MicroRNA-188 aggravates contrast-induced apoptosis by targeting SRSF7 in novel isotonic contrast-induced acute kidney injury rat models and renal tubular epithelial cells
Introduction
Contrast-induced acute kidney injury (CI-AKI) is a clinical syndrome that often follows cardiac catheterization. As the frequency of interventional cardiac procedures have steadily increased, CI-AKI has become the third cause for hospital-acquired AKI (1), affecting 1% to 2% of the general population and up to 50% of high-risk patients following emergency coronary angiography or percutaneous coronary intervention (PCI) (2). CI-AKI is also associated with significantly higher mortality and worsened clinical outcome, resulting in a 3-fold and 7-fold increase in short-term and long-term mortality respectively (3,4). Modern frameworks theorize that contrast media (CM) activate renal tubular epithelial cell apoptosis mainly through direct CM toxicity, vasoconstriction, and oxidative stress, which leads to renal function loss and acute kidney injury (5). Though the macroscopic the pathophysiological mechanism of CI-AKI has been demonstrated, the underlying molecular mechanisms and regulatory networks are still unclear. Due to the absence of molecular mechanisms, the effectiveness of clinical prevention strategies for CI-AKI, like hydration, are controversial and limited, while specific targeted drugs are lacking (6,7). Therefore, the investigation of potential pathways and the identifying of key regulatory molecules underlying CI-AKI remain an area of critical research interest.
MicroRNAs (miRNAs) are endogenous, non-coding, and 21–25 nt RNAs, which post-transcriptionally induce mRNA decay by binding e 3’-untranslated region (UTR) competitively (8,9). Numerous studies have shown that miRNAs actively participate in the pathological process of acute kidney injury (AKI), and understanding this may have great potential for the diagnosis and treatment of AKI (10,11). However, research investigating the miRNAs involved in CI-AKI is rare, and what roles they may play in regulation and their potential as novel drug targets in CI-AKI are still unclear.
In order to explore the regulatory effect miRNAs exert and to identify the key miRNAs for drug design, we established a novel isotonic CI-AKI rat model by using isotonic CM iodixanol which is commonly used in clinic. After sequencing microRNAs from renal tissues, we found microRNA-188 (miRNA-188) was significantly up-regulated in isotonic CI-AKI rat models. Other research has shown that miRNA-188 can promote cell proliferation and autophagy in cancer, along with myocardial infarction (12,13). Despite this, the function that miRNA-188 performs in AKI remains unreported. To determine the regulatory function of miRNA-188 and to explore potential therapeutic targets, we examined miR-188 expression levels and performed gain- and loss-of-function experiments in the present study. The results suggest that miRNA-188 aggravates CI-AKI through apoptosis by directly targeting SRSF7 and could thus be a potential target for intervention.
Methods
Novel isotonic CI-AKI rat model
Classic CI-AKI animal model is induced by injecting high-osmotic, high renal toxic CM such as iohexol and ioversol, which are rarely used in cardiac catheterization. In order to establish a representative, close-to-clinic isotonic CI-AKI model, we built the novel isotonic CI-AKI rat model by injecting furosemide along with an isotonic, lower toxicity CM, iodixanol, which is now widely used in interventional cardiac procedures. Male Sprague-Dawley rats (200–220 g) were purchased from the Guangdong Animal Center (Guangzhou, China). All experimental procedures were performed in accordance with the Guide for the Care and Use of Laboratory Animals (National Research Council Publication, 8th Edition, 2011) and were in compliance with the Chinese Regulations for the Administration of Affairs Concerning Experimental Animals. The animal experimental protocol was approved by the Guangdong General Hospital Ethics Research Committee (No. 2015253). The rats were acclimated for 7 d prior to the study and then divided into 2 groups (the CI-AKI group and control group). Based on a previous method used for the CI-AKI rat model (14), we established a novel method to induce isotonic CI-AKI rat model. Before the experiments, the rats were anesthetized with 15 mL/kg 10% chloral hydrate by intraperitoneal injection. To maintain a dehydration state, furosemide was administrated intraperitoneally with 15 mL/kg for 6 h before and after CM exposure. Following a 6-hour dehydration period, the rats in the CI-AKI group were injected with the iodixanol CM (viviparus, 320 mgI/mL, 290 mosm/kg H2O, 11.8 mPs at 37 °C; GE Healthcare, Shanghai, China) at a dose of 15 mL/kg via the tail vein over the course of 5 min. The control group received an equal volume of normal saline.
