NK-mediated antibody-dependent cell-mediated cytotoxicity in solid tumors: biological evidence and clinical perspectives
Introduction
Cell-mediated immune defence includes the antibody-dependent cell-mediated cytotoxicity (ADCC), where an immune system effector cell actively lyses a target cell after its binding, by specific antibodies, to the membrane-surface antigens.
ADCC requires three different players working together: the cell expressing target antigens, the antibody and the immune effector cells expressing Fc-gamma receptors (FcγR).
In cancer therapy, the target on the cell surface may be an antigen highly expressed by many tumors such as epidermal growth factor receptor (EGFR). The antibody may be a monoclonal antibody (mAb), preferentially of class IgG1 or IgG3 since these two antibodies link any kind of FcγR. The third actor is the effector cell, harbouring the FcγR. There are many different FcγRs (Ia, IIa-b-c, IIIa-b), including one (FcγR IIb) with a strong inhibitory effect. All the effector cell lineages but one harbour both activating and inhibitory FcγR. Noteworthy is the finding that the affinity of FcγR IIb to IgG1 is higher than the affinity of IgG1 with the activating receptors.
Unlike other effector cells, natural killer (NK) cells harbour only activating FcγRs (FcγR IIIa, also known as CD16a, and FcγR IIc, also known as CD32c) and therefore these cells are considered the most important effectors of ADCC in humans.
Our review will focus on NK cells, mAbs, possible mechanisms to enhance ADCC and preclinical and clinical data focusing on ADCC in vivo.
NK cells: innate, adaptive, or both?
NK cells are innate immune effector cells that are able to recognise and destroy abnormal cells, in particular tumor or virus-infected cells.
Different from antigen-specific T cells, NK cells are innate immune lymphocytes. In the germline, NK cells are not able to rearrange genes encoding receptors, and, thus, they do not recognize many antigens and molecules of the major histocompatibility complex (MHC) (1).
In humans, there are two important lineages of NK cells, classifiable according to the expression of CD56: CD56dim and CD56bright. CD56dim NK cells are either CD16+ or CD16−. They constitute around 90% of the NK cells in blood and primarily negotiate cytotoxicity. Conversely, CD56bright cells are CD16− or CD16dim and form 5–15% of total NK cells. They produce mostly cytokines and play a minor role in cytolytic processes (1).
Elimination, equilibrium and escape are the three phases currently proposed in the context of cancer immunoediting, which range from surveillance to escape. In the innate response, effector cells (i.e., NK cells) and secreted IFN-γ are able to initially eliminate transformed cells. This results, in the equilibrium phase, in immune sculpting and selection of tumour variants, that are less immunogenic and become resistant to immune effectors (2).
NK cells are genetically programmed to destroy cellular malignancies through unique surface receptors that, upon contacting a target cell, transmit either inhibitory or activating intracellular signals. Acquisition of the capacity to kill comes only after the NK cell is rendered tolerant to healthy self cells through interaction with naturally expressed MHC I molecules (1,2). In fact, NK cells mainly target cells with a low expression of class I MHC (MHC-I), or with expressions of MIC-A or MIC-B which represent markers of stressed cells (1). The directed exocytosis of cytolytic granules, with the subsequent release of granzymes and perforins, and the expression of cytotoxic effector molecules in response to activation such as Fas ligand, is the mechanism by which NK cells perforate the plasma membrane of the target cell and cause apoptosis (1). ADCC was firstly described as a mechanism of where effector cells secrete cytotoxic molecules and lyse antibody-coated target cells. However, it is now understood as a multi-tiered process involving coordination and crosstalk of immune cells. Moreover, in recent literature, NK cell activation has been shown to provoke functions akin to those of a memory cell (3-6).
Thus, a reassessment of the role of the NK cell even in adaptive immunity is necessitated by these observations, taking into account that NK cells do not lead to immunity on their own, do not carry antigen specific recognizing receptors and do not make memory on their own. They are innate cells that are part of the network but do not do the specific adaptive job.
The importance of NK cells in tumor immune surveillance was studied primarily in mice lacking NK cells that clearly demonstrated an increased susceptibility to cancer (7-9). In humans, NK cell deficiencies are tormented by acute persistent viral infections, in particular the herpes simplex virus (10).
NK cell effector properties can be made use of in treating certain tumors due to their ability to evoke ADCC. Antibody-dependent tumor killing through NK mediation takes place via various routes, including cytotoxic granule exocytosis, tumor necrosis factor (TNF) family death receptor signalling and the release of a pro-inflammatory cytokine, such as interferon-gamma (IFNγ). Target cell apoptosis is caused by both the uptake of granzymes and perforins by target cells and by TNF family death receptor signalling, whereas NK-cell-released IFNγ activate nearby immune cells to encourage antigen presentation and adaptive immune responses (11).
ADCC
Recent studies have confirmed the role of ADCC in clearing tumors and have concentrated on accentuating ADCC in this context.
ADCC depends on the bifunctional structure of IgG molecules. The binding of the mAbs via the fragment antigen-binding (Fab) portion to the antigen associated with the tumor on the target cell confers ADCC specificity. When both cell antigen and an activating FcγR are engaged respectively by the Fab and Fc portions of the mAb, ADCC is initiated, since this creates a bridge from the tumor cell to the effector cell. The recognition of target cells is then combined to a lytic attack on the target cell mounted by effector cells. ADCC does not depend on the immune complement system in which targets are also lysed but no other cell is required. ADCC requires an effector cell, mainly NK cells (due to the lack of inhibitory FcγR IIb) that typically interact with IgG antibodies (12) (Figure 1).
Clynes et al. [2000] (13) suggested that Fc is perhaps the dominant component in vivo, causing host responses to destroy target cells. Those researchers showed that in mice lacking FcγR I and FcγR III, there was a significant reduction in the anti-tumor effects of certain mAbs. Conversely, mice with a deficiency in the inhibitory receptor FcγR IIb were seen to have tumor growth inhibition and enhanced ADCC (14).
