New treatments of multidrug-resistant Gram-negative ventilator-associated pneumonia
Introduction
Hospital-acquired pneumonia (HAP) remains one of the most important hospital-acquired infections, being associated with significant mortality, morbidity and increase in health expenditures (1,2). Gram-negative pathogens and particularly Enterobacteriaceae, Pseudomonas aeruginosa and Acinetobacter baumannii have progressively become predominant, the latter being more prevalent in patients with ventilator-associated pneumonia (VAP) (3). These pathogens harbor a variety of mechanisms that confer resistance to antibiotics, rendering sometimes infections untreatable (4,5). A dry pipeline for years, led to the belief that we are approaching “the end of antibiotics” (5).
Inappropriate treatment has clearly been associated with increased mortality and healthcare costs (6,7) and MDR pathogens have been associated with inappropriate initial treatment, leading to a vicious cycle (8). In a recent meta-analysis, infections by carbapenem-resistant Klebsiella pneumoniae portended a 42% overall mortality versus 21% for carbapenem-susceptible counterparts (9). The World Health Organization (WHO) has listed carbapenem-resistant Enterobacteriaceae (CRE) among the highest priority pathogens in order to strive antibiotic development as a response to unmet public health threats (10). Following regulatory initiatives and social pressure, the antimicrobial pipeline has produced in the last decade a considerable number of new molecules. The aim of this article is to summarize the newly available or in late stage of development agents (phase 3 trials), expected to treat HAP and VAP caused by multi-drug resistant Gram-negative bacteria (MDR-GNB), focusing on agents with activity against CRE, MDR P. aeruginosa, and MDR A. baumannii.
Basic terms and mechanisms of resistance
After the introduction of the term MDR, a designation implying resistance to at least three different antibiotic classes, the terms extensively-drug-resistant (XDR) and pan-drug-resistant (PDR) have been introduced, implying respectively non-susceptibility in all but two or fewer antimicrobial categories and all agents in all antimicrobial categories (11). Multiple mechanisms of resistance contribute to an MDR, XDR or PDR profile in GNB (12,13). Intrinsic antimicrobial resistance mechanisms may be gradually selected by evolutional pressures; as an example, chromosomal genes that encode efflux pumps which pull antibiotics out of the bacterial cell. Acquired resistance in GNB can emerge through horizontal gene transfers, which are most commonly plasmid-mediated, or spontaneous mutations of existing genes (12). Acquired resistance mechanisms may be enzymatic (i.e., β-lactamases) or non-enzymatic (i.e., alteration of the bacterial membrane composition). Production of β-lactamases stands out as an important mechanism, hydrolysing β-lactam antibiotics which have been for decades the cornerstone of antimicrobial treatment of critically ill patients. The most relevant β-lactamases encountered in MDR pathogens causing HAP and VAP according to Ambler classification are presented in Table 1 (12,13).
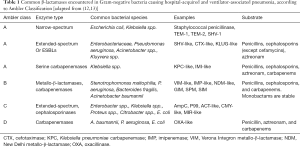
Full table
New antimicrobials
In this section we will present newly launched antibiotics and antimicrobials in late stage of development (having entered in phase 3 clinical trials). Data will be presented from the view point of their potential treatment of VAP or HAP. The main characteristics of the presented antibiotics, are shown in Table 2.
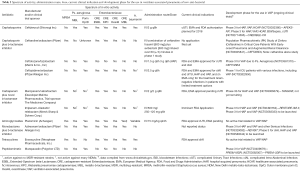
Full table
Combinations of cephalosporins or monobactam with β-lactamase inhibitors
Ceftazidime avibactam (Avycaz®Allergan, Inc for North America only, Zavicefta®, Pfizer)
Ceftazidime–avibactam (CAZ/AVI) combines a well-established third-generation cephalosporin, with a novel non β-lactam β-lactamase inhibitor, avibactam. The latter is active against a variety of β-lactamases, including Ambler Class A (KPC and ESBL type enzymes), Class C (AmpC) and some class D serine enzymes (such as oxacillinase OXA-48). However, it is vulnerable to metallo-β-lactamases (MBL) (Table 2) (14). A potent in vitro activity of CAZ/AVI was shown against CRE and P. aeruginosa excluding isolates producing MBLs (15). Avibactam is minimally active against A. baumannii and vulnerable to OXA-type carbapenemases carried in these species; in addition, it has poor activity against anaerobic Gram-negative bacteria and no activity against Gram-positive cocci (16). Susceptibility of P. aeruginosa to CAZ/AVI is improved relative to ceftazidime alone, but to a lesser extend compared to Enterobacteriaceae (17-19), with susceptibility of isolates resistant to ceftazidime and carbapenems not exceeding 50% in some studies (19-22). Isolates of P. aeruginosa from VAP had CAZ/AVI MIC90 at 16 whereas meropenem-non-susceptible strains had 32 mg/L, compared to 2 mg/L for Enterobacteriaceae and 8 mg/L for non-respiratory Pseudomonas isolates, indicating a more difficult PK/PD target (23,24).
KPC-3 displayed significantly higher MICs of CAZ/AVI, compared to KPC-2 variants, attributed to a compilation of resistance mechanisms, including de novo mutations in the blaKPC-3 gene, production of multiple carbapenemases, reduced porin expression and overexpression of efflux pumps (25,26). Emergence of resistance to CAZ/AVI reported after a relative short course of therapy and particularly after monotherapy, for infections caused by K. pneumoniae, prompts vigilance in clinical practice (4,27). Structural derangements of the omega-loop of KPC-2 were identified, resulting in a new active site of the enzyme, which however can no longer hydrolyze aztreonam or imipenem (28,29). As a consequence, carbapenems and aztreonam could overcome this new mechanism of resistance.
Ceftazidime exerts a time-dependent bactericidal effect, possesses linear pharmacokinetics and is excreted with glomerular filtration (30). Avibactam is eliminated primarily unchanged in the urine (31). Its half-life increases by 2–3 fold, 4 fold, and 12 fold with creatinine clearance (CrCL) values of 30–79 mL/min, <30 mL/min (non-hemodialysis), and <30 mL/min (hemodialysis) respectively (32). The recommended dosage and frequency of administration of CAZ/AVI in patients with normal renal function is 2.5 g every 8 h. Dosage adjustment is required in patients with moderately or severely impaired renal function (33,34). The avibactam pharmacokinetic/pharmacodynamic (PK/PD) target related with efficacy is the percent of time that the free drug levels exceed threshold concentration (%fT>CT). Area under curve (AUC) and maximum plasma concentration (Cmax) seem to play an important role in its pharmacodynamics and particularly against KPC producers (35,36). Early PK/PD studies in humans have demonstrated that both ceftazidime and avibactam penetrate into human epithelial lining fluid (ELF) with concentrations proportionally (25–30%) lower compared to plasma (37). In a recent study, pooled PK/PD data from preclinical pneumonia mice models and phase 1 and phase 2 studies in humans verified plasma levels as a surrogate for lung penetration in patients with HAP and VAP (38). Monte Carlo simulation verified PK/PD target attainment in patients with HAP with the approved CAZ/AVI dose (35,39).
CAZ/AVI has been approved by FDA and EMA for the treatment of complicated Urinary Tract Infections (cUTIs), complicated Intraabdominal Infections (cIAIs), HAP/VAP and for the treatment of infections due to aerobic Gram-negative organisms in adult patients with limited treatment options (EMA only) (33,34). CAZ-AVI with metronidazole in registrational phase 3 trials (RECLAIM 1 and 2) showed non-inferiority to meropenem in the treatment of cIAIs (40). However, patients with moderate renal impairment (creatinine clearance/CrCl >30–50 mL/min) receiving CAZ/AVI had lower response rates compared to those receiving meropenem (40). In the REPRISE registrational phase 3 trial, CAZ/AVI established non-inferiority to doripenem in cUTIs (41). In an open-label trial comparing CAZ/AVI with “best available therapy” in infections caused by ceftazidime-resistant isolates—mostly cUTIs—response rates were 90.9% and 91.2% respectively (42).
In a randomized prospective study, CAZ/AVI was tested versus with meropenem for the treatment of nosocomial pneumonia (NP), including VAP (REPROVE study, Clinical-Trials.gov Identifier NCT01808092) (43). Adults with NP (N=879), were randomized (1:1) to CAZ/AVI or meropenem for 7–14 days. Predominant Gram-negative baseline pathogens were K. pneumoniae (36.6%) and P. aeruginosa (29.6%), with 28.2% being ceftazidime-non-susceptible. Non-inferiority of ceftazidime-avibactam to meropenem was demonstrated in both co-primary analysis populations, with clinical cure rates at test-of-cure 68.8% for CAZ/AVI vs. 73% for meropenem [difference (95% CI), –4.2 (–10.76, 2.46), P=0.007] in the modified intent-to -treat population and 77.4% and 78.1%, respectively [difference (95% CI), –0.7 (–7.86, 6.39), P<0.001] in the clinically evaluable population. Secondary analysis produced similar results, including patients with VAP vs. non-VAP, renal function status (augmented renal clearance, normal renal function/mild impairment or moderate to severe impairment) and concomitant medication with aminoglycosides (43). Based on the results of the RECLAIM1 and 2 studies, the REPROVE protocol was amended to increase the CAZ/AVI dose for patients with moderate/severe renal impairment reflecting PK/PD data and amendments in the Summary of Product Characteristics (SPC) (33,34,40,44). Bacterial persistence with increasing MIC (≥4-fold MIC increase) at end of treatment and/or test of cure was observed in 2/125 (1.6%) patients in the CAZ/AVI group and 11/131 (8.4%) patients in the meropenem group. Overall, CAZ/AVI was well tolerated as in phase 2 and previous phase 3 trials with no similar to the comparators adverse event rates in all published studies (40-43).
In real life, CAZ/AVI is primarily used against CRE infections (not enrolled in registrational trials) displaying clinical response rates between 45% and 76% and relapse rates of 23% (4,26,45,46). A single-center observational study showed higher rates of clinical success and lower mortality with CAZ/AVI compared with other regimens employed for carbapenem-resistant K. pneumoniae (KPC-3 or -2) bacteraemia (47). Development of resistance to CAZ/AVI (MIC ≥16 mg/L) following relatively short courses of therapy generated questions as to whether combination treatment could avert this event (47). A recently published meta-analysis of 4,951 patients deriving from nine Randomized-controlled trials (RCTs) and three observational studies, reported comparable clinical response rate with CAZ-AVI vs. carbapenems and non-inferior bacterial eradication, with no significant difference in mortality rates and adverse events. However, in patients infected by CRE, CAZ/AVI produced improved clinical response (RR =1.61; 95% CI, 1.13–2.29) with reduced mortality (RR =0.29; 95% CI, 0.13–0.63) than comparator regimens. Improved clinical response with CAZ/AVI was also shown in bloodstream infections (48).
