Old and new insights into the diagnosis of hereditary spherocytosis
Introduction
Hereditary spherocytosis (HS) belongs to the group of congenital hemolytic anemias resulting from plasma membrane protein deficiency (1,2). It is the most common inherited red blood cell (RBC) plasma membrane disorder in Northern Europe and Northern America, and is diagnosed in 1 in every 2000 individuals. Mild forms of the disorder might be asymptomatic, therefore, it is thought that the incidence could be underestimated (3). In people with HS, the lifespan of erythrocytes is shortened, mainly due to decreased deformability of the cell. This results from an altered surface-to-volume ratio. The inability of spherocytes to absorb hypoosmotic fluid makes them osmotically fragile and prone to hemolysis in the spleen (4). The molecular mutations that cause HS may be localized in genes encoding one or more plasma membrane proteins, including ankyrin, band 3 protein, α or β spectrin and protein 4.2 (4-11). Furthermore, the clinical severity may differ between individuals with different genetic mutations. Hemolysis may appear constantly or only occasionally, induced by events such as postinfectious hypersplenism (4). The first symptom of HS in neonates is hyperbilirubinemia which is not associated with blood group type incompatibility (12,13). Non-diagnosed HS may lead to kernicterus, a severe neurological complication, in addition to severe hemolytic anemia and the development of gall stones. Thus, early diagnosis of HS is crucial to reduce the risk of complications later in life (4,12,14). To date, several methods for HS screening and diagnosis have been developed, and these are still undergoing improvements.
Complete blood count (CBC) analysis
The first laboratory tests for HS screening were the mean corpuscular hemoglobin concentration (MCHC) and red blood cell distribution width (RDW) (14,15). Both indices are elevated during the course of HS, allowing the diagnosis of HS with satisfactory sensitivity and specificity (12,16,17). High MCHC in HS individuals is partially assessed by the RBC shape and a method of its measurement (used in conductance-orifice analyzers). The mean corpuscular volume (MCV) and MCHC measurements are based on the analysis of cells in a fluid in which flow is accelerated, causing cells to deform into a “cigar” shape. The MCV is measured in these deformed cells by assessing the cross-sectional area of the cell. Cells with a high hemoglobin concentration or spherocytes, which cannot be deformed due to their shape, affected by plasma membrane protein deficiency, are classified as having a higher MCHC and smaller volume than normal RBCs (18). Beckman Coulter introduced mean sphered corpuscular volume (MSCV) as a way to measure erythrocyte volume in hypoosmotic solutions (2,19). Low MSCV has been demonstrated to be a sensitive parameter for HS detection (20). When expressed as delta MSCV, i.e., the difference between MCV and MSCV (MCV-MSCV), this measurement has even higher discriminatory value for the detection of HS, which is confirmed by increased delta MSCV (19,21-23).
Among the measurements which can be obtained by automated hematology analyzers, reticulocyte parameters have been shown to have high utility for HS screening (2). Patients with HS present a high reticulocyte count with a low immature reticulocyte fraction (IRF). These less mature reticulocytes are recognized as cells with higher RNA levels (21). Mullier et al. showed that mild cases of HS have a Ret/IRF ratio higher than 19, while severe cases of HS have a Ret/IRF ratio higher than 7.7 (15). Lazarova et al. were more cautious with their interpretation of Ret/IRF parameters as different automated analyzers measure IRF with different methods, therefore, they cannot be compared (20). Other parameters measured in regard to reticulocytes are the Hypo-He and Hyper-He indices, which discriminate RBCs with low and high hemoglobin concentrations, respectively. Individuals with HS have a higher Hyper-He count, which is associated with the increased number of microcytic erythrocytes (MicroR), an index measured exclusively by the Sysmex analyzer (15,24,25). Persijn et al. proposed that the threshold for the MicroR value be decreased from ≥3.5% to ≥2.6% to discriminate between HS and non-HS subjects, which would significantly increase the sensitivity of the test (26). A low mean reticulocyte volume (MRV) has also been shown to be a good parameter to distinguish between HS and non-HS subjects; however, it has a lower sensitivity and specificity than other reticulocyte indices (20,21,27). Combining some new parameters into diagnostic algorithms, like MCV-MSCV >10 with MRV-MSCV <25, obtains results with satisfactory sensitivity and specificity (28) (Table 1).
