necrosis factor-α-stimulated protein 6 (TSG-6) promotes early gingival wound healing: an in vivo study. J Periodontol 2015;86:62-71. [Crossref] [PubMed]
- Feng HM, Walker DH. Interferon-gamma and tumor necrosis factor-alpha exert their antirickettsial effect via induction of synthesis of nitric oxide. Am J Pathol 1993;143:1016-23. [PubMed]
- Gao Y, Luo L, He F. Effect of monocyte chemotactic protein-1 on the intraperitoneal adhesion formation. J Tongji Med Univ 2000;20:340-2. [Crossref] [PubMed]
- Zeyneloglu HB, Senturk LM, Seli E, et al. The role of monocyte chemotactic protein-1 in intraperitoneal adhesion formation. Hum Reprod 1998;13:1194-9. [Crossref] [PubMed]
- Lee SH, Kang HY, Kim KS, et al. The monocyte chemoattractant protein-1 (MCP-1)/CCR2 system is involved in peritoneal dialysis-related epithelial-mesenchymal transition of peritoneal mesothelial cells. Lab Invest 2012;92:1698-711. [Crossref] [PubMed]
- Heo SH, Cho CH, Kim HO, et al. Plaque rupture is a determinant of vascular events in carotid artery atherosclerotic disease: involvement of matrix metalloproteinases 2 and 9. J Clin Neurol 2011;7:69-76. [Crossref] [PubMed]
- Wei G, Wu Y, Gao Q, Zhou C, Wang K, Shen C, Wang G, Wang K, Sun X. Li Xet al. Effect of Emodin on Preventing Postoperative Intra-Abdominal Adhesion Formation. Oxid Med Cell Longev 2017;2017:1740317. [Crossref] [PubMed]
- Fredriksson F, Christofferson RH, Carlsson PO, et al. Locally increased concentrations of inflammatory cytokines in an experimental intraabdominal adhesion model. J Pediatr Surg 2014;49:1480-4. [Crossref] [PubMed]
- Kuru S, Bozkirli OB, Barlas AM, et al. The Preventive Effect of Dexmedetomidine Against Postoperative Intra-abdominal Adhesions in Rats. Int Surg 2015;100:87-95. [Crossref] [PubMed]
- Kweider N, Wruck CJ, Rath W. New Insights into the Pathogenesis of Preeclampsia - The Role of Nrf2 Activators and their Potential Therapeutic Impact. Geburtshilfe Frauenheilkd 2013;73:1236-40. [Crossref] [PubMed]
- Velagapudi R, Kumar A, Bhatia HS, et al. Inhibition of neuroinflammation by thymoquinone requires activation of Nrf2/ARE signalling. Int Immunopharmacol 2017;48:17-29. [Crossref] [PubMed]
- Jung KA, Kwak MK. The Nrf2 system as a potential target for the development of indirect antioxidants. Molecules 2010;15:7266-91. [Crossref] [PubMed]
- Li L, Dong H, Song E, et al. Nrf2/ARE pathway activation, HO-1 and NQO1 induction by polychlorinated biphenyl quinone is associated with reactive oxygen species and PI3K/AKT signaling. Chem Biol Interact 2014;209:56-67. [Crossref] [PubMed]
- Kim HJ, Zheng M, Kim SK, et al. CO/HO-1 Induces NQO-1 Expression via Nrf2 Activation. Immune Netw 2011;11:376-82. [Crossref] [PubMed]
- Yang XM. Research progress on the pharmacological effects of Ligustrazine. Chin J Biochem Pharm 2010;31:215-7.
- Bei L. Analysis of 50 Cases of Adverse Drug Reactions Due to Salvia Miltiorrhiza and Ligustrazine Hydrochloride Injection. Chin J Drug Evaluation 2013;30:159-64.
- Wang TZ, Huang P. Adverse drug reaction caused by Ligustrazine Hydrochloride for Injection: An analysis of 506 cases. Anhui Med and Pharm J 2015;19:610-2.
- Wen Z, Jiang L, Ting L. Review of irrational combined use of TCMIA in a hospital. Chin Hosp Pharm J 2017;37:2193-6.