Several studies have demonstrated that the anti-tumor activity of specific mAbs is linked to high affinity FcRs genotype (14).
Much effort has been made on the basis of these findings to manipulate the Fc region to optimize their therapeutic effects, such us glycoengineered mAbs to improve their cytotoxicity and neonatal Fc receptors (FcRn) engineered to increase the Fc domain affinity and also their half-life (15,16).
Furthermore, recently a novel concept has come to the fore. Concurrently to the direct short-term effects of mAbs, these are now also regarded as being immunomodulatory molecules able to recruit Fc-receptor-expressing innate immune cells so as to bring about a long-term endogenous adaptive immune response (with an effect like a vaccine), which is accountable for tumor development being controlled in a better and more sustained way as observed in certain patients (16).
Monoclonal Abs functioning through ADCC
Following binding to transformed cells or following neutralizing trophic signals delivered by the tumor stroma, mAbs targeting tumors provoke antineoplastic effects. As a result, there may be various mechanisms determining the clinical efficacy of these agents, which are often not mutually exclusive (17).
It has recently become clear that mAbs might exert action mechanisms different from the selective blockade of cell membrane receptors.
Following rituximab (anti-CD20), approved in 1997 for the treatment of non-Hodgkin’s lymphoma (18-20), various mAbs, including trastuzumab (21), cetuximab (22), the anti-GD2-mAbs (23) and daratumumab (anti-CD38 mAb) (24) have become the standard care in treating both solid and hematological malignancies [for an updated list, refer to (25)].
The Fc region may also utilise NK-mediated ADCC as a mechanism of action. Thus, NK cells are influential effectors in the immune response to tumors driven by mAbs, and data highlighting their importance continue to amass (1,8,26).
Despite the fact that various effective antibodies have come to the fore, responses that are long-term and durable are hard to determine; thus, resistance and relapse continue to represent significant limitations in cancer therapy (27).
In particular, cetuximab, as well as blockading downstream EGFR signalling, has several clinically significant immune mechanisms: (I) activating the FcγR IIIa on NK cells and ADCC; (II) IFN-γ secretion by activated NK cells, enhancing antigen presentation and upregulation of antigen processing machinery (APM) in dendritic cells (DCs); (III) cross-priming of NK and DCs, activating tumor-associated (TA)-specific cytotoxic lymphocytes (CTLs) (28).
It is important that we continue to understand the factors affecting the response to Ab-coated tumor cells in their environment in order to create the next generation of mAbs and new therapeutic strategies to stimulate immune effector cells involved in ADCC.
NK-mediated ADCC as potential predictors of a successful clinical outcome
A few studies suggested a potential role of ADCC as prognosticators (collected in Table 1). For instance, considering cetuximab, available experimental and clinical data agree that there is a direct correlation between the receptor density on the target cell membrane and the intensity of the ADCC response. Similarly, in both oropharyngeal cancer and colorectal cancer (CRC), it has been reported that a large-scale intratumoral infiltration of NK cells is associated with improved prognosis.
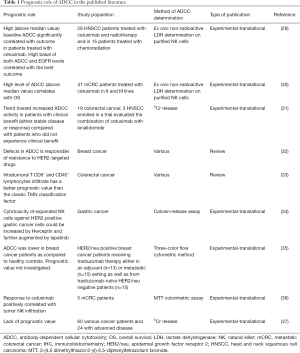
Full table
It is still unclear whether ADCC induced by cetuximab is associated with mutations in of RAS and BRAF genes in metastatic CRC (mCRC) (30,38). ADCC activities were shown to be significantly linked to the membrane expression of EGFR but not to the KRAS nor BRAF mutations. Conversely, Kasper et al. [2013] (39) suggest that RAS mutation defends at the same time against anti-EGFR antibody-dependent cellular cytotoxicity and EGFR signalling blockade.
Immunotargeting of NK cells, γδ T cells, macrophages and DCs can enhance the anticancer properties of mAbs and elicit an effective immune response (40). NKT cells are a subpopulation of T lymphocytes that co-express αβ T-cell receptor (TCR) and markers that are usually associated with NKs. They have a wide range of immune effector properties. In particular, a subset of CD1d-dependent NKT cells exist which expresses an invariant TCR α chain (iNKT) cells. They rapidly respond to stress and inflammatory signals (41).
A number of independent studies have shown that a reduction of number of iNKT cells in the blood of patients with a variety of cancers. Interestingly, an increased frequency of peripheral blood iNKT cells heralds a more favourable response to therapy (42). Molling et al. (43) correlated a severe circulating iNKTs deficiency with poor clinical outcome in head and neck squamous cell carcinoma. Lo Nigro et al. (30,44) assessed the extent to which cetuximab-mediated ADCC and circulating iNKT cell levels have valid prognostic and predictive properties in mCRC treated with cetuximab in II and III lines, and examined how they correlate with EGFR level, KRAS/NRAS/BRAF mutational status, progression-free survival (PFS) and overall survival (OS) in a prospective cohort of mCRC patients undergoing treatment with therapy based on cetuximab. They noticed that, at basal level, the combination of iNKT number and ADCC be identified a group of patients which had both characteristics above the respective median level together with a longer OS. This advantage appeared to be greater than the role played by ADCC by itself, giving weight to the theory that there is a positive interchange between iNKT and ADCC effector cells. No correlation between key SNPs was seen to be implicated in ADCC ability and OS nor was PFS found to be significant.