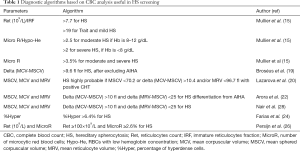
Full table
Osmotic fragility
Osmotic fragile cells easily hemolyze in hypoosmotic solutions. Spherocytes, which are unable to increase in volume due to their surface-to-volume area, cannot absorb hypotonic solutions. As a result, they hemolyze in higher concentrations of NaCl, thus presenting lower osmotic resistance than normal biconcave RBCs. The first osmotic fragility test introduced into routine diagnostics was Dacie’s method. Briefly, RBCs are placed in serial solutions of saline at concentrations ranging from 0.1% to 0.9% NaCl. Hemolysis is evaluated by spectrophotometric measurement of the hemoglobin concentration in the solution (29,30). Acidified glycerol lysis test (AGLT), a modified version of the classic Dacie’s method, was introduced in the early 1980s by Zanella and colleagues. RBCs are transferred into phosphate-buffered saline with a pH of 6.85 (pH is crucial for proper test performance) and placed in the spectrophotometer cuvette. Then, 0.3 M glycerol solution is added to the RBC sample and the decrease in turbidity of the specimen is measured over time. The AGLT50 is the time in which the absorbance of the solution reaches 50% of the absorbance of the solution without glycerol (31). Despite satisfactory sensitivity, the AGLT test has limited specificity and is time consuming, with the analysis taking 30 minutes to perform. The most recent osmotic fragility test, introduced by Won and Suh in 2009, is based on flow cytometric assessment (32). This assay is based on measurement of RBC count in a 0.9% NaCl solution before and after the addition of water. The ratio of the number of RBCs measured during a 30-s acquisition period before the addition of water to the mean RBC count during two gates (time periods) after the addition of water (fifth minute of the analysis), corrected for the dilution factor, represents the actual result of the test (32,33) (Figure 1). Attempts were made to combine flow cytometric assessment with the classic osmotic fragility test (34); however, this proved to be a laborious and time-consuming method which did not gain a foothold. The flow cytometric osmotic fragility (FCOF) test has very high sensitivity and specificity for detecting HS (33,35-38). Moreover, Shim and Won found that the result of the FCOF test is correlated with the clinical severity of the disease (39). Although it represents a sensitive assay which would perfectly complement extended CBC analysis, it has one important limitation (40). As flow cytometers are not available in all routine diagnostic laboratories, FCOF cannot be introduced as a screening test for HS in the international guidelines (41).