Assessment of rat renal functions
Blood samples were collected from the orbital vein at baseline and 24 h after CM administration. Serum creatinine (Scr) and cystatin C (Cys C) concentrations were examined as markers of renal dysfunction using the AU 5800 chemistry analyzer (Beckman Coulter, Inc., CA, USA). CI-AKI was defined as an increase in serum Scr or Cys C concentration of 25% above baseline within 48 to 72 h after contrast administration (15).
The kidneys were excised after contrast exposure for 24 h. The renal tissues were embedded in paraffin and were cut into 5 µm slices stained with hematoxylin and eosin (HE) to evaluate kidney morphology. The kidney morphology characteristics were evaluated according to Billings’ paper (16), including the interstitial edema, cytoplasmic vacuolar changes, and intratubular cast formation. Histopathological analysis was performed by an experienced pathologist blinded to the groups.
Cell culture
HK-2 cells were provided by the Stem Cell Bank (Chinese Academy of Sciences, Shanghai, China). HK-2 cells were cultured in low glucose DMEM/F12 medium (Gibco™; Thermo Fisher Scientific, Waltham, MA, USA), containing 10% fetal bovine serum (FBS), 0.1% penicillin, and streptomycin at 37 °C with 5% CO2. Cells were subcultured when cells reached 80% confluence. In contrast-media-treated groups, cells were treated with 150 mgI/mL contrast media iodixanol (Visipaque, 320 mgI/mL, 290 mosm/kg of water and 11.8 mPs at 37 °C; GE Healthcare, Shanghai, China) for 2 h, and were then cultivated in DEME/F12 medium with 10% FBS for 24 h.
MTS assay of cell viability
Cell viability was assessed with CellTiter96™ AQueous One Solution Cell Proliferation Assay (Promega, Madison, WI, USA) according to the manufacturer’s protocol. HK-2 cells were prepared in 96-well plates containing a final volume of 100 µl/well. After transfection of miR-188 mimics and inhibitors for 48 h, cells were added with MTS Reagent 20 µL/well and incubated for 2 h at 37 °C. Percentages of cell viability were assessed by spectrophotometry at 490 nm.
TUNEL assay
Terminal deoxynucleotidyl transferase dUTP nick-end labeling (TUNEL) assay for cell apoptosis in HK-2 cells was used according to the manufacturer’s protocol (DeadEnd™; Promega, Madison, WI, USA). Cells were seeded on Lab-Tek® Chamber Slides, and then were fixed with 4% paraformaldehyde for 25 min at 4 °C. After two washes with PBS, cells were immersed in 0.2% Triton® X-100 solution in PBS for 5 min, and incubated with rTdT incubation buffer at 37 °C for 60 min. Following 3 washes with PBS to remove unincorporated fluorescein-12-dUTP, cell nuclei were stained with DAPI, and were subsequently analyzed under a fluorescence microscope. Positive cells were counted under 5 randomly collected visual fields at 400× magnification.
Reverse transcription-quantitative polymerase chain reaction (RT-qPCR) analysis
Total RNA from cultured cells was extracted using TRIzol reagent (Thermo Fisher Scientific, Waltham, MA, USA) according to the manufacturer’s protocol. The concentration and purity of the RNA samples were assayed by Nanodrop. To determine the expression of miRNA, the isolated RNA was reverse transcribed using the TaqMan™ MicroRNA Reverse Transcription Kit (Applied Biosystems, Thermo Fisher Scientific, Waltham, MA, USA). The quantitative PCR was performed using TaqMan Advanced miRNA Assay (Applied Biosystems, Thermo Fisher Scientific, Waltham, MA, USA). The primers for miRNA in the reactions were synthesized by Applied Biosystems. MiRNA expression level was normalized against snU6.