With the same approach, Lattanzio et al. [2017] (29) more recently delineated baseline ADCC in 28 cetuximab- and radiotherapy (RT)-treated HNSCC patients and in 15 chemoradiation-treated patients and associated the values found with complete response and OS. They also considered the role of EGFR expression and studied the combined effect of EGFR and ADCC. High (above median value) baseline ADCC significantly correlated with outcome with respect to low (below median value), though not in non-cetuximab-treated patients. It was interesting to note that those patients with both high baseline ADCC and EGFR3+ levels obtained the best outcome with respect to the others.
At present, we do not know the mechanistic link sustaining the positive value of iNKT described above, and dedicated translational studies are needed in this direction.
Strategies potentially enhancing ADCC
We will evaluate and describe some of the main potential approaches to enhance the antitumor activity of ADCC [for a complete review, refer to (25)].
Such strategies may involve attempts to augment the receptor affinity and activation of NK, accessibility to tumor site, combination with other molecules and immune-modulatory strategies, i.e., RT and checkpoint blockage.
Receptor affinity
An effective approach to increasing ADCC might be to alter the Fc fragment so as to enhance NK-mediated ADCC. For instance, engineering mAbs to remove fucose sugar units from the Fc region increases binding with FcγR and therefore ADCC. Mogamulizumab (an anti-CCR4 mAb) is an afucosylated mAb which brought about ADCC against CCR4-positive cell lines successfully and hindered the growth of EBV-positive NK cell lymphomas in preclinical models (45,46).
CD137 is an activating receptor on the NK cell membrane (8,47). The anti-CD137 (4-1BB) antibody activated NKs in a mouse model of B-cell lymphoma (48,49). The co-activation with 4-1BB agonist antibody between rituximab cycles in vivo resulted in the NK-mediated complete regression of the subcutaneous murine lymphoma (1,48).
A new type II glycoengineered anti-CD20 mAb with increased FcγR III binding and ADCC is represented by obinutuzumab (GA101). Unlike rituximab, GA101 induces NK cell activation regardless of the cells’ inhibitory immunoglobulin-like receptor (KIR) expression. Furthermore, its activity is not adversely modulated by KIR/HLA (25,46).
Several other promising combination strategies aimed at boosting NK cell ADCC responses to tumors are now being developed pre-clinically and clinically [for a complete list, refer to (50)].
mAb + radiation therapy
An anti-tumor effect is obtained by radiation (RT) due to DNA damage being caused but the same RT may increase tumor susceptibility to immune response too. As a result, the immune response might modulate the effect of RT, and RT may increase immunotherapy efficacy (51) perhaps by causing inflammatory cytokines to be produced and tumor-specific antigens to be released, as well as causing changes in the expression of immune susceptibility markers and in the on vasculature, which enhance immune control (52).
Moreover, RT upregulates the expression of tumor-specific antigens, MHC class I, NKG2D ligand and the Fas death receptor; all these mechanisms have been shown to potentiate ADCC in experimental models (20).
mAb + matrix metalloproteases (MMPs) inhibitor
It has been demonstrated that NK cells, once activated, discard FcγR IIIa from the cell membrane by activating MMPs (20,53,54). NK cell in vitro treatment with the MMP inhibitor, GM6001, redeems FcγR IIIa loss provoked by K562 tumor cells, but does not impede NKs degranulation, which points to the fact that FcγR IIIA shedding and NK cell degranulation are independent. It is, therefore, reasonable to suppose that by maintaining FcγR IIIa on the NK cell surface through an MMP inhibitor, the NK-dependent ADCC might be enhanced without interfering with degranulation (55). Recently, Romee et al. [2013] determined one specific MMP, ADAM17, as the key MMP mediating the FcγR IIIa loss after NKs activation (19).
Combination of immune checkpoint inhibitors and NK cell therapy
PD-1 and ligands (PD-L1 and PD-L2) represent another important signalling pathway able to regulate NK cell and, as consequence, ADCC. It is now believed that PD-1 and PD-L1 blockade firstly impact T-cell subsets, but, after activation, NK cells express PD-1 and send negative regulatory signals, which limit cytotoxicity (49,56).
In the study by Guo et al. [2016] (57), the introduction of the anti-PD1 antibody to NK cell therapy improves cells’ in vitro activity (i.e., higher levels of the NK activation receptors NKG2D, NKp44 and NKp30). They also incremented NK-mediated ADCC and drug efficacy in a in vivo model of multiple myeloma (MM) and in primary MMs (1,50).
In particular, it was seen that PD-1 was upregulated on NK cells in patients with MMs expressing PD-L1, and the NK cell–mediated cytotoxicity of these tumors was shown to be potentiated by a PD-1 blocking antibody (58).
In patients with MM, renal cell carcinoma and follicular lymphoma cytolytic activity of NK cells was restored by autologous NK cells with blocking anti-PD-1 antibodies (pidilizumab), alone or combined with rituximab (59,60).
Anti-NKG2A antibodies
CD94/NKG2A is an HLA-E-binding inhibitory receptor. It was seen that, in several solid tumors and hematological malignancies, HLA-E might inhibit the cytotoxic properties of NKs and CD8+ cytotoxic T lymphocytes (61). Monalizumab (IPH2201), anti-NKG2A checkpoint inhibitor, has been evaluated in clinical trials in ovarian cancers, head and neck cancers, advanced malignancies and chronic lymphocytic leukemia (CLL) (62). Its use might represent a novel approach in NK-based immunotherapy not only enhancing the cytotoxic potential of the cells, but also hypothesizing a role tor them in ADCC augmentation (50).
Killer cell immunoglobulin-like receptors (KIRs)
The KIR family are key modulators of NKs activation. KIR molecules bind to the self MHC class I ligands (HLA-A, HLA-B, HLA-C) and transmit inhibitory signals that abrogate the effects of activating receptors. The application of blocking antibodies toward KIRs is the basis of an alternative checkpoint inhibitory strategy. Since MHC class I is virtually represented on all healthy cells, KIR molecules are regarded as being one of the principal mechanisms responsible for NK cell tolerance to self. In mice models of leukemia, it has been shown that the reduction of KIR-mediated inhibitory signals in NK cells through antibody blockade increases NK cytotoxicity and possibly NK cell-mediated ADCC. A KIR-blocking mAb (IPH2102/BMS-986015), lirilumab, has been tested in clinical research (8,27). Human NK cell function in vitro, in humanized mice, and, most recently, in clinical experimental patient sets, was shown to be boosted by another anti-KIR antibody (IPH2101) that blocks MHC-I recognition (1).