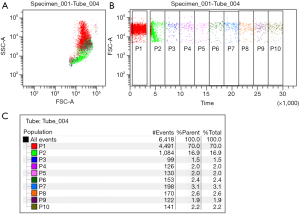
There are two other tests based on measurement of the osmotic fragility and deformability of RBCs, the cryohemolysis test (CHT) and osmotic gradient ektacytometry test. The CHT is based on an observation by Streichman et al., who found that spherocytes are more susceptible to cold temperatures (0 °C) in hypertonic conditions (42). The CHT is more specific for HS diagnosis, as the principle of the test is hemolysis due to affected integrity of membrane proteins rather than an unfavorable volume-to-surface area (43). However, the utility of the CHT is disputable, as the sensitivities and specificities obtained in several studies differ significantly, making this assay unreliable (37,41,43-46). Ektacytometry determines the deformability of cells at a constant shear stress but gradually changing osmolality, which depends on the inner viscosity, volume-to-surface area and viscosity of the cell membrane (47-49). A next-generation osmotic gradient ektacytometry (NG-OGE) assay was recently introduced, which allowed adaption of the primary research method into clinical diagnostics (50). Lazarova et al. found that the NG-OGE is useful for distinguishing between HS and other inherited anemias; however, it cannot differ between HS and autoimmune hemolytic anemia (51). Recent studies demonstrated the three main parameters of the NG-OGE curve which can identify RBCs affected by HS with high sensitivity and specificity: 0min, the osmolality at which 50% of the RBCs are lysed in the classic osmotic fragility test, associated with surface-to-volume ratio; EI max, the maximal elongation index; and area under the curve (AUC) (3,50,51). Although, it has been suggested that NG-OGE could represent an intermediate diagnostic step between screening tests and advanced diagnostic assays, the use of these analyzers is not prevalent. In the most recent HS diagnostic guidelines, NG-OGE was presented as one of the screening tests for HS (2,51).
Eosin-5’-maleimide test
Among the described screening tests for HS, the eosin-5’-maleimide (EMA) binding assay is the most specific for HS (37,52-54). This flow cytometric test with fluorescent EMA dye allows for the detection of RBCs with plasma membrane deficiency with high sensitivity and specificity (46). Eosin-5’-maleimide binds to band 3 protein, but also to CD47 and Rh-associated glycoprotein (55). Its fluorescence corresponds to the plasma membrane protein content on the surface of RBCs (56). When performing the EMA test, 5–6 healthy control samples should be analyzed in parallel for comparison of EMA fluorescence (35). In regard to the results, HS is highly probable when the patient’s RBCs present approximately 85% or less fluorescence than EMA-bound control samples (Figure 2). There are still ongoing studies attempting to improve the utility of the EMA test. Our team proposed that MCV-matched control samples be chosen to avoid obtaining false negative results for macrocytosis (found in newborns) or false positive results for microcytosis (HS with the coexistence of iron deficiency anemia) (57). Agarwal et al. suggested the use of an EMA “footprint”, which is the position of EMA-bound RBCs in cytogram presenting side scatter versus EMA fluorescence channel, in order to allow better interpretation of the test (58). Finally Crisp et al. demonstrated that a very small volume of blood (5 µL), even from capillary blood sampling, is sufficient for straightforward diagnosis (59). The volume of blood needed for the EMA test is a huge advantage of this test. Moreover, several studies have confirmed the stability of RBCs stored under refrigeration, which can bind the same amount of EMA dye as freshly collected samples after up to 7 days of storage (54,60). Moreover, the stability of EMA-bound RBCs was demonstrated in stained samples kept refrigerated for 24 hours (60). However, there is a discrepancy between the presentation of EMA test results between different laboratories. Some prefer to express the result as a percentage of the decrease in EMA fluorescence compared to control RBCs (43,46,53,61,62), while others use the mean fluorescence intensity obtained for EMA-bound cells (63,64) or the EMA “footprint” (52,58). Despite these differences in presentation of EMA test results, it remains the most reliable screening test, with a relatively short time-around-time (TAT), high sensitivity, cost-effectiveness and availability in laboratories equipped with even the most basic flow cytometer. Importantly, the results of the EMA test are not affected by splenectomy (65), and even the smallest populations of RBCs with plasma membrane deficiency can be extracted, including after blood transfusion (66).
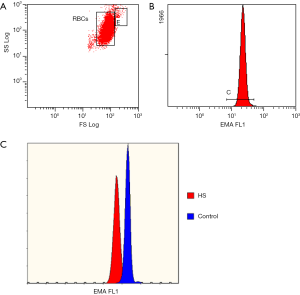
Plasma membrane protein electrophoresis
Although screening tests for HS are characterized by high sensitivity and specificity, they do not indicate which plasma membrane protein is deficient or which genetic mutation is responsible for the observed symptoms. The first-line tests require only a small sample of blood, and can be performed on a specimen collected for CBC. However, final diagnosis requires more specific methods to identify the actual disorder.