Summarizing, CAZ/AVI represents an important addition to our armamentarium against MDR Gram-negative pathogens, being the first marketed treatment option against KPC producers. Treatment as monotherapy is however questionable with existing data, particularly in the context of life-threatening infections. Expert-driven recommendations argue for combination treatment (an aminoglycoside, fosfomycin, tigecycline or colistin and hopefully plazomicin in the near future), based on the antibiogram and the required PK/PD parameters in the infectious focus (4). Its expanded spectrum, covering a considerable proportion of Enterobacteriaceae harboring ESBLs and variable proportions of P. aeruginosa strains, makes CAZ/AVI an appealing treatment option in the empiric treatment of severe infections by MDR pathogens and low probability of metallo-β-lactamases and A. baumannii. Empiric use of CAZ/AVI should be reserved for patients with strong risk factors for infections by KPC- or OXA-48-producers. Monitoring of prescriptions by antibiotic-expert teams is highly advisable. The need of combination treatment should be balanced, considering microbiology data and severity of infection. Figure 1 summarizes potential indications for use of ceftazidime avibactam (4).
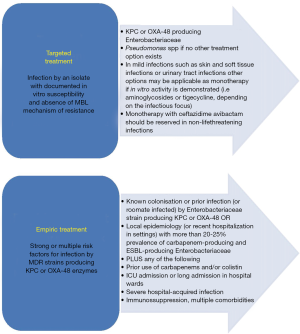
Ceftolozane/Tazobactam (Zerbaxa®, Merck & Co., Inc)
Ceftolozane/tazobactam (C/T) combines a well-established β-lactamase inhibitor with ceftolozane, a novel oxyimino-cephalosporin structurally related to ceftazidime. The latter has higher affinity than ceftazidime for the penicillin-binding proteins PBP1b, PBP1c, PBP2 and PBP3, which are essential in Pseudomonas aeruginosa (49,50). Ceftolozane remains active in AmpC overproduction, overexpression of efflux pumps, and loss of the OprD outer membrane porin, which are common in P. aeruginosa (51). The development of mutational resistance to C/T in P. aeruginosa requires several mutations leading to AmpC overexpression and structural modification (52).
Tazobactam provides stability against most extended-spectrum β-lactamases (ESBLs) which hydrolyze ceftolozane alone (49), but the combination of C/T is vulnerable in the presence of carbapenemases (e.g., KPC, VIM, NDM, GES), except for OXA-48 (51). Large surveillance studies show that C/T has the most potent in vitro activity among β-lactams against P. aeruginosa and excellent activity against Enterobacteriaceae. Haemophilus influenzae and Moraxella catarrhalis are susceptible to C/T whereas some of the Burkholderia spp. and Stenotrophomonas maltophilia isolates have relatively low MICs to C/T. A. baumannii displays no substantial susceptibility (51-53). Among Gram-positive bacteria, C/T shows considerable in vitro activity against Streptococcus viridans and β-hemolytic streptococci (Streptococcus pyogenes and S. agalactiae), but has uncertain activity against S. pneumoniae. While it is not active against staphylococci and enterococci and has minimal anaerobic activity (49,51,53). Time-kill studies have also shown a greater bactericidal activity against P. aeruginosa compared with other cephalosporins whereas carbapenems appear to be more potent against Enterobacteriaceae (54). Pharmacodynamic studies have shown that the time of free drug concentration above the MIC required to induce the same magnitude of bactericidal activity or to prevent the emergence of resistance is lower for C/T compared with other cephalosporins against Gram-negative bacteria (55).
C/T is currently approved for clinical use in the United States and Europe for the indications of cIAIs and cUTIs, based on phase 3, non-inferiority clinical trials that compared C/T in combination with metronidazole versus meropenem and C/T versus levofloxacin, respectively (56,57). Another phase 3 clinical trial (ASPECT-NP) comparing C/T with meropenem in patients with HAP requiring mechanical ventilation or VAP has been completed in June 2018. Up to this writing, the results have not been announced.
The dosage of C/T used in the nosocomial pneumonia trial was double (3 g every 8 h for normal renal function) the dosage used in the previous trials (1.5 g every 8 h). This was based on a phase 1 pharmacokinetic study in healthy volunteers that showed the area under the concentration (AUC)—time curve for ceftolozane in the ELF was approximately half than that in plasma (12,58). A population pharmacokinetic model verified that doubling of the ceftolozane dosage is required for a higher than 90% probability of pharmacodynamic target attainment for 1-log killing of pathogens with an MIC ≤8 mg/L (13,59). Of note, the breakpoint of susceptibility for C/T against P. aeruginosa is 4 mg/L according to both CLSI and EUCAST. The 3 g every 8 h dosage for C/T has been further evaluated in healthy volunteers for a duration of 10 days. No safety concerns were raised (60). A study using an experimental rabbit P. aeruginosa pneumonia model showed that C/T was equally effective to ceftazidime, piperacillin/tazobactam, and imipenem, if a dosage of C/T of 1.5 g every 8 h used, but more effective than the comparators with doubling of the dosage (61).
Pending the announcement of the results of the ASPECT-NP trial, data on the clinical effectiveness of C/T for respiratory infections and VAP in particular, are currently based on small case series and case-reports relating to off-label use. Variable dosage regimens were used and C/T was often administered in combination with other antibiotics. The published relevant case-series up to this writing overall show that C/T resulted in clinical success in 61.4% of patients with P. aeruginosa pneumonia (62-69). Based on the available data, failure of C/T therapy in serious P. aeruginosa infections is associated with an MIC higher than 4 mg/L (70) and the use of the lower dosage regimen (71). Development of C/T resistance during therapy of P. aeruginosa infections has also been described (64,67,70).
Regarding the effectiveness of C/T for infections caused by ESBL-producing Enterobacteriaceae, in a secondary analysis of the cases included in the clinical trials of cIAIs and cUTIs C/T achieved clinical cure rates (97.4%) among patients with E. coli or K. pneumoniae infections compared with 82.6% for levofloxacin and 88.5% for meropenem (72).
Both ceftolozane and tazobactam are excreted mainly through kidneys. Substantial reductions in the dosage of C/T should be made with decreasing creatinine clearance according the approved label of the drug. Monte Carlo simulations have shown the currently approved dosage of C/T for various degrees of renal impairment have a probability of pharmacodynamic target attainment more than 90% for an MIC up to 8 mg/L (73). This figure decreases to an MIC of 4 mg/L for patients with normal renal function or augmented renal clearance (73). Moderate renal impairment has not shown to affect the outcomes of C/T therapy in the clinical trials of cIAIs and cUTIs (71). Higher than the currently approved dosage may be required though to treat serious respiratory infections due to MDR P. aeruginosa (74). Case-reports have proposed a dosage up to 1.5 g three times daily for continuous venovenous hemofiltration (75) and of 1.5–3 g three times daily for continuous venovenous hemodiafiltration (76,77). The labelled duration of the infusion is 1 hour. However, a 4- to 5-hour extended infusion regimen has been shown to maximise the probability of pharmacodynamic target attainment for infections with pathogens with elevated MICs, according to Monte Carlo simulations (75,78).
As part of combination empiric regimens, C/T can provide adequate coverage of P. aeruginosa isolates in settings where carbapenemase-production is low (79). In conclusion, C/T appears to be a valuable addition to the antimicrobial armamentarium for the treatment of VAP. The main place in therapy of C/T in this setting is for empirical or targeted coverage of infections caused by P. aeruginosa. It has been recommended for this indication in a reiteration of the current international guidelines for ventilator-associated pneumonia (80). C/T may be the treatment of choice for P. aeruginosa strains with resistance to carbapenems attributed to mechanisms other than the production of carbapenemases. Against ESBL-producing Enterobacteriaceae C/T may be considered as a carbapenem-sparing option. The results of a recently completed phase 3 clinical trial are awaited to establish the role of C/T in HAP/VAP.
Aztreonam/avibactam (Pfizer)
Aztreonam, a well-established monobactam antibiotic since 1986, with clinically useful activity against aerobic MBL-producing bacteria, is currently being re-examined due to its potential to treat MDR Gram negative bacteria. Its activity in current clinical practice against MBL-producers is hampered by the frequent co-production of additional β-lactamases including ESBLs, AmpC enzymes, and serine carbapenemases on the same isolate, which hydrolyse and inactivate aztreonam (81-84). Its combination with avibactam expands the in vitro spectrum to include strains producing Ambler class A and class C, and some class D β-lactamases, including ESBLs, and serine carbapenemases (KPC and OXA-48-type) (85). The combination of aztreonam-avibactam achieved a significant reduction in MIC values for Enterobacteriaceae isolates producing β-lactamases, ESBL and AmpC enzymes, including OXA-48 (86). The addition of avibactam to aztreonam failed to restore susceptibility in MDR strains of P. aeruginosa and A. baumannii, suggesting that resistance to aztreonam in these species is primarily driven by other mechanisms (82,83,87-89).
At present, a phase 3 clinical trial is recruiting patients with serious infections due to Gram- negative bacteria and limited or no treatment options, in order to determine efficacy, safety and tolerability of aztreonam/avibactam ± metronidazole versus meropenem ± colimycin (NCT03329092). In addition, a randomized phase 3 clinical trial is estimated to start in September 2018, with aim to evaluate the clinical use of aztreonam/avibactam compared to best available therapy (BAT) in hospitalized patients with infections due to metallo-β-lactamase (MBL)- producing Gram-negative bacteria, including HAP and VAP.
In this regard, aztreonam/avibactam represents one of the most promising treatment options for serious infections, including HAP and VAP, caused by resistant bacteria, and particularly those expressing metallo-b-lactamases, for which currently very few agents are coming from the pipeline.
Ceftaroline/Avibactam (ZinforoTM, Pfizer)
Ceftaroline is a novel, fifth generation cephalosporin, which has been approved by the FDA and EMA for acute bacterial skin and skin structure infections caused by MRSA and community-acquired pneumonia (CAP) including that caused by S. pneumoniae (90-92). This novel cephalosporin possesses both Gram-positive and Gram-negative coverage, including methicillin susceptible Staphylococcus aureus (MSSA), MRSA and Enterobacteriaceae, although demonstrates limited anaerobic activity and no activity against abdominal anaerobes as well as ESBL and AmpC expressing species, A. baumannii and P. aeruginosa (90,93). The prodrug, ceftaroline fosamil, is converted to active ceftaroline in the plasma, which binds to the plasma proteins in approximately 20% and it is mainly excreted by the kidneys. The recommended dose is 600 mg iv over one-hour infusion twice daily and adjustment for moderate and severe renal impairment is needed (36,90,94).
Although the ceftaroline/avibactam combination is not yet thoroughly tested, the addition of avibactam seems promising with view to overcoming the above-mentioned limitations in terms of spectrum and extending activity against many multidrug-resistant (MDR) pathogens, while preserving its potent anti-staphylococcal spectrum (including MRSA strains) (95). This combination could prove to be extremely useful as a single-agent empirical coverage for Gram-positive/Gram-negative pathogens with multiple mechanisms of resistance. Although further pharmacokinetic studies are required in critically ill and mechanically ventilated populations, this antibiotic, due to its relative antimicrobial spectrum covering both Gram-negative and Gram-positive strains, seems quite promising coupled with avibactam in VAP/HAP.
New cephalosporins
Cefiderocol (Shionogi Inc)
Cefiderocol, formerly S-649266, is a siderophore cephalosporin for parenteral use with a novel mechanism of bacterial cell entry. Siderophores are small, high-affinity iron-chelating compounds that are produced by a variety of bacteria and fungi (96). Microorganisms recognize only certain siderophores, therefore, such conjugates exhibit a selective antimicrobial activity (97). For years, use of the iron transport abilities of siderophores to carry antimicrobials into cells has been a great challenge (98). Cefiderocol possesses a catechol moiety functioning as a siderophore to form a chelating complex with iron; it utilizes the bacterial iron transport system to penetrate susceptible microorganisms (99).