Glucocorticoid-induced TNF receptor family related gene (GITR)
Following cellular activation, the GITR is upregulated (27,63). GITR ligand (GITRL) is often represented on leukemia cells in AML and CLL, and weakens the activity of expressing GITR-NK cells (64). GITRL additionally inhibits the ADCC of NK cells induced by rituximab (65). In NK cells, the anti-GITR mAb TRX518 prevents GITR interaction, with its ligand GITRL, thus augmenting NK-cell cytotoxicity and intensifying NK cell-mediated ADCC. A phase I study with TRX518 (NCT01239134) was carried out in melanoma (27).
CD27
CD27 (TNFRSF7), a costimulatory receptor on T cells, B cells and NKs, supplies a target for intensifying anticancer immunity (27,66). CD27 and interaction with its ligand, CD70, accelerated NK-mediated tumor clearance whilst causing an adaptive immune response. Anti-CD27 antagonist therapy strongly induced ADCC in clinical trials of patients with solid tumors and hematologic cancers. In clinical trials, in solid and hematologic cancers, anti-CD27 treatment increased ADCC dramatically (67).
Bruton’s tyrosine kinase (BTK) inhibitor
Ibrutinib, an irreversible BTK inhibitor, displays encouraging activity in CD20+ B-cell tumors and recently gained US FDA approval in mantle cell lymphoma (68). Ibrutinib inhibited NK cell cytokine secretion and cytotoxicity induced by both rituximab and trastuzumab. Yonezawa et al. [2016] (27) showed that the annulment of this antitumor efficacy is the result of the inhibition by ibrutinib of FcR-stimulated NK cell function, in particular ADCC.
Adoptive transfer of ex vivo-activated NK cells
Processes of ex vivo cytokine activation and expansion of NK cells have characterised recent approaches. Several cytokines [interleukin-2 (IL-2), IL-12, IL-15, IL-18, IL-21 and type I IFNs] have been investigated to activate and expand NK cells ex vivo. IL-2 (46) is the most widely-studied cytokine, as the co-administration of mAb and IL-2 improves the NK cells’ antitumor activity (8,69,70).
ADCC activity against mAb-coated tumor cells has been increased by IL-2-activated lymphokine-activated killer (LAK) cells (71).
Recent efforts have concentrated on bettering methods for ex vivo NK cells expansion from peripheral blood mononuclear cells (PBMCs) in order to produce significant amounts of purified and functionally active NKs for clinical practice.
Following early reports of successful adoptive NK-cell transfer, there has been considerable pre-clinical investigation carried out by many groups into the ideal manufacturing procedures for NK cell ex vivo manipulation. Various methods were optimized in the preclinical context and have been successfully transferred to the clinic (72-78).
In the process of NK expansion, effectors are activated to kill tumors more effectively, so that it is possible to infuse them into patients. It may be that autologous NK cells are partially tolerant to self-tumors and, in some contexts, have a lower anti-cancer activity with respect to the allogeneic ones (20).
It has been demonstrated in various investigations that the infusion of enriched haploidentical NK cells, particularly those taking advantage of KIR/HLA mismatches, is safe and effective in children, adults and elderly cancer patients (75-77). Despite the fact that this is an encouraging approach, the need for specialized procedures and equipment and good manufacturing practices, together with the various logistical issues, has concentrated these adoptive NK cell therapies into few specialized centres (78). Notwithstanding this, it has been shown that the centralized manipulation, conservation and safe shipping of active NKs for infusion to distant hospitals is possible and the improved practice might become more common (79).
Genetically-engineered killer engagers
Based upon genetically-engineered bispecific killer engagers (BiKEs) and trispecific killer engagers (TriKEs), alternative approaches have emerged to augment the activity and killing specificity of NK cells [for a complete review, refer to (50)]. Conjugate formation between tumor and NK cells is promoted by those antibodies. They normally bind both the FcγR IIIa/CD16 and tumor antigen (80). A bispecific tetravalent antibody targeting both CD16 and CD30 was recently introduced in relapsed Hodgkin lymphoma (81). Greater cytotoxicity was displayed by those constructs with respect to conventional antibodies that are independent of the FcγR IIIa (82).
Furthermore, a tetraspecific killer engager (TetraKE) based on a bispecific NK cell engager (BiKE) platform, consisting of single-chain variable fragments binding FcγR III on NK cells, EpCAM on carcinoma cells and CD133 on cancer stem cells, was created so as to increase ADCC (83). BiKEs were constructed to create an immune bridge between cancer and NK cells. Additionally, NK cell-related proliferation was enhanced by an IL-15-crosslinker. IL-15 regulates NK cells, which can augment ADCC, LAK activity and IFN, TNF and GM-CSF signalling (84-86). All of these functions activated by IL-15 play a part in improving cancer defence.
Conclusions
mAbs evolved from original mouse antibodies, chimeric antibodies, humanized and fully human antibodies. However, their evolution to a more efficient weapon is still in progress. The present challenge is to engineer them to improve anti-tumor efficacy. Other methods of improving anti-tumor effects exist in addition to optimising the mAb itself, one of which is to improve ADCC by coupling mAbs with immune stimulatory agents.
Though ADCC is to date overshadowed by the exciting results of target immunotherapy, such as immune check-point inhibitors, due to the recent progress in the NK cell biology and translational research, it is hoped that the therapy with NK cells become first line in the immunotherapy of cancer in the coming years.