To detect plasma membrane deficiency, electrophoresis of disintegrated RBC ghosts is performed. Sodium dodecyl sulfate-polyacrylamide gel electrophoresis (SDS-PAGE) allows for detection of abnormal plasma membrane protein content, either quantitatively or qualitatively [such as band 3 protein modification in congenital dyserythropoietic anemia type II (CDAII)] (2). A disadvantage of SDS-PAGE is the volume of sample needed for analysis. This method is laborious and time consuming, and requires prior preparation of RBC ghosts and removal of hemoglobin from plasma membranes (67). This technique is also only available in highly specialized diagnostic centers, while the availability of the EMA test or osmotic fragility assessment is much more widespread (68). In case of mutation within the ankyrin gene, interpretation of SDS-PAGE might be challenging because it presents as a thin band located directly under the spectrin band (69). Classic SDS-PAGE is a one-dimensional method, which, in 10–20% of cases, does not allow detection of the deficient plasma membrane protein. However, there is no difference in clinical symptoms between patients with characterized or non-characterized defects (44). An improved method, the two-dimensional (2D) electrophoresis of RBC plasma membrane proteins, was introduced to increase the sensitivity of the applied method (70). In this 2D technique, proteins are separated not only based on their molecular weight, but also according to charge. However, it should be taken into consideration that proteolysis, contamination from cytosolic proteins, and variable loss of extrinsic membrane proteins may interfere with the interpretation of 2D electrophoresis, which is not an issue with the one-dimensional technique (71). To improve the precision of plasma membrane protein quantification, the electrophoretic method was adapted for capillary electrophoresis (sodium dodecyl sulphate capillary gel electrophoresis, SDS-CGE). Unfortunately, SDS-CGE shows a lower degree of resolution than SDS-PAGE. Thus, despite being less time-consuming, it will probably not find high utility for this purpose (67).
Molecular diagnostics
HS may be diagnosed based on clinical features, screening tests (including extended CBC), osmotic fragility or EMA test, and finally, plasma membrane electrophoresis, which will reveal the deficient protein. Molecular diagnostics allows for identification of the genetic mutation within genes encoding plasma membrane proteins, the deficiency of which leads to HS. The genetic defects confirmed to cause HS include the SPTA1 gene encoding alpha spectrin, SPTB gene encoding beta-spectrin, ANK1 gene encoding ankyrin, SLC4A1 gene encoding band 3 protein and/or EPB42 gene encoding protein 4.2 (2,5-10,72-77). Mutations in the genes encoding ankyrin, beta spectrin and band 3 proteins are inherited dominantly, whereas mutations in the genes encoding alpha spectrin and protein 4.2 are inherited in a non-dominant manner. Moreover, alpha spectrin and protein 4.2 defects are recessively inherited or arise as de novo mutations (5). Non-dominant HS often bares higher risk of a severe disease course (78). Molecular diagnostics is the most advanced and most expensive method, and is generally the last option, especially in subjects who present symptoms, but the previously mentioned methods do not indicate a direct diagnosis. As the presence of a genetic mutation does not determine the severity of the disease or its course, it is not necessary to obtain precise information regarding the specific gene mutation (2). Molecular diagnostics is used to distinguish between a polymorphism and a gene mutation. Moreover, it may help to predict the disease course if any detrimental polymorphisms are present (2,7,79).
For many years, gene mutations have been detected by polymerase chain reaction [i.e., using single-stranded conformation polymorphism analysis (PCR-SSCP)] (80). Nowadays, applied methods are more advanced, allowing screening for gene mutations over unlimited amounts of DNA. Whole genome sequencing allows the identification of new mutations that cause HS. Advances in molecular diagnostics, from Sanger sequencing through to next-generation sequencing (NGS) and whole exome sequencing, enable the discovery of new mutations and allow for a final diagnosis of HS in unresolved cases with the coexistence of two different diseases (7,81).