For mRNA expression, isolated RNA was reverse transcribed to cDNA, quantitative PCR was performed using SYBR green real-time PCR kit (Thermo Fisher Scientific, Waltham, MA, USA) according to the manufacturer’s protocol. The expression level was normalized against β-actin. All reactions were repeated in triplicate, and the differential expression levels were determined by the comparative CT (ΔΔCt) method. The primers were as follows: miRNA-188: forward 5'-CAUCCCUUGCAUGGUGGAGGG-3' and reverse 5'-CCCTCCACCATGCAAGGGATG-3'; SRSF7: forward 5'- GACGAAGGTCAAGGTCAGCA-3' and reverse 5'- CGACTGCTTCTTGGTCGTGA-3'
Western blotting
Cells were lysed, and protein was extracted with RIPA lysis buffer (Thermo Fisher Scientific, Waltham, MA, USA). Protein concentration was evaluated with the BCA method. Equal amounts of protein (40 µg) were size fractionated in 10% SDS-PAGE and electrophoretically transferred to PVDF membrane. The membranes were blocked in 5% BSA-TBST for 2 h at room temperature, and were then incubated with primary antibodies overnight at 4 °C. After washing with TBST, the membranes were incubated with horseradish peroxidase-conjugated secondary antibody for 1 h at room temperature. Finally, antibody labeling was detected by enhanced chemiluminescence (Thermo Fisher Scientific, Waltham, MA, USA). Taking GAPDH as the internal reference, band intensity was evaluated by Image J v1.48 U software (National Institutes of Health, Bethesda, MD, USA).
Transfection of oligonucleotides and small interfering RNA
Cells were seeded in 6-well plates and grown to 70–90% confluence at the time of transfection. The miR-188 mimic, miR188 inhibitor, and negative controls (NC) were synthesized by GenePharma Co., Ltd (Shanghai, China). Transfection was performed using Lipofectamine 2000 (Invitrogen, Carlsbad, CA, USA) according to the manufacture’s protocol. After transfection for 48 h, cells were exposed to contrast media in a manner described previously. The efficiency of miR-188 mimic or inhibitor transfection was confirmed by RT-qPCR. The sequences were as follows: miRNA-188 mimics, 5'- CUCCACCAUGCAAGGGAUGUU-3'; miRNA-188 inhibitors, 5'- CCCUCCACCAUGCAAGGGAUG-3'; siRNA-SRSF7, 5'-ACUGAAAGCCCU UUCUAACUC-3',
Dual luciferase reporter assay
The potential binding sites of miR-188 in the 3' untranslated region (3'UTR) of SRSF7 mRNA were predicted by the TargetScan algorithm. The wild-type (WT) or mutant (MUT) SRSF7 3'UTR were ligated into the pGL3 luciferase reporter vectors (Promega Corporation, Madison, WI, USA). HEK-293T cells were seeded into 12-well plates and grown to about 70% confluence at the time of transfection. Next, cells were transfected with pGL3-SRSF7-3'UTR-WT or pGL3-SRSF7-3'UTR-MUT along with miR-188 mimics or miR-188 negative control. After 48 h in transfection, the relative firefly luciferase activity was calculated by normalization to the Renilla luciferase activity.
Statistical analysis
Data were reported as mean ± standard deviation. At least three independent repetitive experiments were conducted. Comparisons between the two unpaired groups were performed using a Student’s t-test. A value of two-tailed P<0.05 was considered to indicate a statistically significant difference. Statistical analysis was performed with GraphPad Prism v6.0 (GraphPad Software, Inc., La Jolla, CA, USA) SPSS software v22.0 (IBM Corp., Armonk, NY, USA).
Results
Successful establishment of novel isotonic CI-AKI rat models
Iohexol is a nonionic monomer CM with 830 mOsm/kg H2O of osmotic pressure which is much higher than plasma osmotic pressure (350 mOsm/kg H2O). Due to the high osmotic pressure, iohexol is believed to exhibit high renal toxicity, and it is rarely used in cardiac catheterization. The classical rat model of CI-AKI is induced by the seldom-used iohexol, which cannot representatively reflect the actual state of clinical CI-AKI patients. Iodixanol, however, is widely used in interventional cardiac procedures because of its lower renal toxicity and isotonic pressure (290 mOsm/kg H2O) (17). Due to the distinct differences in physicochemical characteristics and toxicity between iohexol and iodixanol, we believe the classical CI-AKI rat model could be improved. Therefore, we established the representative, close-to-clinic, isotonic CI-AKI model by using a CM commonly used in clinic, iodixanol. By using the same contrast media as in CI-AKI patients, we believe our novel isotonic CI-AKI rat models can more representatively simulate the actual pathological state of CI-AKI than the classic model.