The majority of current data, presented in this work, refer to circulating NK. An open issue and current challenge would be to explore the presence and role of NK at the tumor tissue level, comparing with findings in the peripheral blood.
A direct tumor infiltration by NK cells has been reported in several tumor settings but its biological role and clinical significance is still not completely clear and is sometimes controversial (87-89). For instance, similarities between intratumoral NK and tolerogenic NK present in the maternal decidua have been described (89).
Recently, it was reported that intratumoral NK may cluster together with stimulatory DCs sustaining their positive role, through the production of FLT3L, toward an effective T cell-mediated antitumor activity and response to checkpoint inhibitors (90).
The integration in the clinical studies with next generation IHC methodologies and multiplex analyses will be crucial to advance our knowledge of these topics.
Most probably, the beneficial effects in cancer therapy might be applied by a combination of ex vivo stimulated NK cells and other immune drugs (i.e., mAbs and immune checkpoint inhibitors) and/or standard therapies (i.e., radio- and chemo-therapy).
ADCC might be improved by drugs able to enhance NK cell activity, as they are regarded as having a significant role in mAb-mediated ADCC against tumor cells.
In particular, KIRs and FcR genotypes may be useful in forecasting the clinical outcome of ADCC-inducing mAbs, thus enabling a more personalized therapy.
Following continuing preclinical research into the potential synergy of combinations of drugs, and the mechanisms at their base, the rational design of early-phase clinical trials and the use of robust biomarkers (e.g., increase, activation or cytolytic function of NK cell populations) will be enabled. Any combinations displaying the proof-of-principle will be moved on to later-phase trials and will perhaps confirm if a true synergy exists.
Without doubt some mAbs/immunomodulatory drugs combinations, as reviewed in this paper, will become an important tool in anticancer therapies over the next few years.
The main challenge remaining is the difficulty to distinguish in the clinical setting, between the target effect that many mAbs exert against specific cell membrane receptors and the ADCC effect that they also can induce.
Therefore, despite the fact that the evidence existing in animal models and the many small studies in humans continue to provide convincing weight, the real strength of ADCC in clinical practice remains unclear.
Acknowledgements
We thank Laurence Preston for the English revision of the manuscript.
Footnote
Conflicts of Interest: The authors have no conflicts of interest to declare.
References
- Campbell KS, Hasegawa J. Natural killer cell biology: an update and future directions. J Allergy Clin Immunol 2013;132:536-44. [Crossref] [PubMed]
- Kim R, Emi M, Tanabe K. Cancer immunoediting from immune surveillance to immune escape. Immunology 2007;121:1-14. [Crossref] [PubMed]
- O'Leary JG, Goodarzi M, Drayton DL, et al. T cell- and B cell-independent adaptive immunity mediated by natural killer cells. Nat Immunol 2006;7:507-16. [Crossref] [PubMed]
- Cooper MA, Elliott JM, Keyel PA, et al. Cytokine-induced memory-like natural killer cells. Proc Natl Acad Sci U S A 2009;106:1915-9. [Crossref] [PubMed]
- Raulet DH. Natural Killer Cells: Remembrances of Things Past. Curr Biol 2009;19:R294-6. [Crossref] [PubMed]
- Sun JC, Beilke JN, Lanier LL. Adaptive immune features of natural killer cells. Nature 2009;457:557-61. [Crossref] [PubMed]
- Trapani JA, Voskoboinik I. Infective, neoplastic, and homeostatic sequelae of the loss of perforin function in humans. Adv Exp Med Biol 2007;601:235-42. [Crossref] [PubMed]
- Alderson KL, Sondel PM. Clinical cancer therapy by NK cells via antibody-dependent cell-mediated cytotoxicity. J Biomed Biotechnol 2011;2011:379123. [Crossref] [PubMed]
- Macagno M, Bandini S, Stramucci L, et al. Multiple roles of perforin in hampering ERBB-2 (Her-2/neu) carcinogenesis in transgenic male mice. J Immunol 2014;192:5434-41. [Crossref] [PubMed]
- Orange JS. Human natural killer cell deficiencies and susceptibility to infection. Microbes and Infection 2002;4:1545-58. [Crossref] [PubMed]
- Stevenson GT. Three major uncertainties in the antibody therapy of cancer. Haematologica 2014;99:1538-46. [Crossref] [PubMed]
- Mellor JD, Brown MP, Irving HR, et al. A critical review of the role of Fc gamma receptor polymorphisms in the response to monoclonal antibodies in cancer. J Hematol Oncol 2013;6:1. [Crossref] [PubMed]
- Clynes RA, Towers TL, Presta LG, et al. Inhibitory Fc receptors modulate in vivo cytotoxicity against tumor targets. Nat Med 2000;6:443-6. [Crossref] [PubMed]
- Eccles SA. Monoclonal antibodies targeting cancer: ‘magic bullets’ or just the trigger? Breast Cancer Res 2001;3:86-90. [Crossref] [PubMed]
- Monnet C, Jorieux S, Souyris N, et al. Combined glyco- and protein-Fc engineering simultaneously enhance cytotoxicity and half-life of a therapeutic antibody. MAbs 2014;6:422-36. [Crossref] [PubMed]