Differential diagnosis
Misdiagnosis or missed diagnosis of HS is rare, especially when appropriate laboratory investigations based on screening tests are undertaken. The majority of cases without proper diagnosis are patients who were not subjected to specific screening tests (82). Inherited hemolytic anemias present similar symptoms, thus differential diagnosis is necessary for these cases. Most misdiagnosed cases are HS patients with an asymptomatic form of the disease or who present symptoms suggestive of other diagnoses, such as liver dysfunction. To avoid misdiagnosis, a wide spectrum of specific laboratory tests should be performed, including direct antiglobulin test, hemoglobin electrophoresis and assessing the enzymatic activities of glucose-6-phosphate dehydrogenase (G6PD) and pyruvate kinase (82). Some mild cases are diagnosed following infection with parvovirus B19, which causes aplastic crisis. Laboratory tests performed during the recovery period may help establish a final diagnosis of HS (83). The most sensitive methods used to distinguish between HS and other hemolytic anemias are SDS electrophoresis and molecular diagnostics. Of the several hemolytic anemias with similar symptoms to HS, CDAII patients also show peripheral blood film with spherocytes and normocytic anemia with increased RDW. For differential diagnosis, hypoglycosylation of band 3 protein in CDAII subjects can usually be detected by SDS-PAGE (2). On the other hand, hereditary pyropoikilocytosis (HPP), a severe form of hereditary elliptocytosis (HE), is characterized by decreased fluorescence of EMA-bound RBCs in flow cytometric analysis. Hence, in families with a history of HE, decreased fluorescence in the EMA test should be considered as HPP and not HS (54). Finally, diagnostic algorithms, when properly implemented, would avoid the misdiagnosis of HS.
Summary
Diagnostics for HS, the most common inherited anemia in Northern Europe and Northern America, is still under improvement. Advances in biotechnology and the availability of most modern analyzers for routine and extended laboratory analyses have led to a decrease in the number of undiagnosed or misdiagnosed patients, which has a significantly influence on the development of acute and long-term disease complications. Importantly, expanding the panel of tests with high utility for HS diagnostics to include CBC analysis is the first step to improve the effectiveness of first-line screening and differentiation of inborn hemolytic anemias.
Acknowledgements
None.
Footnote
Conflicts of Interest: The author has no conflicts of interest to declare.
References
- Manciu S, Matei E, Trandafir B. Hereditary Spherocytosis - Diagnosis, Surgical Treatment and Outcomes. A Literature Review. Chirurgia (Bucur) 2017;112:110-6. [Crossref] [PubMed]
- King MJ, Garcon L, Hoyer JD, et al. ICSH guidelines for the laboratory diagnosis of nonimmune hereditary red cell membrane disorders. Int J Lab Hematol 2015;37:304-25. [Crossref] [PubMed]
- Da Costa L, Galimand J, Fenneteau O, et al. Hereditary spherocytosis, elliptocytosis, and other red cell membrane disorders. Blood Rev 2013;27:167-78. [Crossref] [PubMed]
- Bolton-Maggs PH, Langer JC, Iolascon A, et al. General Haematology Task Force of the British Committee for Standards in H. Guidelines for the diagnosis and management of hereditary spherocytosis--2011 update. Br J Haematol 2012;156:37-49. [Crossref] [PubMed]