Cefiderocol demonstrated in vitro activity against ESBL-producers and CRE. Organisms producing Ambler Class A, D and B enzymes (metallo-β-lactamases) including NDM-1 enzymes were susceptible to cefiderocol (100). In vitro study of a large international collection of clinical strains showed for cefiderocol MIC90s of 0.5 and 1 µg/mL respectively, including a variety of MDR-bacterial pathogens such as P. aeruginosa, A. baumannii, S. maltophilia and Burkholderia cepacia (101). In an in vitro study of cefiderocol against 282 meropenem-nonsusceptible isolates of P. aeruginosa, A. baumannii, K. pneumoniae, and Providencia stuartii collected from Greek hospitals, cefiderocol displayed the lowest MIC values among 10 comparators (102). In the neutropenic murine thigh infection model, cefiderocol achieved bacterial stasis 85%) P. aeruginosa isolates, 88% A. baumannii isolates, and 77% of Enterobacteriaceae isolates with cefiderocol MIC ≤4 µg/mL. Furthermore, it was efficacious against two tested isolates of NDM-producing K. pneumoniae (103).
Cefiderocol is currently in phase 3 of clinical development. Data from one Phase 3 trial known as APEKS-cUTI has been reported by Shionogi (104). Cefiderocol in hospitalized patients at risk for MDR cUTI, demonstrated non-inferiority over treatment with imipenem/cilastatin. Cefiderocol non-inferiority was consistent across patient clinical and microbiologic subgroups demonstrating a treatment difference of 15–20% vs. comparator arm, while being generally well tolerated, with no unexpected safety concerns identified (104).
Two phase 3 clinical trials are currently ongoing for various infections, including VAP. The APEKS-NP trial is a clinical study on adults with nosocomial pneumonia [HAP, VAP and healthcare-associated pneumonia (HCAP)] caused by Gram-negative pathogens versus meropenem (NCT03032380). A second multicenter Phase 3 trial comparing cefiderocol to best available therapy in infections caused by carbapenem-resistant pathogens (CREDIBLE) is also underway (NCT02714595).
Cefiderocol stands out as one of the most promising new antimicrobials against MDR Gram negative pathogens, with possible indication nosocomial pneumonia. Some of the most appealing characteristics of this molecule are the unique mechanism of entry into the bacterial cell and the expanded antimicrobial spectrum including metallo-β-lactamase producers and A. baumannii, against which very few forthcoming therapeutic options will be active.
Combinations of carbapenems with novel β-lactamase inhibitors
Meropenem/vaborbactam (VabomereTM, Melinta Therapeutics)
This combination includes an existing β-lactam antibiotic (meropenem) with a novel inhibitor (vaborbactam) which is a cyclic boronate non-β-lactam β-lactamase inhibitor. Vaborbactam (formerly RPX7009) possesses high affinity for serine proteases therefore inhibits KPC enzymes, as well as other Ambler class A and C enzymes but not members of Amber class B (metallo-β-lactamases/MBLs). It has not antibacterial activity itself (105). In a concentration of 8 µg/mL, vaborbactam augments in vitro the activity of meropenem against carbapenemase-producing Enterobacteriaceae isolates by least 64-fold but has no effect on meropenem non-susceptible A. baumannii spp producing OXA-type carbapenemases or on P. aeruginosa (106,107). The half-life of vaborbactam is 1.23 h and the steady state volume of distribution 21.0 L mimicking PK properties of β-lactams (108). The used combination dose of meropenem–vaborbactam in clinical studies is 2 g—2 g iv every 8 h. With this dose the achieved concentrations in plasma and ELF display a similar time-course and magnitude, with a penetration of 65% and 79% for meropenem and vaborbactam respectively (108).
In a phase 3, multi-center, 1:1 randomized, double-blind study comparing meropenem-vaborbactam to piperacillin-tazobactam in the treatment of cUTI in adults (TANGO-1) non-inferiority was achieved for the FDA and the EMA endpoints (109). According to the TANGO-1 study data, the US FDA approved recently meropenem–vaborbactam for the treatment of cUTIs including pyelonephritis (110). The TANGO-2 study (ClinicalTrials.gov Identifier: NCT02168946) was a randomized 2:1 study comparing meropenem-vaborbactam to best-available therapy (BAT) for the treatment of a variety of infections including cUTI, hospital-acquired and ventilator-associated bacterial pneumonia (HABP/VABP), cIAI and bacteremia by suspected carbapenem-resistant Enterobacteriaceae (111). TANGO-2 recruitment was prematurely terminated following interim analysis by the independent Data and Safety Monitoring Board (DSMB) based on 72 patients. Preliminary results showed statistically significant efficacy over BAT (57.1% vs. 33.3%), decreased nephrotoxicity and fewer treatment-related adverse events across all groups (111,112). In the cohort of patients with HABP/VABP and bacteremia, a lower day-28 all-cause mortality rate was demonstrated versus BAT (25.0% vs. 44.4%, respectively) (112). TANGO-3 (ClinicalTrials.gov identifier: NCT03006679, not yet recruiting at the writing of this review) will compare meropenem-vaborbactam vs. piperacillin-tazobactam in patients with HABP/VABP. Furthermore, a new phase 1 trial is currently underway in pediatric patients (ClinicalTrials.gov Identifier: NCT02687906).
Imipenem-relebactam (Merck Sharp & Dohme Corp.)
This combination includes an existing β-lactam antibiotic, imipenem-cilastatin, with the novel non β-lactam β-lactamase inhibitor relebactam (formerly MK-7655) which is a bridged bicyclic urea molecule (113). Relebactam, is a serine-based molecule with a diazabicyclooctane core and a piperidine ring has a mechanism of action similar to that of avibactam, resulting in a potent inhibition of both class A and C β-lactamases including KPC enzymes but is not active against metallo-beta-lactamases (MBLs) and class D carbapenemases (113). The combination of relebactam with imipenem broadens the spectrum to include imipenem-resistant Enterobacteriaceae and P. aeruginosa strains without however providing any benefit against A. baumannii. At a concentration of 4 mg/L it relebactam reduced the imipenem MIC for KPC-producing Enterobacteriaceae from 16–64 mg/L to 0.12–1 mg/L, but is not clear if there is an activity against OXA-48 producing isolates (114,115).
The PK index correlating to efficacy of relebactam is AUC while the optimal dosing has not been determined yet. However, phase 1 studies suggested that relebactam doses at or above 125 mg every 6 h achieve an adequate PK/PD target (116). Imipenem and relebactam are associated with a promising intrapulmonary penetration (ELF 53% of plasma) reflecting a good therapeutic profile against nosocomial respiratory infections (117). Clinical but not published yet data from phase 1 trials showed a favorable tolerability and the most common observed adverse events (incidence >5%) are diarrhea, nausea, and vomiting (117). In phase 2 clinical studies in patients with cIAI and cUTI the administration of imipenem (500 mg every 6 h) with relebactam in different dosages (125 and 250 mg) vs. imipenem-cilastatin alone was associated with high clinical response (>97%) in both infections and similar rates of microbiological response, confirming non-inferiority of both imipenem/cilastatin + relebactam doses to imipenem/cilastatin alone (118). All 23 patients with imipenem non-susceptible pathogens had favorable microbiological responses (100% in each group). A phase 3, randomized, double-blind, controlled trial (including HAP and VAP) in adult patients with infections due to imipenem-resistant strains (imipenem-relebactam versus colistimethate sodium plus imipenem/cilastatin was used) has been completed without announced results yet (ClinicalTrials.gov Identifier: NCT02452047). Currently a phase 3 study is ongoing, aiming to assess the non-inferiority of imipenem-relebactam against piperacillin/tazobactam in patients with HAP or VAP (ClinicalTrials.gov Identifier: NCT02493764).
New tetracyclines
Eravacycline (Xerava™, Tetraphase Pharmaceuticals, Inc.)
Eravacycline is a novel, synthetic fluorocycline, with in vitro broad-spectrum activity against aerobic bacteria, both Gram-positive cocci and Gram-negative bacilli, including difficult-to-treat resistant pathogens such as methicillin-resistant Staphylococcus aureus (MRSA), Vancomycin-resistant Enterococcus (VRE), GNB producing ESBL and KPC enzymes and MDR A. baumannii except for P. aeruginosa (119,120). Eravacycline, compared to commonly used antibiotics showed a potent in vitro activity against the majority of MDR pathogens with an MIC90 of 0.5 mg/L against E. coli isolates that were non-susceptible to third-generation cephalosporins and fluoroquinolones (120,121). In a PK/PD study of eravacycline in a murine model of infection by Enterobacteriaceae, fAUC/MIC index was confirmed, as the parameter which is associated with efficacy of the tetracyclines class (122). Eravacycline, has similar structure, mechanism of action and antibacterial spectrum with tigecycline, retaining activity against most tetracycline resistance mechanisms (efflux pumps and ribosomal protection proteins) (123). However, eravacycline is more potent in vitro, 2- to 4-fold versus Gram-positive cocci and 2- to 8-fold versus Gram-negative bacilli (119,124). In addition, eravacycline has excellent oral bioavailability, high metabolic stability and low potential for drug to drug interactions while its activity in biofilm cultures appeared more effective than tigecycline (119). Against clinical isolates of A. baumannii, eravacycline displayed greater susceptibility than tigecycline and demonstrated improved in vitro activity against CRE isolates, higher serum levels, and better tolerability (120,125). PK/PD studies support once-daily administration. The availability of both oral and intravenous formulations is an advantage, whereas good lung penetration and activity on bacterial biofilm provide promising features for the treatment of ICU infections and particularly VAP and VAT (119,125,126). PK/PD studies in murine thigh infection have shown maximum plasma concentration (Cmax) values of 0.34 to 2.58 mg/L, area under the concentration-time curve (AUC) from time zero to infinity (AUC0–∞) values of 2.44 to 57.6 mg·h/L, and elimination half-lives of 3.9 to 17.6 h (127).
The clinical efficacy of eravacycline was demonstrated in community-acquired cIAIs and cUTIs (128-130). At the time of the writing of this review, several studies have been completed, including a phase 2 clinical trial for the treatment of cIAIs and four phase 3 clinical trials known as IGNITE trials (Investigating Gram-negative Infections Treated with Eravacycline). IGNITE1 and IGNITE4 were randomized, double-blind, double-dummy, multi-center studies assessing the efficacy and safety of intravenous (IV) eravacycline (1 mg/kg iv q12h) compared to ertapenem and meropenem, respectively, in patients with cIAI. Both IGNITE1 (541 enrolled patients) and IGNITE 4 (500 enrolled patients) met the primary endpoints of clinical cure (128,129). In a pooled analysis of IGNITE1 and IGNITE 4 studies announced as congress presentation, favorable clinical and microbiological responses were observed for eravacycline against Enterobacteriaceae and Acinetobacter baumannii, including those demonstrating resistant phenotypes and genotypes (130). In patients with cIAIs caused by A. baumannii, of which most strains were MDR, eravacycline-treated patients achieved a 100% clinical and microbiological response rate. Both IGNITE 2 and 3 phase 3 randomized, multi-center, double-blind, clinical trials evaluating the efficacy and safety of once-daily iv eravacycline (1.5 mg/kg every 24 h) compared to levofloxacin (750 mg every 24 h) and ertapenem (1 g every 24 h) respectively for the treatment of cUTI failed to meet non-inferiority criteria. Full data analysis is not yet available however the selected lower dose compared to that administered in the cIAI trials may have contributed to this. The manufacturing company applied in US and European regulatory agencies only for the cIAI indication. Approval was granted at the time of writing for US market (131).