- Michaud HA, Eliaou JF, Lafont V, et al. Tumor antigen-targeting monoclonal antibody-based immunotherapy: Orchestrating combined strategies for the development of long-term antitumor immunity. Oncoimmunology 2014;3:e955684. [Crossref] [PubMed]
- Vacchelli E, Bloy N, Aranda F, et al. Trial Watch: Immunotherapy plus radiation therapy for oncological indications. Oncoimmunology 2016;5:e1214790. [Crossref] [PubMed]
- Cartron G, Dacheux L, Salles G, et al. Therapeutic activity of humanized anti-CD20 monoclonal antibody and polymorphism in IgG Fc receptor FcgammaRIIIa gene. Blood 2002;99:754-8. [Crossref] [PubMed]
- Romee R, Foley B, Lenvik T, et al. NK cell CD16 surface expression and function is regulated by a disintegrin and metalloprotease-17 (ADAM17). Blood 2013;121:3599-608. [Crossref] [PubMed]
- Wang W, Erbe AK, Hank JA, et al. NK cell-mediated antibody-dependent cellular cytotoxicity in cancer immunotherapy. Frontiers in Immunology 2015;6:368. [Crossref] [PubMed]
- Junttila TT, Parsons K, Olsson C, et al. Superior in vivo efficacy of afucosylated trastuzumab in the treatment of HER2- amplified breast cancer. Cancer Res 2010;70:4481-9. [Crossref] [PubMed]
- Messersmith WA, Ahnen DJ. Targeting EGFR in colorectal cancer. N Engl J Med 2008;359:1834-6. [Crossref] [PubMed]
- Koehn TA, Trimble LL, Alderson KL, et al. Increasing the clinical efficacy of NK and antibody-mediated cancer immunotherapy: potential predictors of successful clinical outcome based on observations in high-risk neuroblastoma. Front Pharmacol 2012;3:91. [Crossref] [PubMed]
- van de Donk NW, Janmaat ML, Mutis T, et al. Monoclonal antibodies targeting CD38 in hematological malignancies and beyond. Immunol Rev 2016;270:95-112. [Crossref] [PubMed]
- Terszowski G, Klein C, Stern M. KIR/HLA interactions negatively affect rituximab- but not GA101 (obinutuzumab)-induced antibody-dependent cellular cytotoxicity. J Immunol 2014;192:5618-24. [Crossref] [PubMed]
- Beano A, Signorino E, Evangelista A, et al. Correlation between NK function and response to trastuzumab in metastatic breast cancer patients. J Transl Med 2008;6:25. [Crossref] [PubMed]
- Yonezawa A, Chester C, Rajasekaran N, et al. Harnessing the innate immune system to treat cancer: enhancement of antibody-dependent cellular cytotoxicity with anti-CD137 Ab. Chin Clin Oncol 2016;5:5. [PubMed]
- Gildener-Leapman N, Ferris RL, Bauman JE. Promising systemic immunotherapies in head and neck squamous cell carcinoma. Oral Oncol 2013;49:1089-96. [Crossref] [PubMed]
- Lattanzio L, Denaro N, Vivenza D, et al. Elevated basal antibody-dependent cell-mediated cytotoxicity (ADCC) and high epidermal growth factor receptor (EGFR) expression predict favourable outcome in patients with locally advanced head and neck cancer treated with cetuximab and radiotherapy. Cancer Immunol Immunother 2017;66:573-9. [Crossref] [PubMed]
- Lo Nigro C, Ricci V, Vivenza D, et al. Prognostic and predictive biomarkers in metastatic colorectal cancer anti-EGFR therapy. World J Gastroenterol 2016;22:6944-54. [Crossref] [PubMed]
- Bertino EM, McMichael EL, Mo X, et al. A Phase I Trial to Evaluate Antibody-Dependent Cellular Cytotoxicity of Cetuximab and Lenalidomide in Advanced Colorectal and Head and Neck Cancer. Mol Cancer Ther 2016;15:2244-50. [Crossref] [PubMed]
- Madrid-Paredes A, Cañadas-Garre M, Sánchez-Pozo A, et al. De novo resistance biomarkers to anti-HER2 therapies in HER2-positive breast cancer. Pharmacogenomics 2015;16:1411-26. [Crossref] [PubMed]
- Pernot S, Terme M, Voron T, et al. Colorectal cancer and immunity: what we know and perspectives. World J Gastroenterol 2014;20:3738-50. [Crossref] [PubMed]
- Mimura K, Kamiya T, Shiraishi K, et al. Therapeutic potential of highly cytotoxic natural killer cells for gastric cancer. Int J Cancer 2014;135:1390-8. [Crossref] [PubMed]
- Petricevic B, Laengle J, Singer J, et al. Trastuzumab mediates antibody-dependent cell-mediated cytotoxicity and phagocytosis to the same extent in both adjuvant and metastatic HER2/neu breast cancer patients. J Transl Med 2013;11:307. [Crossref] [PubMed]
- Maréchal R, De Schutter J, Nagy N, et al. Putative contribution of CD56 positive cells in cetuximab treatment efficacy in first-line metastatic colorectal cancer patients. BMC Cancer 2010;10:340. [Crossref] [PubMed]
- McCredie JA, MacDonald HR. Antibody-dependent cellular cytotoxicity in cancer patients: lack of prognostic value. Br J Cancer 1980;41:880-5. [Crossref] [PubMed]
- Seo Y, Ishii Y, Ochiai H, et al. Cetuximab mediated ADCC activity is correlated with the cell surface expression level of EGFR but not with the KRAS/BRAF mutational status in colorectal cancer. Oncol Rep 2014;31:2115-22. [Crossref] [PubMed]
- Kasper S, Breitenbuecher F, Reis H, et al. Oncogenic RAS simultaneously protects against anti-EGFR antibody-dependent cellular cytotoxicity and EGFR signaling blockade. Oncogene 2013;32:2873-81. [Crossref] [PubMed]