- Bogardus HH, Maksimova YD, Forget BG, et al. A de novo band 3 mutation in hereditary spherocytosis. Pediatr Blood Cancer 2012;58:1004. [Crossref] [PubMed]
- Bogusławska DM, Heger E, Machnicka B, et al. A new frameshift mutation of the beta-spectrin gene associated with hereditary spherocytosis. Ann Hematol 2017;96:163-5. [Crossref] [PubMed]
- Chen J, Zhou Y, Gao Y, et al. A genetic features and gene interaction study for identifying the genes that cause hereditary spherocytosis. Hematology 2017;22:240-7. [Crossref] [PubMed]
- Guan H, Liang X, Zhang R, et al. Identification of a de novo ANK1 mutation in a Chinese family with hereditary spherocytosis. Hematology 2018;23:357-61. [Crossref] [PubMed]
- Park J, Jeong DC, Yoo J, et al. Mutational characteristics of ANK1 and SPTB genes in hereditary spherocytosis. Clin Genet 2016;90:69-78. [Crossref] [PubMed]
- Van Zwieten R, Francois JJ, Van Leeuwen K, et al. Hereditary spherocytosis due to band 3 deficiency: 15 novel mutations in SLC4A1. Am J Hematol 2013;88:159-60. [Crossref] [PubMed]
- Wang R, Yang S, Xu M, et al. Exome sequencing confirms molecular diagnoses in 38 Chinese families with hereditary spherocytosis. Sci China Life Sci 2018;61:947-53. [Crossref] [PubMed]
- Christensen RD, Yaish HM, Gallagher PG. A pediatrician's practical guide to diagnosing and treating hereditary spherocytosis in neonates. Pediatrics 2015;135:1107-14. [Crossref] [PubMed]
- Will A, Henderson CA, Jnah AJ, et al. Hereditary Spherocytosis in the Neonatal Period: A Case Report. Neonatal Netw 2017;36:280-8. [Crossref] [PubMed]
- Steward SC, Chauvenet AR, O'Suoji C. Hereditary spherocytosis: Consequences of delayed diagnosis. SAGE Open Med 2014;2. [Crossref] [PubMed]
- Mullier F, Lainey E, Fenneteau O, et al. Additional erythrocytic and reticulocytic parameters helpful for diagnosis of hereditary spherocytosis: results of a multicentre study. Ann Hematol 2011;90:759-68. [Crossref] [PubMed]
- Michaels LA, Cohen AR, Zhao H, et al. Screening for hereditary spherocytosis by use of automated erythrocyte indexes. J Pediatr 1997;130:957-60. [Crossref] [PubMed]
- Farias MG. Advances in laboratory diagnosis of hereditary spherocytosis. Clin Chem Lab Med 2017;55:944-8. [Crossref] [PubMed]
- Bull B, Aller R, Houwen B. MCHC -Red Cell Index or Quality Control Parameter? 26th World Congress of the International Society of Haematology 1996.
- Broséus J, Visomblain B, Guy J, et al. Evaluation of mean sphered corpuscular volume for predicting hereditary spherocytosis. Int J Lab Hematol 2010;32:519-23. [Crossref] [PubMed]
- Lazarova E, Pradier O, Cotton F, et al. Automated reticulocyte parameters for hereditary spherocytosis screening. Ann Hematol 2014;93:1809-18. [Crossref] [PubMed]
- Ciepiela O, Adamowicz-Salach A, Radgowska A, et al. Usefulness of Reticulocyte Parameters for Diagnosis of Hereditary Spherocytosis in Children. Indian J Hematol Blood Transfus 2017;33:239-47. [Crossref] [PubMed]
- Arora RD, Dass J, Maydeo S, et al. Utility of mean sphered cell volume and mean reticulocyte volume for the diagnosis of hereditary spherocytosis. Hematology 2018;23:413-6. [Crossref] [PubMed]
- Liao L, Deng ZF, Qiu YL, et al. Values of mean cell volume and mean sphered cell volume can differentiate hereditary spherocytosis and thalassemia. Hematology 2014;19:393-6. [Crossref] [PubMed]