As shown in Figure 1, we randomly divided 70 rats into two groups (the CI-AKI group and control group). After we established the isotonic CI-AKI rat models, serum creatinine (Scr) and cystatin C (cys C) were determined in order to identify whether the CI-AKI rat models were established successfully (Figure 1B); 94% (47/50) of the rats from the CI-AKI group developed CI-AKI successfully as indicated by an increase in SCr or cys C ≥25% (15). HE staining and electron microscope observation of the renal tissues were conducted for the detection of histopathological and subcellular changes. HE staining showed that the renal tissues of the control group were basically normal, while the CI-AKI group demonstrated evident renal tubular edema, occlusion, and necrosis. Under electron microscope observation, the CI-AKI group exhibited increased thickening of the glomerular basement membrane with vacuoles, microvilli in tubular detachment, and mitochondrial swelling when compared with the control group.
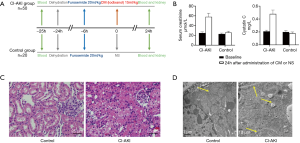
According to Scr and cys C measurements, in combination with histopathological and subcellular changes observed by HE staining and an electron microscope, novel CI-AKI rat models induced by injected furosemide and iodixanol were established successfully.
MiRNA-188 is highly expressed in CI-AKI rat models and cells
Based on the microRNA expressions of renal tissues collected from novel isotonic CI-AKI rat models we sequenced in a previous study, 41 significant differently expressed miRNAs, including miRNA-188, were screened by bioinformatics. MiRNA-188 was significantly up-regulated in all 6 sequenced isotonic CI-AKI rat models when contrasted with the control group, with a mean difference in fold change of 3.57 and P<0.001 (Figure 2A). In order to evaluate miRNA-188 expressions in renal tubular epithelial cells, RT-qPCR was performed in HK-2 cells treated with iodixanol, which revealed that the miRNA-188 levels were 1.87 times higher (P<0.001) in CI-AKI cell models than in normal HK-2 cells (Figure 2B). Additionally, miRNA-188 expression levels in cell and animal models were consistent with the expression in CI-AKI patient plasma found by Sun et al., with a mean difference in fold change of 1.594 and P<0.001 (18). As indicated by the miRNA-188 expressions in cell models, isotonic CI-AKI rat models, and patients’ plasma, miRNA-188 was highly expressed in CI-AKI.
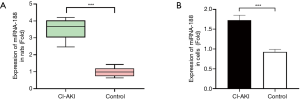
MiRNA-188 overexpression aggravates apoptosis in CI-AKI cell models
To further investigate the role that miRNA-188 plays in CI-AKI, HK-2 cells were successfully transfected with miRNA-188 mimics (miRNA-188 mimics group) or negative control miRNAs (mimics-NC group) (Figure 3A). After treating the miRNA-188 mimics group and the mimics-NC group with CM, experiments including western blot and TUNEL assay were ordered to determine the apoptosis levels in these groups. Western blot analysis showed that the Bcl-2 expression in the miRNA-188 mimics group was 0.61 times lower than in the mimics-NC group with P<0.05 (Figure 3B), which suggests apoptosis is upregulated after transfection of miRNA-188 mimics. TUNEL assay also revealed that the apoptosis level in the miRNA-188 mimics group was 2.34 times higher than in the mimics-NC group (P<0.01) (Figure 3C). Therefore, western blot and TUNEL assay in the CI-AKI cell models both demonstrate that miRNA-188 may participate in CI-AKI through aggravating renal tubular epithelial cell apoptosis.