A phase 1 study in healthy volunteers showed an excellent penetration profile in lung tissue (concentrations greater than plasma by six-fold in the ELF and 50-fold in the alveolar macrophages), highlighting eravacycline as a perfect candidate for treatment against MDR bacteria causing pneumonia in critically ill patients (126). Summarizing, eravacycline allows good coverage of the vast majority of difficult-to-treat bacteria except P. aeruginosa. It is the only antibiotic under development with potential activity against MDR A. baumannii and it may play a role in reducing the use of cephalosporins and carbapenems. However, considering the discordance of pharmacokinetics encountered in healthy volunteers compared to critically ill patients with tigecycline, and the surprising failure of eravacycline pivotal trials in cUTIs, further PK/PD studies of eravacycline in critically ill patients with HAP/VAP are warranted in order to establish adequate lung tissue penetration in mechanically ventilated patients and inflamed lung tissue (132,133).
New aminoglycosides
Plazomicin (Zemdri™, Achaogen)
Plazomicin is a next-generation semisynthetic aminoglycoside which acts by inhibiting bacterial protein synthesis and was developed to target MDR Enterobacteriaceae and specifically strains that express aminoglycoside-modifying enzymes (134,135). However, before its entry into clinical practice, ribosomal ribonucleic acid (rRNA) methyltransferase enzymes were already identified in Enterobacteriaceae, P. aeruginosa, and A. baumannii, conferring broad-spectrum resistance to all aminoglycosides, including plazomicin (136-139). Plazomicin exhibits dose-dependent bactericidal activity and is active in vitro against Gram-negative bacteria, such as Enterobacteriaceae, including CRE, ESBL and MDR isolates resistant to currently available aminoglycosides (136,138,140). In vitro activity with MIC90 ≤2 mg/L or 4 mg/L was demonstrated against KPC-producers exhibiting resistance to other aminoglycosides (137,140). In vitro activity of plazomicin against nonfermenters is less potent compared to Enterobacteriaceae. MDR P. aeruginosa had plazomicin MICs similar to those of other aminoglycosides (138). However, OXA-producing A. baumannii resistant to other aminoglycosides may be susceptible to plazomicin (139). Tested against 82 isolates of CRE, plazomicin showed 79% in vitro susceptibility with MICs ≤2 mg/L, including isolates producing metallo-β-lactamases type VIM or IMP but not NDM-1. Ribosomal methyltransferases are commonly co-produced by NDM-1-positive Enterobacteriaceae, therefore, plazomicin is not anticipated to be active against these isolates (136). In addition, plazomicin has shown potent activity against Gram-positive bacteria such as MRSA, including isolates with resistance to previous generation aminoglycosides (138).
The PK/PD properties of plazomicin revealed a linear and dose-proportional profile, with an elimination half time (t1/2) estimated at 4 h and lack of accumulation supportive of once daily therapy, whereas the dosage of plazomicin 15 mg/kg should be considered as therapeutic (141,142). With 15 mg/kg once daily dose, steady state volume of distribution was 0.19±0.02 L/kg, clearance 1.04±0.11 mL/min/kg, urinary excretion 89%±6% of the dosage after a single dose, Cmax at 144±45 mg/L for the first dose and 142±32 mg/L following repeated administration, whereas the trough levels were 0.21±0.06 mg/L after multiple dose.
Plazomicin showed promising clinical characteristics and safety in the phase 2 trial (ClinicalTrials.gov under identifier NCT01096849) (143). EPIC (Evaluating Plazomicin in cUTI), was the first phase 3 registration trial (NCT02486627), in which plazomicin met the non-inferiority endpoint when compared with meropenem (144). The second phase 3, CARE descriptive trial (ClinicalTrials.gov Identifier NCT01970371), evaluated the efficacy and safety of plazomicin versus colistin as part of a definitive combination regimen for the treatment of serious infections due to CRE excluding NDM-producers. CARE enrolled 39 patients with a variety of infections including 29 bloodstream infections (BSI), and 8 HAP/VAP. Patients received plazomicin 15 mg/kg every 24 h or colistin, in both arms combined with a second agent (tigecycline or meropenem). The plazomicin group was associated with a lower rate of mortality (23.5% vs. 50.0%, respectively; 90% confidence interval: 0.7–51.2%). Serious disease-related complications were observed in 5.6% vs. 19% in plazomicin and colistin groups respectively (145,146). Plazomicin exhibited a favorable safety profile including renal function and no reported ototoxicity.
Plazomicin was FDA approved in June 2018 for patients 18 years of age or older for the intravenous treatment of cUTIs, including pyelonephritis caused by the following susceptible microorganism(s): Escherichia coli, Klebsiella pneumoniae, Proteus mirabilis, and Enterobacter cloacae. In the drug’s SPC it is advised to be reserved for use in cUTI patients who have limited or no alternative treatment options, whereas potential nephrotoxicity, ototoxicity, neuromuscular blockade and foetal harm have been included in a boxed warning (147,148).
In summary, plazomicin is characterized by a favorable lung penetration, minor toxicity issues and a challenging antimicrobial spectrum including MDR Gram-negatives (mainly Enterobacteriaceae but variably P. aeruginosa and A. baumannii) and MRSA. It could be an important treatment option in patients with VAP, particularly as part of empiric regimens and also as combination treatment where monotherapy is not advised. As an aminoglycoside, its use as monotherapy particularly in VAP requires further strong clinical evidence.
Outer membrane protein targeting antibiotics (OMPTA)
Murepavadin (Polyphor Ltd)
Murepavadin (POL7080) is a 14-amino-acid macrocyclic peptide and is the first in-class pathogen-specific peptidomimetic antimicrobial. OMPTA possess a novel, non-lytic mechanism of action against Gram-negative bacteria. Murepavadin is Pseudomonas-specific and acts by binding to the lipopolysaccharide transport protein D (LptD), involved in lipopolysaccharide biogenesis (149). Disruption of the lipopolysaccharide transport causes alterations in the outer membrane of the bacterial cell and, ultimately death. Due to its selective affinity for Pseudomonas spp and the targeted mechanism of action, selection of resistance to other bacterial species or disruption of the colonic flora is not anticipated with murepavadin (150). In vitro, murepavadin exhibits potent antimicrobial activity against P. aeruginosa and good activity against some other tested Pseudomonas species but is not active against other Gram-negative species (i.e., S. maltophilia, B. cepacia, Enterobacteriaceae, A. baumannii) or Gram-positive cocci (151). In large in vitro studies of murepavadin and comparators, carbapenemase-producing, colistin-resistant, XDR and PDR isolates of P. aeruginosa were susceptible to murepavadine and exhibited an MIC90 of 0.12 mg/L, similar to non-MDR isolates (152,153).
Murepavadin has shown to be highly effective in several animal infection models and particularly potent in neutropenic lung infection models against XDR isolates where polymyxins were the only treatment option (151). Murepavadin has completed 6 phase 1 (POL7080-001, NP29332, NP29333, NP29334, POL7080-005 and POL7080-009 and two phase 2 trials (POL7080-002 and POL7080-003) (151,154), assessing safety and pharmacokinetics. PK studies after a single dose of murepavadin, showed a geometric mean half-life of 2.52 to 5.30 h, a total clearance of 80.1 to 114 mL/h/kg, and a volume of distribution of 415 to 724 mL/kg, consistent across dose levels, with linear and dose-proportional pharmacokinetics (155,156). Lung penetration was assessed in a multiple dose study (ClinicalTrials.gov identifier: NCT02165293); ELF concentrations measured in this and subsequent studies were comparable with free plasma concentration with a third compartment effect (157-159).
Murepavadin was well tolerated, adverse events were transient and generally mild (155). Nephrotoxicity, might be a concern in clinical practice, since systemic exposure to murepavadin (both as AUC and Cmax) increased in subjects with renal function impairment compared to subjects with normal renal function; therefore dose adjustments according to CrCl will be required (156). A phase 2 open-label study in VABP (ClinicalTrials.gov identifier: NCT02096328) enrolled 25 VABP patients who received murepavadin, twelve of which had a microbiologically documented infection due to P. aeruginosa (in nine cases MDR or XDR isolate). Clinical cure rate was 83% at test of cure among patients with confirmed P. aeruginosa with a reported 28-day all-cause mortality rate of 8%, far below the 20–40% expected mortality rate (160). No development of treatment-emergent resistance to murepavadin was detected. Small sample size calls for caution in the interpretation of this encouraging however preliminary data in humans with VABP. Currently a phase 3 clinical study of murepavadin in HABP/VABP is underway.
In conclusion, murepavadin is a promising “concept approach to pathogen-specific treatment”. Its broad in vitro spectrum against XDR isolates of Pseudomonas spp, offers therapeutic option against this notorious pathogen and could enhance initial appropriate treatment regimens in patients with strong risk factors for infections by this pathogen. The drug would also be ideal for de-escalation of initial treatment, once identification and susceptibility are available. Due to its unique mechanism of action, no cross-resistance with other antibiotics and no ecologic damage in bacterial flora seem to be additional advantages.
Conclusions
As management of VAP requires adequate and timely antibiotic administration, global emergence of antimicrobial resistance poses serious challenges over our ability to maintain this axiom. Among recently developed antimicrobials presented herein, it is obvious that we will have good therapeutic options against Enterobacteriaceae excluding those producing metallo-β-lactamases, against which only cefiderocol and aztreonam/avibactam are expected to be active. As far as carbapenem non-susceptible P. aeruginosa is concerned, ceftolozane/tazobactam and to a lesser extend ceftazidime/avibactam may cover a proportion of current medical needs, but there still remain a considerable proportion of strains which harbor other resistance mechanisms. Murepavadin and cefiderocol hold promise against this particularly notorious pathogen. On the other hand, A. baummannii remains a treatment-challenge. Eravacycline, cefiderocol and maybe plazomicin seem to be the most promising agents against this difficult-to treat pathogen, but we have still a long road ahead, to see their position in clinical practice and particularly in VAP.
In summary, despite persisting and increasing unmet medical needs, several newly approved and forthcoming agents hold promise for the treatment of VAP and hopefully will enrich our antimicrobial arsenal in the next few years. Some very optimists believe that we will be able to delete the term PDR from our medical dictionary. Recent experience with new antibiotics however, calls for studies assessing the efficacy of these novel agents in real-life, particularly considering their potential of selecting resistance. A crucial question is the potential of these agents to stand alone as monotherapy, which is questionable in an empiric regimen given their “precise” spectrum, whereas in the context of targeted therapy more real-life data are required. Companion drugs have to be carefully evaluated in terms of potential adverse events and ecologic consequences; finally, the economic component of combinations must be carefully balanced. The introduction of rapid microbiological methods of identification albeit costly and labour-demanding at this timepoint, could provide invaluable support in the rational use of new antimicrobials. Newly approved and forthcoming antibiotics with activity against MDR, XDR and currently PDR Gram negative pathogens have to be protected against unjustified use, otherwise, the joyful dawn will end and the PDR nightmare will be a global reality.