- Farias MG, Freitas PA. Percentage of hyperdense cells: Automated parameter to hereditary spherocytosis screening. Clin Biochem 2015;48:1341-3. [Crossref] [PubMed]
- Rooney S, Hoffmann JJ, Cormack OM, et al. Screening and confirmation of hereditary spherocytosis in children using a CELL-DYN Sapphire haematology analyser. Int J Lab Hematol 2015;37:98-104. [Crossref] [PubMed]
- Persijn L, Bonroy C, Mondelaers V, et al. Screening for hereditary spherocytosis in routine practice: evaluation of a diagnostic algorithm with focus on non-splenectomised patients. Ann Hematol 2012;91:301-2. [Crossref] [PubMed]
- Xu Y, Yang W, Liao L, et al. Mean reticulocyte volume: a specific parameter to screen for hereditary spherocytosis. Eur J Haematol 2016;96:170-4. [Crossref] [PubMed]
- Nair SC, Arora N, Jain S, et al. Mean reticulocyte volume enhances the utility of red cell mean sphered cell volume in differentiating peripheral blood spherocytes of hereditary spherocytosis from other causes. Indian J Pathol Microbiol 2015;58:307-9. [Crossref] [PubMed]
- Walski T, Chludzinska L, Komorowska M, et al. Individual osmotic fragility distribution: a new parameter for determination of the osmotic properties of human red blood cells. Biomed Res Int 2014;2014. [Crossref] [PubMed]
- Zgodzinska A, Ciepiela O. Osmotic fragility of red blood cells – a review of diagnostic methods. Diagn Lab 2015;51:229-34.
- Zanella A, Izzo C, Rebulla P, et al. Acidified glycerol lysis test: a screening test for spherocytosis. Br J Haematol 1980;45:481-6. [Crossref] [PubMed]
- Won DI, Suh JS. Flow cytometric detection of erythrocyte osmotic fragility. Cytometry B Clin Cytom 2009;76:135-41. [Crossref] [PubMed]
- Ciepiela O, Adamowicz-Salach A, Zgodzinska A, et al. Flow cytometric osmotic fragility test: Increased assay sensitivity for clinical application in pediatric hematology. Cytometry B Clin Cytom 2018;94:189-95. [Crossref] [PubMed]
- Yamamoto A, Saito N, Yamauchi Y, et al. Flow cytometric analysis of red blood cell osmotic fragility. J Lab Autom 2014;19:483-7. [Crossref] [PubMed]
- Arora RD, Dass J, Maydeo S, et al. Flow cytometric osmotic fragility test and eosin-5'-maleimide dye-binding tests are better than conventional osmotic fragility tests for the diagnosis of hereditary spherocytosis. Int J Lab Hematol 2018;40:335-42. [Crossref] [PubMed]
- Manivannan P, Tyagi S, Chandra D, et al. Flow cytometric analysis of patients with hereditary spherocytosis - an Indian scenario. Hematology 2018;23:175-80. [Crossref] [PubMed]
- Park SH, Park CJ, Lee BR, et al. Comparison study of the eosin-5'-maleimide binding test, flow cytometric osmotic fragility test, and cryohemolysis test in the diagnosis of hereditary spherocytosis. Am J Clin Pathol 2014;142:474-84. [Crossref] [PubMed]
- Tao YF, Deng ZF, Liao L, et al. Evaluation of a Flow-Cytometric Osmotic Fragility Test for Hereditary Spherocytosis in Chinese Patients. Acta Haematol 2016;135:88-93. [Crossref] [PubMed]
- Shim YJ, Won DI. Flow cytometric osmotic fragility testing does reflect the clinical severity of hereditary spherocytosis. Cytometry B Clin Cytom 2014;86:436-43. [Crossref] [PubMed]
- Tao YF, Deng ZF, Liao L, et al. Comparison and evaluation of three screening tests of hereditary spherocytosis in Chinese patients. Ann Hematol 2015;94:747-51. [Crossref] [PubMed]
