Reactive astrocytes foster brain metastases via STAT3 signaling
Brain metastasis remains a grim and complicating factor in many cancers, regardless of the primary tumor origin (1). Moreover, the unique brain microenvironment inadvertently aides in brain metastasis growth and progression (2). In a recently published paper by Priego et al. (3), it was uncovered that there is a subpopulation of reactive astrocytes (RA) that surround brain metastases and are driven by signal transducer and activator of transcription 3 (STAT3) activation. The metastatic tumor cells in the brain secrete various factors that trigger astrocytes to become reactive and display enhanced STAT3 activation. These STAT3+ RA produce cytokines and other factors that inhibit an appropriate anti-tumor immune response. Both innate and adaptive immune functions are compromised due to the activities of these STAT3+ RA. Targeting the STAT3 signaling pathway in the microenvironment helps reduce pro-tumorigenic signaling and decreases brain metastasis burden.
This study first examined the RA population in several brain metastasis tumor samples from patients with lung cancer, breast cancer and melanoma (3). They found that all brain metastases contained RA, but that there was a distinct population of the RA that stained positive for STAT3 activation, i.e., tyrosine (Y705) phosphorylation. Interestingly, the staining for total STAT3 in RA was primarily nuclear, indicating ongoing transcriptional activity, whereas total STAT3 staining in the tumor cells of the brain metastasis was diffuse, non-nuclear, and cytoplasmic. The authors replicated their findings in mouse models of intracranial metastasis and again found a subpopulation of RA that displayed strong STAT3 activation. This finding is noteworthy because numerous studies implicate STAT3 as a driver of oncogenesis primarily in the tumor cells themselves (4). In primary brain tumors such as glioblastoma (GBM), STAT3 has been shown to be a driver of the mesenchymal subtype of tumors (5-7). The observance of strong STAT3 activation in the surrounding RA highlights an important role for the influence of microenvironmental STAT3 activation.
When STAT3 was deleted in the RA, brain metastasis burden was diminished. Mice with conditionally deleted STAT3 in glial fibrillary acidic protein (GFAP) positive astrocytes had reduced tumor growth compared to those with STAT3 activated RA. Upon examining the tumor cell secretome, they found that several factors were being secreted by the tumor cells that resulted in STAT3 activation in RA, including epidermal growth factor (EGF), transforming growth factor alpha (TGF-α), ciliary neurotrophic factor (CNTF) and interleukin-6 (IL-6) (Figure 1). This was confirmed in vitro by an observed increase in STAT3 activation in cultured astrocytes incubated with conditioned media collected from the tumor cells.
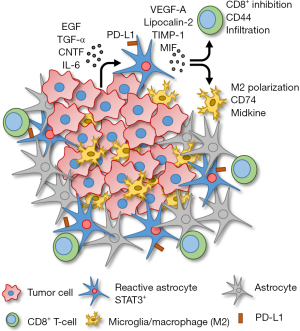
The subpopulation of STAT3+ RA produce several immunosuppressive cytokines as well as cell surface expression of programmed cell death 1-ligand 1 (PD-L1) that would potentially prevent an immune response (Figure 1). The STAT3+ RA decrease the activation and behavior of cytotoxic CD8+ T-cells. Specifically, CD44 expression (activation marker) on CD8+ T-cells is decreased, and STAT3+ RA physically act as a barrier, surrounding the brain metastasis mass, preventing infiltration of cytotoxic CD8+ T-cells into the tumor core. STAT3+ RA also secrete macrophage migration inhibitory factor (MIF), the CD74 ligand, that results in increased midkine expression and a skewed M2 (immunosuppressive) tumor associated macrophage/microglia phenotype. Therefore, STAT3+ RA in brain metastases prevent both adaptive (CD8+ T-cell) and innate (macrophage/microglia) immune responses, allowing unrestricted growth of the tumor.
Astrocytic STAT3 activation has also been linked to promoting an inflammatory response and exacerbating disease in other neuroinflammatory and acute brain injury models. For example, STAT3 is activated in RA in mouse models of Alzheimer’s and Huntington’s disease, and inhibition of astrocytic STAT3 decreased plaque accumulation and rescued spatial learning and memory decline in an Alzheimer’s disease model (8,9). In an ischemic stroke mouse model, STAT3 deletion in astrocytes resulted in reduced stroke volume and improved motor function, indicating astrocytic STAT3 activation promotes an inflammatory toxic effect in this model (10). Thus, STAT3 activation in a subpopulation of RA in brain tumor metastasis further validates the importance of the astrocyte and STAT3 in the brain microenvironment.
Next, therapeutic intervention targeting STAT3+ RA was assessed. Silibinin is a nutraceutical product (naturally occurring polyflavonoid found in plants such as milk thistle) that crosses the blood-brain barrier (BBB) (11), and has been shown to inhibit STAT3 activity through inhibition of the DNA binding properties of STAT3 (12,13). Treating mice with silibinin inhibited STAT3 activation in the RA surrounding the brain metastasis, and resulted in decreased brain metastasis growth and disease burden (3). Interestingly, treating only tumor cells with silibinin did not decrease tumor cell growth or activation, indicating that the effects of silibinin on tumor growth are due to inhibition of the microenvironmental factors that promote tumor growth.