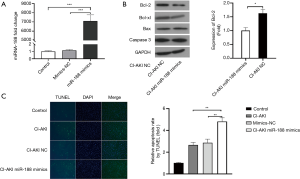
MiRNA-188 induce apoptosis by targeting SRSF7 in CI-AKI cell models
Bioinformatics predicted that SRSF7 may be a potential target of miRNA-188, since the SRSF7 3' UTR possesses a miRNA-188 binding site in 51-57 of SRSF7 3' UTR (Figure 4A). RT-qPCR was performed to explore the association between miRNA-188 and SRSF7 mRNA expressions. SRSF7 expression was significantly downregulated in CI-AKI cell models transfected miRNA-188 mimics than those transfected mimic-NC (P<0.01) (Figure 4B). Therefore, RT-qPCR verified that miRNA-188 overexpression represses SRSF7 expression, which is consistent with our hypothesis that miRNA-188 induced apoptosis by targeting SRSF7. In order to confirm the interaction between miRNA-188 and SRSF7, luciferin reporter gene assay was ordered. HK-2 were transfected with plasmids containing WT or MUT SRSF 3' UTR, and fluorescence intensity was recorded. The luciferase activity in HK-2 cells transfected with pGL3-SRSF7-3'UTR-WT was reduced by 56% (P<0.001) relative to that in cells transfected with the MUT construct (Figure 4C), suggesting that miRNA-188 may regulate SRSF7 expression by directly binding to the transcript. SiRNA was used to knock down SRSF7 expression in HK-2 cells, and the effect of this was validated by RT-qPCR (Figure 4D). This decrease was accompanied by a 51% decline (P<0.01) in cell viability rate according to MTS assay. Based on the above experimental results, miRNA-188 may induce renal tubular epithelial cell apoptosis by targeting SRSF7 in CI-AKI cell models (Figure 4E).
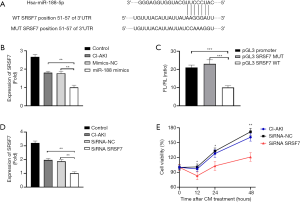
Discussion
Contrast-induced acute kidney injury is the third cause of hospital-acquired AKI that results from administration of contrast media. CI-AKI is correlated with high hospital costs, high mortality, and worsened clinical outcome (1,3). During the process, direct tubular cell toxicity, an enormous amount of oxygen-free radicals, and severe vasoconstriction effect are caused by CM molecules (19). These damaging effects combine to induce apoptosis, redistribution of membrane proteins, reduction of extracellular Ca2+, DNA fragmentation, disruption of intercellular junctions, reduced cell proliferation, and altered mitochondrial function, which ultimately lead to renal function loss and acute kidney failure (20,21). Although the macroscopic pathophysiological mechanism of CI-AKI has been clarified, how CM induces direct tubular cell toxicity, oxygen-free radical release, and vasoconstriction is still unknown; understanding these processes is crucial for clinical early diagnosis and intervention. Recently, microRNAs were reported to be implicated in the nosogenesis of AKI and may be able to serve as prognostic biomarkers and drug targets (10). In this study, we aimed to find out the mechanism for which miRNA-188 affects CI-AKI, and we ultimately found that miRNA-188 could induce apoptosis by binding SRSF7, thereby further aggravating CI-AKI progression.
Initially, we observed that the classical CI-AKI rat model is induced by CM that are rarely used in clinic, the highly osmotic iohexol and ioversol, which have different physicochemical characteristics and toxicity from iodixanol, a more clinically common CM. Given this, we established a representative, close-to-clinic isotonic CI-AKI rat model by using iodixanol. In our previous study, we sequenced microRNAs from renal tissues and discovered that miRNA-188 was significantly up-regulated in isotonic CI-AKI rat models. The expression of miRNA-188 was also highly expressed in CI-AKI cell models, which is in line with the expressions in rat model and in CI-AKI patients’ plasma found by Sun et al. (18). Western blot and TUNEL assay found, with significance, that an overexpression of miRNA-188 aggravates apoptosis in CI-AKI cell models. Additionally, MiRNA-188 overexpression directly inhibited the expression of SRSF7, as determined by a dual luciferase reporter assay, and SRSF7 silencing reduced the cell viability rate of HK-2 cell according to MTS assay.