Acknowledgements
None.
Footnote
Conflicts of Interest: The authors have no conflicts of interest to declare.
References
- Magill SS, Edwards JR, Bamberg W, et al. Multistate point-prevalence survey of health care-associated infections. N Engl J Med 2014;370:1198-208. [Crossref] [PubMed]
- Bouadma L, Sonneville R, Garrouste-Orgeas M, et al. Ventilator-associated events: prevalence, outcome, and relationship with ventilator-associated pneumonia. Crit Care Med 2015;43:1798-806. [Crossref] [PubMed]
- Centers for Disease Control and Prevention. Antibiotic resistance threats in the United States. 2013. Available online: http://www.cdc.gov/drugresistance/threat-report-2013/pdf/ar-threats-2013-508.pdf, Accessed 23 July 2018.
- Bassetti M, Giacobbe DR, Giamarellou H, et al. Management of KPC producing Klebsiella pneumoniae infections. Clin Microbiol Infect 2018;24:133-44. [Crossref] [PubMed]
- Luna CM, Aruj P, Niederman MS, et al. Appropriateness and delay to initiate therapy in ventilator-associated pneumonia. Eur Respir J 2006;27:158-64. [Crossref] [PubMed]
- Zilberberg MD, Nathanson BH, Sulham K, et al. Carbapenem resistance, inappropriate empiric treatment and outcomes among patients hospitalized with Enterobacteriaceae urinary tract infection, pneumonia and sepsis. BMC Infect Dis 2017;17:279. [Crossref] [PubMed]
- Vardakas KZ, Rafailidis PI, Konstantelias AA, et al. Predictors of mortality in patients with infections due to multidrug resistant Gram-negative bacteria: the study, the patient, the bug or the drug? J Infect 2013;66:401-14. [Crossref] [PubMed]
- Xu L, Sun X, Ma X. Systematic review and meta-analysis of mortality of patients infected with carbapenem-resistant Klebsiella pneumoniae. Ann Clin Microbiol Antimicrob 2017;16:18. [Crossref] [PubMed]
- Prioritization of pathogens to guide discovery, research and development of new antibiotics for drug-resistant bacterial infections, including tuberculosis. Geneva: World Health Organization; 2017 (WHO/EMP/IAU/2017.12).
- Cerceo E, Deitelzweig SB, Sherman BM, et al. Multidrug-resistant Gram-negative bacterial infections in the hospital setting: Overview, implications for clinical practice, and emerging treatment options. Microb Drug Resist 2016;22:412-31. [Crossref] [PubMed]
- Magiorakos AP, Srinivasan A, Carey RB, et al. Multidrug-resistant, extensively drug-resistant and pandrug-resistant bacteria: an international expert proposal for interim standard definitions for acquired resistance. Clin Microbiol Infect 2012;18:268-81. [Crossref] [PubMed]
- Ruppé É, Woerther PL, Barbier F. Mechanisms of antimicrobial resistance in Gram negative bacilli. Ann Intensive Care 2015;5:61. [Crossref] [PubMed]
- Miriagou V, Cornaglia G, Edelstein M, et al. Acquired carbapenemases in Gram-negative bacterial pathogens: detection and surveillance issues. Clin Microbiol Infect 2010;16:112-22. [Crossref] [PubMed]
- Keepers TR, Gomez M, Celeri C, et al. Bactericidal activity, absence of serum effect, and time-kill kinetics of ceftazidime-avibactam against beta-lactamase producing Enterobacteriaceae and Pseudomonas aeruginosa. Antimicrob Agents Chemother 2014;58:5297-305. [Crossref] [PubMed]
- Citron DM, Tyrrell KL, Merriam V, et al. In vitro activity of ceftazidime- NXL104 against 396 strains of beta-lactamase-producing anaerobes. Antimicrob Agents Chemother 2011;55:3616-20. [Crossref] [PubMed]
- Haidar G, Clancy CJ, Shields RK, et al. Mutations in blaKPC-3 that confer ceftazidime-avibactam resistance encode novel KPC-3 variants that function as extended-spectrum beta-lactamases. Antimicrob Agents Chemother 2017;61:e02534-16. [Crossref] [PubMed]
- Flamm RK, Sader HS, Farrell DJ, et al. Ceftazidime-avibactam and comparator agents tested against urinary tract isolates from a global surveillance program (2011). Diagn Microbiol Infect Dis 2014;80:233e8.
- Levasseur P, Girard AM, Claudon M, et al. In vitro antibacterial activity of the ceftazidime-avibactam (NXL104) combination against Pseudomonas aeruginosa clinical isolates. Antimicrob Agents Chemother 2012;56:1606-8. [Crossref] [PubMed]
- Sader HS, Castanheira M, Mendes RE, et al. Ceftazidime- avibactam activity against multidrug-resistant Pseudomonas aeruginosa isolated in U.S. medical centers in 2012 and 2013. Antimicrob Agents Chemother 2015;59:3656-9. [Crossref] [PubMed]
- Flamm RK, Stone GG, Sader HS, et al. Avibactam reverts the ceftazidime MIC90 of European Gram-negative bacterial clinical isolates to the epidemiological cut-off value. J Chemother 2014;26:333-8. [Crossref] [PubMed]
- Walkty A, DeCorby M, Lagace-Wiens PR, et al. In vitro activity of ceftazidime combined with NXL104 versus Pseudomonas aeruginosa isolates obtained from patients in Canadian hospitals (CANWARD 2009 study). Antimicrob Agents Chemother 2011;55:2992-4. [Crossref] [PubMed]
- Winkler ML, Papp-Wallace KM, Hujer AM, et al. Unexpected challenges in treating multidrugresistant Gram-negative bacteria: resistance to ceftazidime-avibactam in archived isolates of Pseudomonas aeruginosa. Antimicrob Agents Chemother 2015;59:1020-9. [Crossref] [PubMed]
- Flamm RK, Nichols WW, Sader HS, et al. In vitro activity of ceftazidime/avibactam against Gram negative pathogens isolated from pneumonia in hospitalised patients, including ventilated patients. Int J Antimicrob Agents 2016;47:235-42. [Crossref] [PubMed]
- Sader HS, Castanheira M, Flamm RK. Antimicrobial activity of ceftazidime-avibactam when tested against gram-negative bacteria isolated from patients hospitalized with pneumonia in U.S. Medical Centers, 2011 to 2015. Antimicrob Agents Chemother 2017;61:e02083-16. [Crossref] [PubMed]
- Shields RK, Clancy CJ, Hao B, et al. Effects of Klebsiella pneumoniae carbapenemase subtypes, extended-spectrum b-lactamases, and porin mutations on the in vitro activity of ceftazidime avibactam against carbapenem-resistant K. pneumoniae. Antimicrob Agents Chemother 2015;59:5793-7. [Crossref] [PubMed]
- Temkin E, Torre-Cisneros J, Beovic B, et al. Ceftazidime-avibactam as salvage therapy for infections caused by carbapenem-resistant organisms. Antimicrob Agents Chemother 2017;61:e01964-16. [PubMed]
- Shields RK, Chen L, Cheng S, et al. Emergence of Ceftazidime-Avibactam Resistance Due to Plasmid-Borne blaKPC-3 Mutations during Treatment of Carbapenem-Resistant Klebsiella pneumoniae Infections. Antimicrob Agents Chemother 2017;61:e02097-16. [PubMed]
- Barnes MD, Winkler ML, Taracila MA, et al. Klebsiella pneumoniae Carbapenemase-2 (KPC-2), Substitutions at Ambler Position Asp179, and Resistance to Ceftazidime-Avibactam: Unique Antibiotic-Resistant Phenotypes Emerge from β-Lactamase Protein Engineering MBio. 20171;8:e00528-17.
- Compain F, Arthur M. Impaired Inhibition by Avibactam and Resistance to the Ceftazidime-Avibactam Combination Due to the D179Y Substitution in the KPC-2 β-Lactamase. Antimicrob Agents Chemother 2017;61:e00451-17. [Crossref] [PubMed]
- Cousson J, Floch T, Guillard T, et al. Lung concentrations of ceftazidime administered by continuous versus intermittent infusion in patients with ventilator-associated pneumonia. Antimicrob Agents Chemother 2015;59:1905-9. [Crossref] [PubMed]
- Tominaga N, Edeki T, Li J, et al. Phase I study assessing the safety, tolerability, and pharmacokinetics of avibactam and ceftazidime-avibactam in healthy Japanese volunteers. J Infect Chemother 2015;21:551-8. [Crossref] [PubMed]
- Merdjan H, Tarral A, Das S, et al. Phase 1 Study Assessing the Pharmacokinetic Profile and Safety of Avibactam in Patients With Renal Impairment. J Clin Pharmacol 2017;57:211-8. [Crossref] [PubMed]
- Allergan, Summary of Product Characteristics, Food and Drug Administration, Ceftazidime avibactam (Avycaz™). Available online: https://www.allergan.com/assets/pdf/avycaz_pi, Accessed 18 July 2018.
- AstraZeneca. Summary of Product Characteristics: European Medicines Agency SPC for ceftazidime avibactam (Zavicefta™), 2016. Available online: https://www.medicines.org.uk/emc/medicine/33061, Accessed 18 July 2018.
- Falcone M, Viale P, Tiseo G, et al. Pharmacokinetic drug evaluation of avibactam + ceftazidime for the treatment of hospital-acquired pneumonia. Expert Opin Drug Metab Toxicol 2018;14:331-40. [Crossref] [PubMed]
- MacGowan A, Tomaselli S, Noel A, et al. The pharmacodynamics of avibactam in combination with ceftaroline or ceftazidime against β-lactamase-producing Enterobacteriaceae studied in an in vitro model of infection. J Antimicrob Chemother 2017;72:762-9. [PubMed]
- Nicolau DP, Siew L, Armstrong J, et al. Phase 1 study assessing the steady-state concentration of ceftazidime and avibactam in plasma and epithelial lining fluid following two dosing regimens. J Antimicrob Chemother 2015;70:2862-9. [Crossref] [PubMed]
- Boselli E, Breilh D, Rimmelé T, et al. Alveolar concentrations of piperacillin/tazobactam administered in continuous infusion to patients with ventilator-associated pneumonia. Crit Care Med 2008;36:1500-6. [Crossref] [PubMed]
- Das S, Nichols W, Li J. Dose selection of ceftazidime-avibactam in patients with nosocomial pneumonia, including ventilator-associated pneumonia, based on preclinical efficacy, preclinical pharmacokinetic/pharmacodynamic and clinical pharmacokinetic data [Poster P1288]. 27th ECCMID, 2017, Vienna, Austria.