- Kohrt HE, Houot R, Marabelle A, et al. Combination strategies to enhance antitumor ADCC. Immunotherapy 2012;4:511-27. [Crossref] [PubMed]
- Carreño LJ, Kharkwal SS, Porcelli SA. Optimizing NKT cell ligands as vaccine adjuvants. Immunotherapy 2014;6:309-20. [Crossref] [PubMed]
- Altman JB, Benavides AD, Das R, et al. Antitumor Responses of Invariant Natural Killer T Cells. J Immunol Res 2015;2015:652875. [Crossref] [PubMed]
- Molling JW, Langius JA, Langendijk JA, et al. Low levels of circulating invariant natural killer T cells predict poor clinical outcome in patients with head and neck squamous cell carcinoma. J Clin Oncol 2007;25:862-8. [Crossref] [PubMed]
- Lo Nigro C, Ricci V, Vivenza D, et al. Evaluation of antibody-dependent cell-mediated cytotoxicity activity and cetuximab response in KRAS wild-type metastatic colorectal cancer patients. World J Gastrointest Oncol 2016;8:222-30. [Crossref] [PubMed]
- Kanazawa T, Hiramatsu Y, Iwata S, et al. Anti CCR-4 monoclonal antibody mogamulizumab for the treatment of EBV-associated T-and NK-cell lymphoproliferative diseases. Clin Cancer Res 2014;20:5075-84. [Crossref] [PubMed]
- Rezvani K, Rouce RH. The Application of Natural Killer Cell Immunotherapy for the Treatment of Cancer. Front Immunol 2015;6:578. [Crossref] [PubMed]
- Lin W, Voskens CJ, Zhang XE, et al. Fc-dependent expression of CD137 on human NK cells: insights into “agonistic” effects of anti-CD137 monoclonal antibodies. Blood 2008;112:699-707. [Crossref] [PubMed]
- Kohrt HE, Houot R, Goldstein MJ, et al. CD137 stimulation enhances the antilymphoma activity of anti-CD20 antibodies. Blood 2011;117:2423-32. [Crossref] [PubMed]
- Rajasekaran N, Chester C, Yonezawa A, et al. Enhancement of antibody-dependent cell mediated cytotoxicity: a new era in cancer treatment. Immunotargets Ther 2015;4:91-100. [PubMed]
- Shevtsov M, Multhoff G. Immunological and Translational Aspects of NK Cell-Based Antitumor Immunotherapies. Front Immunol 2016;7:492. [Crossref] [PubMed]
- Budach W, Taghian A, Freeman J, et al. Impact of stromal sensitivity on radiation response of tumors. J Natl Cancer Inst 1993;85:988-93. [Crossref] [PubMed]
- Formenti SC, Demaria S. Combining radiotherapy and cancer immunotherapy: a paradigm shift. J Natl Cancer Inst 2013;105:256-65. [Crossref] [PubMed]
- Harrison D, Phillips JH, Lanier LL. Involvement of a metalloprotease in spontaneous and phorbol ester-induced release of natural killer cell-associated Fc gamma RIII (CD16-II). J Immunol 1991;147:3459-65. [PubMed]
- Grzywacz B, Kataria N, Verneris MR. CD56dimCD16+ NK cells downregulate CD16 following target cell induced activation of matrix metalloproteinases. Leukemia 2007;21:356-9. [Crossref] [PubMed]
- Liu Q, Sun Y, Rihn S, Nolting A, et al. Matrix metallo-protease inhibitors restore impaired NK cell-mediated antibody-dependent cellular cytotoxicity in human immunodeficiency virus type 1 infection. J Virol 2009;83:8705-12. [Crossref] [PubMed]
- Ferris RL, Lenz HJ, Trotta AM, et al. Rationale for combination of therapeutic antibodies targeting tumor cells and immune checkpoint receptors: Harnessing innate and adaptive immunity through IgG1 isotype immune effector stimulation. Cancer Treat Rev 2018;63:48-60. [Crossref] [PubMed]
- Guo Y, Feng X, Jiang Y, et al. PD1 blockade enhances cytotoxicity of in vitro expanded natural killer cells towards myeloma cells. Oncotarget 2016;7:48360-74. [Crossref] [PubMed]
- Benson DM, Bakan CE, Mishra A, et al. The PD-1/PD-L1 axis modulates the natural killer cell versus multiple myeloma effect: a therapeutic target for CT-011, a novel monoclonal anti-PD-1 antibody. Blood 2010;116:2286-94. [Crossref] [PubMed]
- Romagné F, Andre P, Spee P, et al. Preclinical characterization of 1-7F9, a novel human anti-KIR receptor therapeutic antibody that augments natural killer-mediated killing of tumor cells. Blood 2009;114:2667-77. [Crossref] [PubMed]
- Benson DM Jr, Hofmeister CC, Padmanabhan S, et al. A phase 1 trial of the anti-KIR antibody IPH2101 in patients with relapsed/refractory multiple myeloma. Blood 2012;120:4324-33. [Crossref] [PubMed]
- Vey N, Bourhis JH, Boissel NA, et al. A phase 1 trial of the anti-inhibitory KIR mAb IPH2101 for AML in complete remission. Blood 2012;120:4317-23. [Crossref] [PubMed]
- Westin JR, Chu F, Zhang M, et al. Safety and activity of PD1 blockade by pidilizumab in combination with rituximab in patients with relapsed follicular lymphoma: a single group, open-label, phase 2 trial. Lancet Oncol 2014;15:69-77. [Crossref] [PubMed]
- Schaer DA, Murphy JT, Wolchok JD. Modulation of GITR for cancer immunotherapy. Curr Opin Immunol 2012;24:217-24. [Crossref] [PubMed]
- Placke T, Kopp HG, Salih HR. Glucocorticoid-induced TNFR-related (GITR) protein and its ligand in antitumor immunity: functional role and therapeutic modulation. Clin Dev Immunol 2010;2010:239083. [Crossref] [PubMed]
- Baltz KM, Krusch M, Bringmann A, et al. Cancer immunoediting by GITR (glucocorticoid-induced TNF related protein) ligand in humans: NK cell/tumor cell interactions. FASEB J 2007;21:2442-54. [Crossref] [PubMed]