We compared the novel isotonic CI-AKI rat model with the classical CI-AKI model and found a few interesting differences in biochemical indices and pathology (22). In order to explore the appropriate dosage and time for the isotonic CI-AKI rat model, we performed dose and time gradient experiments in our previous study. We found that isotonic CM iodixanol needed to be used in a higher dosage (15 mL/kg) than the high-osmotic CM iohexol (10 mL/kg) in order to get the same increase in creatinine levels and CI-AKI occurrence rate (15) (Figure 1A,B). Therefore, we verified that isotonic CM indeed has a lower renal toxicity and CI-AKI occurrence rate than the highly osmotic CM in animal models. The pathological changes, however, are different between these two CI-AKI models. The pathological changes of classical CI-AKI model are mainly manifested as renal tubular structure damage (15,22,23); we found more severe occlusion and necrosis in renal tubular HE staining and more detached microvilli and thicker glomerular basement membrane under electron microscope observation. Meanwhile, the pathological changes of isotonic CI-AKI mainly demonstrated an inflammatory reaction and cellular damage. HE staining illustrated more inflammatory cell aggregation, while electron microscope observation showed more mitochondrial swelling and more disordered mitochondrial cristae (Figure 1C,D). We hypothesized these differences were due to varying physicochemical characteristics. Iohexol are nonionic monomer contrast media with high osmotic pressure (830 mOsm/kg H2O) but lower viscosity (10 mPs at 37 °C), and thus may generate more severe direct renal tubular toxicity and cause more damage to the tubular structure. Iodixanol is an isotonic nonionic dimer contrast media with lower osmotic pressure (290 mOsm/kg H2O) but higher viscosity (11.8 mPs at 37 °C), which may take longer to excrete and cause more severe vasoconstriction and oxidative stress. However, relevant studies comparing the toxic effects of different CM are rare, and more research is needed to investigate and to validate these phenomena.
As the key regulatory function of MiRNA in disease occurrence and development has been recognized, and increased attention has been given to therapies which exploit the targeting of specific miRNAs. In vitro studies have found that both upregulating miRNA-126 by vesicles and inhibiting miRNA-709 by oligonucleotides can attenuate AKI (24,25), while the increase of miR-19a/19b expression by lipid-based delivery reagent can effectively improve cardiac function and reduce scar size in myocardial infarction among mice (26). Although the efficacy of miRNAs therapeutics has been demonstrated by many studies using in vitro and small animal experiments, the safety of miRNAs therapeutics in large animal model is still in doubt. Gabisonia found that microRNA-199a can stimulate cardiac repair myocardial infarction in pigs, yet subsequent persistent and uncontrolled expression of the microRNA resulted in sudden arrhythmic death of most of the treated pigs (27). Therefore, how to control the expression of miRNA in vivo and how to determine a safe dosage are issues which need to be urgently addressed; we should remain cautious and exercise restraint before the safety of miRNAs therapeutics can be validated.
This study had several limitations. First, it would have been better if there had been a miRNA-188 inhibition experiment. Since we have demonstrated the strong pro-apoptosis function in CI-AKI, an inhibition experiment could have more cogently illustrated the potential therapeutic effect exerted by miRNA-188. In fact, only one miRNA-188 inhibitor sequence was transfected (5'- CCCUCCACCAUGCAAGGGAUG-3'), but the expression change of miRNA-188 and biological effect was not validated by RT-qPCR, western blot, or TUNEL assay. According a review of the literature, we think the reason for the unsuccessful inhibition experiment could be that miRNA-188 may need post-transcriptional modifications. Also, the new method of stable knockdown of microRNA by lentiviral-vector-incorporated miRT sequences may be a better way to solve this problem (28). Second, we only perform miRNA-188 overexpression and SRSF7 knocking down experiments in vitro. If the overexpression and knocking downing experiments could conducted both in isotonic CI-AKI rat models and renal tubular epithelial cells, it would be more convincing to conclude that miRNA-188 induce apoptosis by targeting SRSF7 in CI-AKI. Therefore, we will perform up and down regulation experiment both in animal model and cells in future, in order to determine the regulatory axis in vivo and vitro. Third, the present study did not explore the mechanism that leads to the elevation of miRNA-188 in CI-AKI. By using the RNA sequencing data of isotonic CI-AKI rat model obtained in our previous study, we conducted an endogenous competition RNA network analysis. Result showed that 3 long-noncoding RNAs (lncRNAs) LOC284581, XLOC_002253, and KCNQ1OT1 which can specifically bind miRNA-188 were significantly downregulated in CI-AKI. Consequently, we suspect that the dysregulated lncRNAs trigger the miRNA-188-SRSF7 apoptosis axis in CI-AKI.