- Mazuski JE, Gasink LB, Armstrong J, et al. Efficacy and safety of ceftazidime-avibactam plus metronidazole versus meropenem in the treatment of complicated intra-abdominal infection:results from a randomized, controlled, double-blind, phase 3 program. Clin Infect Dis 2016;62:1380-9. [Crossref] [PubMed]
- Wagenlehner FM, Sobel JD, Newell P, et al. Ceftazidime-avibactam versus doripenem for the treatment of complicated urinary tract infections, including acute pyelonephritis: RECAPTURE, a phase 3 randomized trial program. Clin Infect Dis 2016;63:754-62. [Crossref] [PubMed]
- Carmeli Y, Armstrong J, Laud PJ, et al. Ceftazidime-avibactam or best available therapy in patients with ceftazidime resistant Enterobacteriaceae and Pseudomonas aeruginosa complicated urinary tract infections or complicated intra-abdominal infections (REPRISE):a randomised, pathogen-directed, phase 3 study. Lancet Infect Dis 2016;16:661-73. [Crossref] [PubMed]
- Torres A, Zhong N, Pachl J, et al. Ceftazidime-avibactam versus meropenem in nosocomial pneumonia, including ventilator-associated pneumonia (REPROVE):a randomised, double-blind, phase 3 non-inferiority trial. Lancet Infect Dis 2018;18:285-95. [Crossref] [PubMed]
- Li J, Zhou D, Das S, et al. PK/PD target attainment analyses and assessment of dose adjustments for renal insufficiency for ceftazidime-avibactam (CAZ-AVI) in patients with complicated intra-abdominal infection (cIAI), complicated urinary tract infection (cUTI) or nosocomial pneumonia (NP). American Association of Pharmaceutical Scientists (AAPS) Annual Meeting and Exposition; 2015 October 25, 2015; Orlando, FL, USA; 2015. p. Abstract 2459.
- Shields RK, Potoski BA, Haidar G, et al. Clinical outcomes, drug toxicity, and emergence of ceftazidime-avibactam resistance among patients treated for carbapenem-resistant Enterobacteriaceae infections. Clin Infect Dis 2016;63:1615-8. [Crossref] [PubMed]
- Gallagher JC, McKinnell J, CAZAVI Compassionate Use Research Group. Compassionate use of ceftazidime/avibactam (Cazavi) for carbapenem resistant (Cr) Gram-negative rod infections. Abst 428. Abst ASM Microbe. Am Soc Microbiol June 17, 2016. Boston MA, USA.
- Shields RK, Nguyen MH, Chen L, et al. Ceftazidime-avibactam is superior to other treatment regimens against carbapenem-resistant Klebsiella pneumoniae bacteremia. Antimicrob Agents Chemother 2017;61:e00883ee17.
- Zhong H, Zhao XY, Zhang ZL, et al. Evaluation of efficacy and safety of ceftazidime-avibactam in the treatment of Gram-negative bacterial infections: a systematic review and meta-analysis. Int J Antimicrob Agents 2018;52:443-50. [Crossref] [PubMed]
- Giacobbe DR, Bassetti M, De Rosa FG, et al. Ceftolozane/tazobactam: place in therapy. Expert Rev Anti Infect Ther 2018;16:307-20. [Crossref] [PubMed]
- Moyá B, Zamorano L, Juan C, et al. Affinity of the new cephalosporin CXA-101 to penicillin-binding proteins of Pseudomonas aeruginosa. Antimicrob Agents Chemother 2010;54:3933-7. [Crossref] [PubMed]
- Livermore DM, Mushtaq S, Meunier D, et al. Activity of ceftolozane/tazobactam against surveillance and “problem” Enterobacteriaceae, Pseudomonas aeruginosa and non-fermenters from the British Isles. J Antimicrob Chemother 2017;72:2278-89. [Crossref] [PubMed]
- Cabot G, Bruchmann S, Mulet X, et al. Pseudomonas aeruginosa ceftolozane-tazobactam resistance development requires multiple mutations leading to overexpression and structural modification of AmpC. Antimicrob Agents Chemother 2014;58:3091-9. [Crossref] [PubMed]
- Farrell DJ, Sader HS, Flamm RK, et al. Ceftolozane/tazobactam activity tested against Gram-negative bacterial isolates from hospitalised patients with pneumonia in US and European medical centres (2012). Int J Antimicrob Agents 2014;43:533-9. [Crossref] [PubMed]
- Noel AR, Bowker KE, Attwood M, et al. Antibacterial effect of ceftolozane/tazobactam in combination with amikacin against aerobic Gram-negative bacilli studied in an in vitro pharmacokinetic model of infection. J Antimicrob Chemother 2018;73:2411-17. [Crossref] [PubMed]
- MacGowan AP, Noel AR, Tomaselli SG, et al. Pharmacodynamics of Ceftolozane plus Tazobactam Studied in an In Vitro Pharmacokinetic Model of Infection. Antimicrob Agents Chemother 2015;60:515-21. [Crossref] [PubMed]
- Solomkin J, Hershberger E, Miller B, et al. Ceftolozane/Tazobactam Plus Metronidazole for Complicated Intra-abdominal Infections in an Era of Multidrug Resistance: Results From a Randomized, Double-Blind, Phase 3 Trial (ASPECT-cIAI). Clin Infect Dis 2015;60:1462-71. [Crossref] [PubMed]
- Wagenlehner FM, Umeh O, Steenbergen J, et al. Ceftolozane-tazobactam compared with levofloxacin in the treatment of complicated urinary-tract infections, including pyelonephritis: a randomised, double-blind, phase 3 trial (ASPECT-cUTI). Lancet 2015;385:1949-56. [Crossref] [PubMed]
- Chandorkar G, Huntington JA, Gotfried MH, et al. Intrapulmonary penetration of ceftolozane/tazobactam and piperacillin/tazobactam in healthy adult subjects. J Antimicrob Chemother 2012;67:2463-9. [Crossref] [PubMed]
- Xiao AJ, Miller BW, Huntington JA, et al. Ceftolozane/tazobactam pharmacokinetic/ pharmacodynamic-derived dose justification for phase 3 studies in patients with nosocomial pneumonia. J Clin Pharmacol 2016;56:56-66. [Crossref] [PubMed]
- Yu B, Adedoyin A, Hershberger E, et al. Safety, Tolerability, and Pharmacokinetics of 3 g of Ceftolozane/Tazobactam in Healthy Adults: A Randomized, Placebo-Controlled, Multiple-Dose Study. Clin Pharmacol Drug Dev 2018;7:382-91. [Crossref] [PubMed]
- Bretonnière C, Boutoille D, Caillon J, et al. In vivo efficacy of ceftolozane against Pseudomonas aeruginosa in a rabbit experimental model of pneumonia: comparison with ceftazidime, piperacillin/tazobactam and imipenem. Int J Antimicrob Agents 2014;44:218-21. [Crossref] [PubMed]
- Gelfand MS, Cleveland KO. Ceftolozane/Tazobactam Therapy of Respiratory Infections due to Multidrug-Resistant Pseudomonas aeruginosa. Clin Infect Dis 2015;61:853-5. [Crossref] [PubMed]
- Castón JJ, De la Torre Á, Ruiz-Camps I, et al. Salvage Therapy with Ceftolozane-Tazobactam for Multidrug-Resistant Pseudomonas aeruginosa Infections. Antimicrob Agents Chemother 2017;61:e02136-16. [Crossref] [PubMed]
- Haidar G, Philips NJ, Shields RK, et al. Ceftolozane-Tazobactam for the Treatment of Multidrug-Resistant Pseudomonas aeruginosa Infections: Clinical Effectiveness and Evolution of Resistance. Clin Infect Dis 2017;65:110-20. [Crossref] [PubMed]
- Munita JM, Aitken SL, Miller WR, et al. Multicenter Evaluation of Ceftolozane/Tazobactam for Serious Infections Caused by Carbapenem-Resistant Pseudomonas aeruginosa. Clin Infect Dis 2017;65:158-61. [Crossref] [PubMed]
- Escolà-Vergé L, Pigrau C, Los-Arcos I, et al. Ceftolozane/tazobactam for the treatment of XDR Pseudomonas aeruginosa infections. Infection 2018;46:461-8. [Crossref] [PubMed]
- Katchanov J, Asar L, Klupp EM, et al. Carbapenem-resistant Gram-negative pathogens in a German university medical center: Prevalence, clinical implications and the role of novel β-lactam/β-lactamase inhibitor combinations. PLoS ONE 2018;13:e0195757. [Crossref] [PubMed]
- Xipell M, Paredes S, Fresco L, et al. Clinical experience with ceftolozane/tazobactam in patients with serious infections due to resistant Pseudomonas aeruginosa. J Glob Antimicrob Resist 2018;13:165-70. [Crossref] [PubMed]
- Álvarez Lerma F, Muñoz Bermudez R, Grau S, et al. Ceftolozane-tazobactam for the treatment of ventilator-associated infections by colistin-resistant Pseudomonas aeruginosa. Rev Esp Quimioter 2017;30:224-8. [PubMed]
- Plant AJ, Dunn A, Porter RJ. Ceftolozane-tazobactam resistance induced in vivo during the treatment of MDR Pseudomonas aeruginosa pneumonia. Expert Rev Anti Infect Ther 2018;16:367-8. [Crossref] [PubMed]
- Popejoy MW, Paterson DL, Cloutier D, et al. Efficacy of ceftolozane/tazobactam against urinary tract and intra-abdominal infections caused by ESBL-producing Escherichia coli and Klebsiella pneumoniae: a pooled analysis of Phase 3 clinical trials. J Antimicrob Chemother 2017;72:268-72. [Crossref] [PubMed]
- Xiao AJ, Caro L, Popejoy MW, et al. PK/PD Target Attainment With Ceftolozane/Tazobactam Using Monte Carlo Simulation in Patients With Various Degrees of Renal Function, Including Augmented Renal Clearance and End-Stage Renal Disease. Infect Dis Ther 2017;6:137-48. [Crossref] [PubMed]
- Kullar R, Wagenlehner FM, Popejoy MW, et al. Does moderate renal impairment affect clinical outcomes in complicated intra-abdominal and complicated urinary tract infections? Analysis of two randomized controlled trials with ceftolozane/tazobactam. J Antimicrob Chemother 2017;72:900-5. [PubMed]
- Alessa MA, Almangour TA, Alhossan A, et al. Ceftolozane-tazobactam for the treatment of multidrug-resistant Pseudomonas aeruginosa pneumonia in a patient receiving intermittent hemodialysis. Am J Health Syst Pharm 2018;75:e184-8. [Crossref] [PubMed]
- Oliver WD, Heil EL, Gonzales JP, et al. Ceftolozane-Tazobactam Pharmacokinetics in a Critically Ill Patient on Continuous Venovenous Hemofiltration. Antimicrob Agents Chemother 2015;60:1899-901. [Crossref] [PubMed]
- Bremmer DN, Nicolau DP, Burcham P, et al. Ceftolozane/Tazobactam Pharmacokinetics in a Critically Ill Adult Receiving Continuous Renal Replacement Therapy. Pharmacotherapy 2016;36:e30-3. [Crossref] [PubMed]
- Kuti JL, Ghazi IM, Quintiliani R, et al. Treatment of multidrug-resistant Pseudomonas aeruginosa with ceftolozane/tazobactam in a critically ill patient receiving continuous venovenous haemodiafiltration. Int J Antimicrob Agents 2016;48:342-3. [Crossref] [PubMed]