In a small cohort, patients with lung cancer brain metastasis were given silibinin (provided as Legasil) in addition to their already established standard of care treatments to determine a potential anti-tumor affect. All of the patients displayed different tumor histological and genetic profiles, and had failed previous treatments including radiation and chemotherapies. Given to patients as a single agent (palliative care n=3) or with additional chemotherapies (n=15), Legasil induced an overall response rate in the brain of 75% of the patients, including three complete responses and ten partial responses. In fact, the three patients who received Legasil as palliative care were able to add an additional chemotherapeutic agent due to general status improvement and response seen on magnetic resonance imaging (MRI). However, Legasil did not prevent additional tumor growth outside of the brain, and most patients did have tumor recurrence outside of the brain.
There is increasing evidence that the brain microenvironment, specifically RA, create a unique tumor microenvironment for brain metastases to thrive (2). In addition to STAT3 activation, there are other reports demonstrating a pro-tumorigenic role of astrocytes in brain tumors. For example, Gril et al. found that RA surrounding brain metastases upregulated sphingosine-1 phosphate receptor 3 (S1P3) expression (14). This resulted in an increase in IL-6 and CCL2 secretion and a dysregulated (tumor promoting) blood-tumor barrier. Interestingly, both IL-6 and CCL2 are STAT3 regulated genes, indicating possible cross-talk between S1P3 and STAT3 RA activation pathways. When S1P3 was inhibited in the RA, this reduced the activation and inflammatory response of the RA, and promoted a normalized blood-tumor barrier, which has implications for enhanced drug delivery (14). Future studies should examine combinatorial therapies targeting RA and tumor cells, with STAT3 inhibitors such as Legasil or others playing a primary role.
In conclusion, Priego et al. have elegantly uncovered a tumor promoting mechanism regarding brain metastasis, with RAs having a critical role (3). Tumor cells that have initiated as a brain macro-metastasis secrete cytokines that activate astrocytes in the surrounding area. The astrocytes then become reactive and produce cytokines that are immunosuppressive, block cytotoxic CD8+ T-cell function and access to the tumor mass, and secrete cytokines that promote a M2 (tumor-promoting) myeloid phenotype. These events ultimately produce an optimal microenvironment for the brain metastasis to grow. The authors make a compelling case that the tumor microenvironment is an active participant in tumor growth, and that pharmacologically targeting the microenvironment, in addition to tumor cells, should be considered in future clinical agendas. Lastly, given the immunosuppressive role of RA in brain metastases, it would also be attractive to assess inhibition of RA in combination with immunotherapies such as immune checkpoint inhibitors in clinical trials for patients with brain tumor metastases.
Acknowledgments
Funding: Dr. McFarland has support from the UAB Neuro-oncology Support Fund and Dr. Benveniste has support from National Institutes of Health grants R01CA194414, R01NS057563, and P50NS108675.
Footnote
Conflicts of Interest: The authors have no conflicts of interest to declare.
References
- Lowery FJ, Yu D. Brain metastasis: Unique challenges and open opportunities. Biochim Biophys Acta Rev Cancer 2017;1867:49-57. [Crossref] [PubMed]
- Wasilewski D, Priego N, Fustero-Torre C, et al. Reactive astrocytes in brain metastasis. Front Oncol 2017;7:298. [Crossref] [PubMed]
- Priego N, Zhu L, Monteiro C, et al. STAT3 labels a subpopulation of reactive astrocytes required for brain metastasis. Nat Med 2018;24:1024-35. [Crossref] [PubMed]
- Yu H, Lee H, Herrmann A, et al. Revisiting STAT3 signalling in cancer: new and unexpected biological functions. Nat Rev Cancer 2014;14:736-46. [Crossref] [PubMed]
- Carro MS, Lim WK, Alvarez MJ, et al. The transcriptional network for mesenchymal transformation of brain tumours. Nature 2010;463:318-25. [Crossref] [PubMed]
- Cooper LA, Gutman DA, Chisolm C, et al. The tumor microenvironment strongly impacts master transcriptional regulators and gene expression class of glioblastoma. Am J Pathol 2012;180:2108-19. [Crossref] [PubMed]
- Bhat KP, Salazar KL, Balasubramaniyan V, et al. The transcriptional coactivator TAZ regulates mesenchymal differentiation in malignant glioma. Genes Dev 2011;25:2594-609. [Crossref] [PubMed]
- Ben Haim L, Ceyzeriat K, Carrillo-de Sauvage MA, et al. The JAK/STAT3 pathway is a common inducer of astrocyte reactivity in Alzheimer's and Huntington's diseases. J Neurosci 2015;35:2817-29. [Crossref] [PubMed]
- Reichenbach N, Delekate A, Plescher M, et al. Inhibition of Stat3-mediated astrogliosis ameliorates pathology in an Alzheimer's disease model. EMBO Mol Med 2019;11. [Crossref] [PubMed]
- Rakers C, Schleif M, Blank N, et al. Stroke target identification guided by astrocyte transcriptome analysis. Glia 2019;67:619-33. [Crossref] [PubMed]
- Lee Y, Park HR, Chun HJ, et al. Silibinin prevents dopaminergic neuronal loss in a mouse model of Parkinson's disease via mitochondrial stabilization. J Neurosci Res 2015;93:755-65. [Crossref] [PubMed]
- Bosch-Barrera J, Menendez JA. Silibinin and STAT3: A natural way of targeting transcription factors for cancer therapy. Cancer Treat Rev 2015;41:540-6. [Crossref] [PubMed]
- Verdura S, Cuyas E, Llorach-Pares L, et al. Silibinin is a direct inhibitor of STAT3. Food Chem Toxicol 2018;116:161-72. [Crossref] [PubMed]
- Gril B, Paranjape AN, Woditschka S, et al. Reactive astrocytic S1P3 signaling modulates the blood-tumor barrier in brain metastases. Nat Commun 2018;9:2705. [Crossref] [PubMed]