- Hendriks J, Gravestein LA, Tesselaar K, et al. CD27 is required for generation and long-term maintenance of T cell immunity. Nat Immunol 2000;1:433-40. [Crossref] [PubMed]
- Kelly JM, Darcy PK, Markby JL, et al. Induction of tumor-specific T cell memory by NK cell-mediated tumor rejection. Nat Immunol 2002;3:83-90. [Crossref] [PubMed]
- Herrera AF, Jacobsen ED. Ibrutinib for the treatment of mantle cell lymphoma. Clin Cancer Res 2014;20:5365-71. [Crossref] [PubMed]
- Ortaldo JR, Woodhouse C, Morgan AC. Analysis of effector cells in human antibody-dependent cellular cytotoxicity with murine monoclonal antibodies. J. Immunol 1987;138:3566-72. [PubMed]
- Hank JA, Weil-Hillman G, Surfus JE, et al. Addition of interleukin-2 in vitro augments detection of lymphokine-activated killer activity generated in vivo. Cancer Immunol Immunother 1990;31:53-9. [Crossref] [PubMed]
- Schultz KR, Klarnet JP, Peace DJ, et al. Monoclonal antibody therapy of murine lymphoma: enhanced efficacy by concurrent administration of interleukin 2 or lymphokine activated killer cells. Cancer Res 1990;50:5421-5. [PubMed]
- Fujisaki H, Kakuda H, Shimasak N, et al. Expansion of highly cytotoxic human natural killer cells for cancer cell therapy. Cancer Res 2009;69:4010-7. [Crossref] [PubMed]
- Lundqvist A, Yokoyama H, Smith A, et al. Bortezomib treatment and regulatory T-cell depletion enhance the antitumor effects of adoptively infused NK cells. Blood 2009;113:6120-7. [Crossref] [PubMed]
- Bachanova V, Cooley S, Defor TE, et al. Clearance of acute myeloid leukemia by haploidentical natural killer cells is improved using IL-2 diphtheria toxin fusion protein. Blood 2014;123:3855-63. [Crossref] [PubMed]
- Miller JS, Soignier Y, Panoskaltsis-Mortari A, et al. Successful adoptive transfer and in vivo expansion of human haploidentical NK cells in patients with cancer. Blood 2005;105:3051-7. [Crossref] [PubMed]
- Rubnitz JE, Inaba H, Ribeiro RC, et al. NKAML: a pilot study to determine the safety and feasibility of haploidentical natural killer cell transplantation in childhood acute myeloid leukemia. J Clin Oncol 2010;28:955-9. [Crossref] [PubMed]
- Curti A, Ruggeri L, D’Addio A, et al. Successful transfer of alloreactive haploidentical KIR ligand-mismatched natural killer cells after infusion in elderly high risk acute myeloid leukemia patients. Blood 2011;118:3273-9. [Crossref] [PubMed]
- Koepsell SA, Miller JS, McKenna DH Jr. Natural killer cells: a review of manufacturing and clinical utility. Transfusion 2013;53:404-10. [Crossref] [PubMed]
- Klingemann H, Grodman C, Cutler E, et al. Autologous stem cell transplant recipients tolerate haploidentical related-donor natural killer cell-enriched infusions. Transfusion 2013;53:412-8. [Crossref] [PubMed]
- Spiess C, Zhai Q, Carter PJ. Alternative molecular formats and therapeutic applications for bispecific antibodies. Mol Immunol 2015;67:95-106. [Crossref] [PubMed]
- Rothe A, Sasse S, Topp MS, et al. A phase 1 study of the bispecific anti-CD30/CD16A antibody construct AFM13 in patients with relapsed or refractory Hodgkin lymphoma. Blood 2015;125:4024-31. [Crossref] [PubMed]
- Reusch U, Duell J, Ellwanger K, et al. A tetravalent bispecific TandAb (CD19/CD3), AFM11, efficiently recruits T cells for the potent lysis of CD19(+) tumor cells. MAbs 2015;7:584-604. [Crossref] [PubMed]
- Schmohl JU, Felices M, Todhunter D, et al. Tetraspecific scFv construct provides NK cell mediated ADCC and self-sustaining stimuli via insertion of IL-15 as a cross-linker. Oncotarget 2016;7:73830-44. [Crossref] [PubMed]
- Fehniger TA, Shah MH, Turner MJ, et al. Differential cytokine and chemokine gene expression by human NK cells following activation with IL-18 or IL-15 in combination with IL-12: implications for the innate immune response. J Immunol 1999;162:4511-20. [PubMed]
- Carson WE, Giri JG, Lindemann MJ, et al. Interleukin (IL) 15 is a novel cytokine that activates human natural killer cells via components of the IL-2 receptor. J Exp Med 1994;180:1395-403. [Crossref] [PubMed]
- Caligiuri MA, Zmuidzinas A, Manley TJ, et al. Functional consequences of interleukin 2 receptor expression on resting human lymphocytes. Identification of a novel natural killer cell subset with high affinity receptors. J Exp Med 1990;171:1509-26. [Crossref] [PubMed]
- Carrega P, Morandi B, Costa R, et al. Natural killer cells infiltrating human nonsmall-cell lung cancer are enriched in CD56 bright CD16(-) cells and display an impaired capability to kill tumor cells. Cancer 2008;112:863-75. [Crossref] [PubMed]
- Vgenopoulou S, Lazaris AC, Markopoulos C, et al. Immunohistochemical evaluation of immune response in invasive ductal breast cancer of not-otherwise-specified type. Breast 2003;12:172-8. [Crossref] [PubMed]
- Levi I, Amsalem H, Nissan A, et al. Characterization of tumor infiltrating natural killer cell subset. Oncotarget 2015;6:13835-43. [Crossref] [PubMed]
- Barry KC, Hsu J, Broz ML, et al. A natural killer-dendritic cell axis defines checkpoint therapy-responsive tumor microenvironments. Nat Med 2018;24:1178-91. [Crossref] [PubMed]