- Natesan S, Pai MP, Lodise TP. Determination of alternative ceftolozane/tazobactam dosing regimens for patients with infections due to Pseudomonas aeruginosa with MIC values between 4 and 32 mg/L. J Antimicrob Chemother 2017;72:2813-6. [Crossref] [PubMed]
- Goodlet KJ, Nicolau DP, Nailor MD. In Vitro Comparison of Ceftolozane-Tazobactam to Traditional Beta-Lactams and Ceftolozane-Tazobactam as an Alternative to Combination Antimicrobial Therapy for Pseudomonas aeruginosa. Antimicrob Agents Chemother 2017;61:e01350-17. [Crossref] [PubMed]
- Torres A, Niederman MS, Chastre J, et al. Summary of the international clinical guidelines for the management of hospital-acquired and ventilator-acquired pneumonia. ERJ Open Res. 2018;4:e00028-2018. [Crossref] [PubMed]
- Galani I, Souli M, Chryssouli Z, et al. First identification of an Escherichia coli clinical isolate producing both metallo-beta-lactamaseVIM-2 and extended-spectrum beta-lactamase IBC-1. Clin Microbiol Infect 2004;10:757-60. [Crossref] [PubMed]
- Biedenbach DJ, Kazmierczak K, Bouchillon SK, et al. In vitro activity of aztreonam-avibactam against a global collection of Gram-negative pathogens from 2012 and 2013. Antimicrob Agents Chemother 2015;59:4239-48. [Crossref] [PubMed]
- Sy SKB, Beaudoin ME, Zhuang L, et al. In vitro pharmacokinetics/ pharmacodynamics of the combination of avibactam and aztreonam against MDR organisms. J Antimicrob Chemother 2016;71:1866-80. [Crossref] [PubMed]
- Dong Y, Toleman MA, Giske CG, et al. Characterization of a new metallo-β-lactamase gene, blaNDM-1, and a novel erythromycin esterase gene carried on a unique genetic structure in Klebsiella pneumoniae sequence type 14 from India. Antimicrob Agents Chermother 2009;53:5046-54. [Crossref]
- Vasoo S, Cunningham SA, Cole NC, et al. In Vitro Activities of Ceftazidime-Avibactam, Aztreonam-Avibactam, and a Panel of Older and Contemporary Antimicrobial Agents against Carbapenemase-Producing Gram-Negative Bacilli. Antimicrob Agents Chemother 2015;59:7842-6. [Crossref] [PubMed]
- Li H, Estabrook M, Jacoby GA, et al. In vitro susceptibility of characterized β-lactamase-producing strain tested with avibactam combinations. Antimicrob Agents Chemother 2015;59:1789-93. [Crossref] [PubMed]
- Wang X, Zhang F, Zhao C, et al. In vitro activities of ceftazidime-avibactam and aztreonam-avibactam against 372 Gram-negative bacilli collected in 2011 and 2012 from 11 teaching hospitals in China. Antimicrob Agents Chemother 2014;58:1774-8. [Crossref] [PubMed]
- Dupont H, Gaillot O, Goetgheluck AS, et al. Molecular Characterization of Carbapenem-Nonsusceptible Enterobacterial Isolates Collected during a Prospective Interregional Survey in France and Susceptibility to the Novel Ceftazidime-Avibactam and Aztreonam-Avibactam Combinations. Antimicrob Agents Chemother 2015;60:215-21. [Crossref] [PubMed]
- Kazmierczak KM, Biedenbach DJ, Hackel M, et al. Global Dissemination of blaKPC into Bacterial Species beyond Klebsiella pneumoniae and In Vitro Susceptibility to Ceftazidime-Avibactam and Aztreonam-Avibactam. Antimicrob Agents Chemother 2016;60:4490-500. [Crossref] [PubMed]
- Chahine EB. Ceftaroline: potential role and implications in an era of escalating antimicrobial resistance. Am J Health Syst Pharm 2011;68:489. [Crossref] [PubMed]
- SPC of ceftaroline fosamil (Teflaro™). Available online: https://www.teflaro.com/, Accessed 28 July 2018.
- European Medicines Agency, SPC of ceftaroline fosamil (Zinforo™). Available online: http://www.ema.europa.eu/docs/en_GB/document_library/EPAR_-_Product_Information/human/002252/WC500132586.pdf, Accessed 28 July 2018.
- Tattevin P, Boutoille D, Vitrat V, et al. Salvage treatment of methicillin-resistant staphylococcal endocarditis with ceftaroline: a multicentre observational study. J Antimicrob Chemother 2014;69:2010-3. [Crossref] [PubMed]
- Riccobene TA, Su SF, Rank D. Single- and multiple-dose study to determine the safety, tolerability, and pharmacokinetics of ceftaroline fosamil in combination with avibactam in healthy subjects. Antimicrob Agents Chemother 2013;57:1496-504. [Crossref] [PubMed]
- Ghamrawi RJ, Neuner E, Rehm SJ. Ceftaroline fosamil: A super-cephalosporin? Cleve Clin J Med 2015;82:437-44. [Crossref] [PubMed]
- Yu S, Teng C, Bai X, et al. Optimization of Siderophore Production by Bacillus sp. PZ-1 and Its Potential Enhancement of Phytoextration of Pb from Soil. J Microbiol Biotechnol 2017;27:1500-12. [PubMed]
- Górska A, Sloderbach A, Marszałł MP. Siderophore-drug complexes:potential medicinal applications of the 'Trojan horse' strategy. Trends Pharmacol Sci 2014;35:442-9. [Crossref] [PubMed]
- Katsube T, Echols R, Arjona Ferreira JC, et al. Cefiderocol, a Siderophore Cephalosporin for Gram-Negative Bacterial Infections: Pharmacokinetics and Safety in Subjects With Renal Impairment. J Clin Pharmacol 2017;57:584-91. [Crossref] [PubMed]
- Shin HS, Satsu H, Bae MJ, et al. Catechol Groups Enable Reactive Oxygen Species Scavenging-Mediated Suppression of PKD-NFkappaB-IL-8 Signaling Pathway by Chlorogenic and Caffeic Acids in Human Intestinal Cells. Nutrients 2017;9:E165. [Crossref] [PubMed]
- Portsmouth S, Van Veenhuyzen D, Echols R, et al. Clinical response of Cefiderocol Compared with Imipenem/Cilastatin in the Treatment of Adults with Complicated Urinary Tract Infections with or without Pyelonephritis or Acute Uncomplicated Pyelonephritis: Results from a Multicenter, Doubleblind, Randomized Study (APEKS-cUTI). Open Forum Infect Dis 2017;4:S537-8. [Crossref]
- Hackel MA, Tsuji M, Yamano Y, et al. In vitro activity of the siderophore cephalosporin, cefiderocol, against a recent collection of clinically relevant Gram-negative bacilli from North America and Europe, including carbapenem non-susceptible isolates: The SIDEROWT- 2014 Study. Antimicrob Agents Chemother 2017;61:1-22. [Crossref] [PubMed]
- Falagas ME, Skalidis T, Vardakas KZ, et al. Activity of cefiderocol (S-649266) against carbapenem-resistant Gram-negative bacteria collected from inpatients in Greek hospitals. J Antimicrob Chemother 2017;72:1704-8. [Crossref] [PubMed]
- Monogue ML, Tsuji M, Yamano Y, et al. Efficacy of humanized exposures of cefiderocol (S-649266) against a diverse population of Gram-negative bacteria in the murine thigh infection model. Antimicrob Agents Chemother 2017;61:1-10. [Crossref] [PubMed]
- Shionogi Presents Positive Clinical Efficacy Trial Results And In Vitro Data On Cefiderocol at IDWeek 2017 available from PR Newswire. Available online: https://www.prnewswire.com/news-releases/shionogi-presents-positiveclinical- efficacy-trial-results-and-in-vitro-data-on-cefiderocol-at-idweek-2017-300531234.html, Accessed 31 July 2018.
- Hecker SJ, Reddy KR, Totrov M, et al. Discovery of a Cyclic Boronic Acid β-Lactamase Inhibitor (RPX7009) with Utility vs Class A Serine Carbapenemases. J Med Chem 2015;58:3682-92. [Crossref] [PubMed]
- Lapuebla A, Abdallah M, Olafisoye O, et al. Activity of meropenem combined with RPX7009, a novel beta-lactamase inhibitor, against Gram-negative clinical isolates in New York City. Antimicrob Agents Chemother 2015;59:4856-60. [Crossref] [PubMed]
- Castanheira M, Rhomberg PR, Flamm RK, et al. Effect of the beta-Lactamase Inhibitor Vaborbactam Combined with Meropenem against Serine Carbapenemase-Producing Enterobacteriaceae. Antimicrob Agents Chemother 2016;60:5454-8. [Crossref] [PubMed]
- Wenzler E, Gotfried MH, Loutit JS, et al. Meropenem-RPX7009 Concentrations in Plasma, Epithelial Lining Fluid, and Alveolar Macrophages of Healthy Adult Subjects. Antimicrob Agents Chemother 2015;59:7232-9. [Crossref] [PubMed]
- Kaye KS, Bhowmick T, Metallidis S, et al. Effect of Meropenem-Vaborbactam vs Piperacillin-Tazobactam on Clinical Cure or Improvement and Microbial Eradication in Complicated Urinary Tract Infection: The TANGO I Randomized Clinical Trial. JAMA 2018;319:788-99. [Crossref] [PubMed]
- Available online: https://www.fda.gov/newsevents/newsroom/pressannouncements/ucm573955.htm, Accessed 28 July 2018.
- Website of The Medicines Company, announcing TANGO 2 trial results. Available online: http://www.themedicinescompany.com/investors/news/medicines-company-announces-tango-2-trial-meropenem-vaborbactam-formerly-carbavance, Accessed 28 July 2018.
- Paterson DL, Kwak EJ, Bhowmick T, et al. Meropenem-Vaborbactam vs. Best Available Therapy for Carbapenem-Resistant Enterobacteriaceae Infections in TANGO II: Outcomes in Immunocompromised Patients. Open Forum Infectious Diseases 2017;4:S537. [Crossref]
- Blizzard TA, Chen H, Kim S, et al. Discovery of MK-7655, a β-lactamase inhibitor for combination with Primaxin®. Bioorg Med Chem Lett 2014;24:780-5. [Crossref] [PubMed]
- Lapuebla A, Abdallah M, Olafisoye O, et al. Activity of Imipenem with Relebactam against Gram-Negative Pathogens from New York City. Antimicrob Agents Chemother 2015;59:5029-31. [Crossref] [PubMed]
- Hirsch EB, Ledesma KR, Chang KT, et al. In vitro activity of MK-7655, a novel β-lactamase inhibitor, in combination with imipenem against carbapenem-resistant Gram-negative bacteria. Antimicrob Agents Chemother 2012;56:3753-7. [Crossref] [PubMed]
- Mavridou E, Melchers RJ, van Mil AC, et al. Pharmacodynamics of imipenem in combination with β-lactamase inhibitor MK7655 in a murine thigh model. Antimicrob Agents Chemother 2015;59:790-5. [Crossref] [PubMed]
- Rhee E, Jumes P, Rizk M, et al. Intrapulmonary pharmacokinetics of MK-7655, a novel b lactamase inhibitor, dosed in combination with imipenem/cilastatin in healthy subjects. Presented in the 53rd Interscience Conference of Antimicrobial Agents and Chemotherapy 2013; P1028, Denver, US.