In conclusion, the present study indicated that miRNA-188 aggravated CM-induced apoptosis by regulating SRSF7, which may serve as a potential drug target for CI-AKI intervention.
Acknowledgments
Funding: This study was supported by the National Science Foundation for Young Scientists of China (grant no.81500520 and grant no. 81800325), the National Science Foundation of China (grant no. 81670339), the Science and Technology Planning Project of Guangdong Province (grant no. 2015A030302037 and grant no. 2014B070706010), Guangdong Provincial Medical Research Fund Project (grant no. GSIC20140526), Guangdong Provincial Research Fund for Science and Technology (grant no. 2016111016281122), and Guangdong Provincial People’s Hospital Clinical Research Project (grant no. 2014dzx02).
Footnote
Conflicts of Interest: The authors have no conflicts of interest to declare.
Ethical Statement: Authors are accountable for all aspects of the work (if applied, including full data access, integrity of the data and the accuracy of the data analysis) in ensuring that questions related to the accuracy or integrity of any part of the work are appropriately investigated and resolved. The protocol was approved by the Guangdong General Hospital Ethics Research Committee (No. 2015253).
References
- McCullough PA, Choi JP, Feghali GA, et al. Contrast-Induced Acute Kidney Injury. J Am Coll Cardiol 2016;68:1465-73. [Crossref] [PubMed]
- Rear R, Bell RM, Hausenloy DJ. Contrast-induced nephropathy following angiography and cardiac interventions. Heart 2016;102:638-48. [Crossref] [PubMed]
- Rihal CS, Textor SC, Grill DE, et al. Incidence and prognostic importance of acute renal failure after percutaneous coronary intervention. Circulation 2002;105:2259-64. [Crossref] [PubMed]
- Sun G, Chen P, Wang K, et al. Contrast-Induced Nephropathy and Long-Term Mortality After Percutaneous Coronary Intervention in Patients With Acute Myocardial Infarction. Angiology 2019;70:621-6. [Crossref] [PubMed]
- Scharnweber T, Alhilali L, Fakhran S. Contrast-Induced Acute Kidney Injury: Pathophysiology, Manifestations, Prevention, and Management. Magn Reson Imaging Clin N Am 2017;25:743-53. [Crossref] [PubMed]
- Nijssen EC, Rennenberg RJ, Nelemans PJ, et al. Prophylactic hydration to protect renal function from intravascular iodinated contrast material in patients at high risk of contrast-induced nephropathy (AMACING): a prospective, randomised, phase 3, controlled, open-label, non-inferiority trial. Lancet 2017;389:1312-22. [Crossref] [PubMed]
- Subramaniam RM, Suarez-Cuervo C, Wilson RF, et al. Effectiveness of Prevention Strategies for Contrast-Induced Nephropathy: A Systematic Review and Meta-analysis. Ann Intern Med 2016;164:406-16. [Crossref] [PubMed]
- Thomson DW, Dinger ME. Endogenous microRNA sponges: evidence and controversy. Nat Rev Genet 2016;17:272-83. [Crossref] [PubMed]
- Dragomir MP, Knutsen E, Calin GA. SnapShot: Unconventional miRNA Functions. Cell 2018;174:1038-1038.e1. [Crossref] [PubMed]
- Brandenburger T, Salgado Somoza A, Devaux Y, et al. Noncoding RNAs in acute kidney injury. Kidney Int 2018;94:870-81. [Crossref] [PubMed]
- Bhatt K, Mi QS, Dong Z. microRNAs in kidneys: biogenesis, regulation, and pathophysiological roles. Am J Physiol Renal Physiol 2011;300:F602-10. [Crossref] [PubMed]
- Fang F, Chang RM, Yu L, et al. MicroRNA-188-5p suppresses tumor cell proliferation and metastasis by directly targeting FGF5 in hepatocellular carcinoma. J Hepatol 2015;63:874-85. [Crossref] [PubMed]
- Wang K, Liu CY, Zhou LY, et al. APF lncRNA regulates autophagy and myocardial infarction by targeting miR-188-3p. Nat Commun 2015;6:6779. [Crossref] [PubMed]
- Ulusoy S, Ozkan G, Mungan S, et al. GSPE is superior to NAC in the prevention of contrast-induced nephropathy: might this superiority be related to caspase 1 and calpain 1?. Life Sci 2014;103:101-10. [Crossref] [PubMed]
- Sun S, Zhang T, Nie P, et al. A novel rat model of contrast-induced acute kidney injury. Int J Cardiol 2014;172:e48-50. [Crossref] [PubMed]
- Yamasowa H, Shimizu S, Inoue T, et al. Endothelial nitric oxide contributes to the renal protective effects of ischemic preconditioning. J Pharmacol Exp Ther 2005;312:153-9. [Crossref] [PubMed]
- Rosado Ingelmo A, Doña Diaz I, Cabañas Moreno R, et al. Clinical Practice Guidelines for Diagnosis and Management of Hypersensitivity Reactions to Contrast Media. J Investig Allergol Clin Immunol 2016;26:144-55; quiz 2 p following 155.
- Sun SQ, Zhang T, Ding D, et al. Circulating MicroRNA-188, -30a, and -30e as Early Biomarkers for Contrast-Induced Acute Kidney Injury. J Am Heart Assoc 2016;5. [Crossref] [PubMed]
- Geenen RWF, Kingma HJ, van der Molen AJ. Pathophysiology of Contrast-Induced Acute Kidney Injury. Interv Cardiol Clin 2014;3:363-7. [Crossref] [PubMed]
- Haller C, Hizoh I. The cytotoxicity of iodinated radiocontrast agents on renal cells in vitro. Invest Radiol 2004;39:149-54. [Crossref] [PubMed]
- Sendeski MM. Pathophysiology of renal tissue damage by iodinated contrast media. Clin Exp Pharmacol Physiol 2011;38:292-9. [Crossref] [PubMed]
- de Souza Santos V, Peters B, Côco LZ, et al. Silymarin protects against radiocontrast-induced nephropathy in mice. Life Sci 2019;228:305-15. [Crossref] [PubMed]
- Ma X, Jiao Z, Liu Y, et al. Probucol Protects Against Contrast-Induced Acute Kidney Injury via the Extracellular Signal-Regulated Kinases 1 and 2 (ERK1/2)/JNK-Caspase 3 Pathway in Diabetic Rats. Med Sci Monit 2019;25:1038-45. [Crossref] [PubMed]
- Cantaluppi V, Gatti S, Medica D, et al. Microvesicles derived from endothelial progenitor cells protect the kidney from ischemiareperfusion injury by microRNA-dependent reprogramming of resident renal cells. Kidney Int 2012;82:412-27. [Crossref] [PubMed]
- Guo Y, Ni J, Chen S, et al. MicroRNA-709 mediates acute tubular injury through effects on mitochondrial function. J Am Soc Nephrol 2018;29:449-61. [Crossref] [PubMed]
- Gao F, Kataoka M, Liu N, et al. Therapeutic role of miR-19a/19b in cardiac regeneration and protection from myocardial infarction. Nat Commun 2019;10:1802. [Crossref] [PubMed]
- Gabisonia K, Prosdocimo G, Aquaro GD, et al. MicroRNA therapy stimulates uncontrolled cardiac repair after myocardial infarction in pigs. Nature 2019;569:418-22. [Crossref] [PubMed]
- Gentner B, Schira G, Giustacchini A, et al. Stable knockdown of microRNA in vivo by lentiviral vectors. Nat Methods 2009;6:63-6. [Crossref] [PubMed]