- Sims M, Mariyanovski V, McLeroth P, et al. Prospective, randomized, double-blind, Phase 2 dose-ranging study comparing efficacy and safety of imipenem/cilastatin plus relebactam with imipenem/cilastatin alone in patients with complicated urinary tract infections. J Antimicrob Chemother 2017;72:2616-26. [Crossref] [PubMed]
- Bassetti M, Righi E. Eravacycline for the treatment of intra-abdominal infections. Expert Opin Investig Drugs 2014;23:1575-84. [Crossref] [PubMed]
- Abdallah M, Olafisoye O, Cortes C, et al. Activity of eravacycline against Enterobacteriaceae and Acinetobacter baumannii, including multidrug-resistant isolates, from New York City. Antimicrob Agents Chemother 2015;59:1802-5. [Crossref] [PubMed]
- Snydman DR, McDermott LA, Jacobus NV, et al. Evaluation of the in vitro activity of eravacycline against a broad spectrum of recent clinical anaerobic isolates. Antimicrob Agents Chemother 2018;62:e02206-17. [Crossref] [PubMed]
- Thabit AK, Monogue ML, Newman JV, et al. Assessment of in vivo efficacy of eravacycline against Enterobacteriaceae exhibiting various resistance mechanisms: a dose-ranging study and pharmacokinetic/pharmacodynamic analysis. Int J Antimicrob Agents 2018;51:727-32. [Crossref] [PubMed]
- Clark RB, Hunt DK, He M, et al. Fluorocyclines 2. Optimization of the C-9side-chain for antibacterial activity and oral efficacy. J Med Chem 2012;55:606-22. [Crossref] [PubMed]
- Livermore DM, Mushtaq S, Warner M, et al. In Vitro Activity of Eravacycline against Carbapenem-Resistant Enterobacteriaceae and Acinetobacter baumannii. Antimicrob Agents Chemother 2016;60:3840-4. [Crossref] [PubMed]
- Grossman TH, Murphy TM, Slee AM, et al. Eravacycline (TP-434) is efficacious in animal models of infection. Antimicrob Agents Chemother 2015;59:2567-71. [Crossref] [PubMed]
- Connors KP, Housman ST, Pope JS, et al. Phase I, open-label, safety and pharmacokinetic study to assess bronchopulmonary disposition of intravenous eravacycline in healthy men and women. Antimicrob Agents Chemother 2014;58:2113-8. [Crossref] [PubMed]
- Zhao M, Lepak AJ, Marchillo K, et al. In Vivo Pharmacodynamic Target Assessment of Eravacycline against Escherichia coli in a Murine Thigh Infection Model. Antimicrob Agents Chemother 2017;61:e00250-17. [Crossref] [PubMed]
- Solomkin JS, Ramesh MK, Cesnauskas G, et al. Phase 2, randomized, double-blind study of the efficacy and safety of two dose regimens of eravacycline versus ertapenem for adult community-acquired complicated intra-abdominal infections. Antimicrob Agents Chemother 2014;58:1847-54. [Crossref] [PubMed]
- Solomkin J, Evans D, Slepavicius A, et al. Assessing the Efficacy and Safety of Eravacycline vs Ertapenem in Complicated Intra-abdominal Infections in the Investigating Gram-Negative Infections Treated With Eravacycline (IGNITE 1) Trial: A Randomized Clinical Trial. JAMA Surg 2017;152:224-32. [Crossref] [PubMed]
- Tetraphase Pharmaceuticals website announcing data pooled analysis. Available online: http://ir.tphase.com/news-releases/news-release-details/tetraphase-pharmaceuticals-announces-data-pooled-analysis, Accessed 28 July 2018.
- Tetraphase announces approval of eravacysline for the treatment of cIAIs. Available online: https://ir.tphase.com/news-releases/news-release-details/tetraphase-pharmaceuticals-announces-fda-approval-xeravatm, Assessed August 27, 2018.
- Burkhardt O, Rauch K, Kaever V, et al. Tigecycline possibly underdosed for the treatment of pneumonia: a pharmacokinetic viewpoint. Int J Antimicrob Agents 2009;34:101-2. [Crossref] [PubMed]
- Giamarellou H, Poulakou G. Pharmacokinetic and pharmacodynamic evaluation of tigecycline. Expert Opin Drug Metab Toxicol 2011;7:1459-70. [Crossref] [PubMed]
- Poulakou G, Bassetti M, Righi E, Dimopoulos G. Current and future treatment options for infections caused by multidrug-resistant Gram-negative pathogens. Future Microbiol 2014;9:1053-69. [Crossref] [PubMed]
- Zhanel GG, Lawson CD, Zelenitsky S, et al. Comparison of the next-generation aminoglycoside plazomicin to gentamicin, tobramycin and amikacin. Expert Rev Anti Infect Ther 2012;10:459-73. [Crossref] [PubMed]
- Livermore DM, Mushtaq S, Warner M, et al. Activity of aminoglycosides, including ACHN-490, against carbapenem resistant Enterobacteriaceae isolates. J Antimicrob Chemother 2011;66:48-53. [Crossref] [PubMed]
- Endimiani A, Hujer KM, Hujer AM, et al. ACHN-490, a neoglycoside with potent in vitro activity against multidrug-resistant Klebsiella pneumoniae isolates. Antimicrob Agents Chemother 2009;53:4504-7. [PubMed]
- Walkty A, Adam H, Baxter M, et al. In vitro activity of plazomicin against 5,015 Gram-negative and Gram-positive clinical isolates obtained from patients in Canadian hospitals as part of the CANWARD study, 2011-2012. Antimicrob Agents Chemother 2014;58:2554-63. [Crossref] [PubMed]
- Landman D, Kelly P, Backer M, et al. Antimicrobial activity of a novel aminoglycoside, ACHN-490, against Acinetobacter baumannii and Pseudomonas aeruginosa from New York City. J Antimicrob Chemother 2011;66:332-4. [Crossref] [PubMed]
- Galani I, Souli M, Daikos GL, et al. Activity of plazomicin (ACHN-490) against MDR clinical isolates of Klebsiella pneumoniae, Escherichia coli, and Enterobacter spp. from Athens, Greece. J Chemother 2012;24:191-4. [Crossref] [PubMed]
- Cass RT, Brooks CD, Havrilla NA, et al. Pharmacokinetics and safety of single and multiple doses of ACHN-490 injection administered intravenously in healthy subjects. Antimicrob Agents Chemother 2011;55:5874-80. [Crossref] [PubMed]
- Karaiskos I, Souli M, Giamarellou H. Plazomicin:an investigational therapy for the treatment of urinary tract infections. Expert Opin Investig Drugs 2015;24:1501-11. [Crossref] [PubMed]
- Connolly LE, Riddle V, Cebrik D, et al. A Multicenter, Randomized, Double-Blind, Phase 2 Study of the Efficacy and Safety of Plazomicin Compared with Levofloxacin in the Treatment of Complicated Urinary Tract Infection and Acute Pyelonephritis. Antimicrob Agents Chemother 2018;62:e01989-17. [Crossref] [PubMed]
- Results of the EPIC trial released in Achaogen’s website. Available online: http://investors.achaogen.com/news-releases/news-release-details/achaogen-announces-positive-results-phase-3-cuti-and-cre, Accessed 29 July 2018.
- McKinnell JA, Connoly LE, Pushkin R, et al. Improved Outcomes with Plazomicin (PLZ) Compared with Colistin (CST) in Patients with Bloodstream Infections (BSI) Caused by Carbapenem-resistant Enterobacteriaceae (CRE): Results from the CARE Study. Open Forum Infectious Diseases 2017;4:S531. [Crossref]
- Connolly LE, Jubb AM, Keeffe BO, et al. Plazomicin Is Associated With Improved Survival and Safety Compared to Colistin in Serious Carbapenem-resistant Enterobacteriaceae (CRE) Infections: Results of the CARE study. Presented at the annual meeting of the American Society of Microbiology, June 1-5, 2017, New Orleans, LA, US.
- FDA approval of plazomicin (ZEMDRI™). Available online: https://www.fda.gov/Drugs/InformationOnDrugs/ucm612925.htm, Accessed 10 August 2018.
- Summary of product Characteristics and Boxed Warning. Available online: http://www.zemdri.com/, Accessed 10 August 2018.
- Srinivas N, Jetter P, Ueberbacher BJ, et al. Peptidomimetic antibiotics target outer-membrane biogenesis in pseudomonas aeruginosa. Science 2010;327:1010-3. [Crossref] [PubMed]
- Botos I, Majdalani N, Mayclin SJ, et al. Structural and functional characterization of the LPS transporter LptDE from gram-negative pathogens. Structure 2016;24:965-6. [Crossref] [PubMed]
- Martin-Loeches I, Dale GE, Torres A. Murepavadin: a new antibiotic class in the pipeline. Expert Rev Anti Infect Ther 2018;16:259-68. [Crossref] [PubMed]
- Sader HS, Biedenbach DJ, Jones RN, et al. In vitro evaluation of antimicrobial spectrum and determination of optimal MIC test conditions for the novel protein epitope mimetics antibiotic POL7080. Presented in the European Congress of Clinical Microbiology and Infectious Diseases 2012;P1456 London, UK.
- Sader HS, Flamm RK, Dale GE, et al. Murepavadin activity tested against contemporary (2016-17) clinical isolates of XDR Pseudomonas aeruginosa. J Antimicrob Chemother 2018;73:2400-4. [Crossref] [PubMed]
- Dembowsky K, Wilbraham D, Ograbek A, et al. Phase I (multiple ascending dose) study with the novel Pseudomonas aeruginosa antibiotic POL7080 in healthy volunteers. Presented in the European Congress of Clinical Microbiology and Infectious Diseases 2012;P1421 London, United Kingdom.
- Wach A, Dembowsky K, Dale GE. Pharmacokinetics and Safety of Intravenous Murepavadin Infusion in Healthy Adult Subjects Administered Single and Multiple Ascending Doses. Antimicrob Agents Chemother 2018;62:e02355-17. [Crossref] [PubMed]
- Dale GE, Halabi A, Petersen-Sylla M, et al. Pharmacokinetics, Tolerability, and Safety of Murepavadin, a Novel Antipseudomonal Antibiotic, in Subjects with Mild, Moderate, or Severe Renal Function Impairment. Antimicrob Agents Chemother 2018;62:e00490-18. [Crossref] [PubMed]
- Winter E, Boetsch C, Brennan B, et al. Penetration of POL7080/RO7033877 into epithelial lining fluid and alveolar macrophages of healthy volunteers. Presented in the Joint 55th Interscience Conference on Antimicrobial Agents and Chemotherapy and 28th International Congress of Chemotherapy 2015, San Diego, USA.
- Mouton J, Melchers MJ, Teague J, et al. Pharmacokinetics and pharmacodynamics of murepavadin (POL7080) in neutropenic lung infection models. 27th Congress of Clinical Microbiology and Infectious Diseases. Vienna, Austria. 2017, OS1019.14:06-16.
- Armaganidis A, Frantzeskaki F, Diakaki C, et al. Pharmacokinetic and efficacy analysis of murepavadin (POL7080) coadministered with standard-of-care (SoC) in a phase II study in patients with ventilator-associated pneumonia (VAP) due to suspected or documented Pseudomonas aeruginosa infection. 27th Congress of Clinical Microbiology and Infectious Diseases. Vienna, Austria, 2017;12:30-13:30,1308.
- Armaganidis A, Zakynthinos S, Mandragos C, et al. Efficacy of murepavadin coadministered with standard of care in a phase 2 study in patients with ventilator-associated pneumonia due to suspected or documented Pseudomonas aeruginosa infection. ISICEM 2017, Poster A459. Brussels, Belgium.