- Emilse LAM, Cecilia H, Maria TM, et al. Cryohemolysis, erythrocyte osmotic fragility, and supplementary hematimetric indices in the diagnosis of hereditary spherocytosis. Blood Res 2018;53:10-7. [Crossref] [PubMed]
- Streichman S, Gesheidt Y, Tatarsky I. Hypertonic cryohemolysis: a diagnostic test for hereditary spherocytosis. Am J Hematol 1990;35:104-9. [Crossref] [PubMed]
- Crisp RL, Solari L, Vota D, et al. A prospective study to assess the predictive value for hereditary spherocytosis using five laboratory tests (cryohemolysis test, eosin-5'-maleimide flow cytometry, osmotic fragility test, autohemolysis test, and SDS-PAGE) on 50 hereditary spherocytosis families in Argentina. Ann Hematol 2011;90:625-34. [Crossref] [PubMed]
- Mariani M, Barcellini W, Vercellati C, et al. Clinical and hematologic features of 300 patients affected by hereditary spherocytosis grouped according to the type of the membrane protein defect. Haematologica 2008;93:1310-7. [Crossref] [PubMed]
- Romero RR, Poo JL, Robles JA, et al. Usefulness of cryohemolysis test in the diagnosis of hereditary spherocytosis. Arch Med Res 1997;28:247-51. [PubMed]
- Bianchi P, Fermo E, Vercellati C, et al. Diagnostic power of laboratory tests for hereditary spherocytosis: a comparison study in 150 patients grouped according to molecular and clinical characteristics. Haematologica 2012;97:516-23. [Crossref] [PubMed]
- Nemeth N, Kiss F, Klarik Z, et al. Comparative osmotic gradient ektacytometry data on inter-species differences of experimental animals. Clin Hemorheol Microcirc 2014;57:1-8. [PubMed]
- Clark MR, Mohandas N, Shohet SB. Osmotic gradient ektacytometry: comprehensive characterization of red cell volume and surface maintenance. Blood 1983;61:899-910. [PubMed]
- Nemeth N, Kiss F, Miszti-Blasius K. Interpretation of osmotic gradient ektacytometry (osmoscan) data: a comparative study for methodological standards. Scand J Clin Lab Invest 2015;75:213-22. [Crossref] [PubMed]
- Llaudet-Planas E, Vives-Corrons JL, Rizzuto V, et al. Osmotic gradient ektacytometry: A valuable screening test for hereditary spherocytosis and other red blood cell membrane disorders. Int J Lab Hematol 2018;40:94-102. [Crossref] [PubMed]
- Lazarova E, Gulbis B, Oirschot BV, van Wijk R., et al. Next-generation osmotic gradient ektacytometry for the diagnosis of hereditary spherocytosis: interlaboratory method validation and experience. Clin Chem Lab Med 2017;55:394-402. [Crossref] [PubMed]
- Christensen RD, Agarwal AM, Nussenzveig RH, et al. Evaluating eosin-5-maleimide binding as a diagnostic test for hereditary spherocytosis in newborn infants. J Perinatol 2015;35:357-61. [Crossref] [PubMed]
- Joshi P, Aggarwal A, Jamwal M, et al. A comparative evaluation of Eosin-5'-maleimide flow cytometry reveals a high diagnostic efficacy for hereditary spherocytosis. Int J Lab Hematol 2016;38:520-6. [Crossref] [PubMed]
- King MJ, Telfer P, MacKinnon H, et al. Using the eosin-5-maleimide binding test in the differential diagnosis of hereditary spherocytosis and hereditary pyropoikilocytosis. Cytometry B Clin Cytom 2008;74:244-50. [Crossref] [PubMed]
- King MJ, Smythe JS, Mushens R. Eosin-5-maleimide binding to band 3 and Rh-related proteins forms the basis of a screening test for hereditary spherocytosis. Br J Haematol 2004;124:106-13. [Crossref] [PubMed]
- King MJ, Behrens J, Rogers C, et al. Rapid flow cytometric test for the diagnosis of membrane cytoskeleton-associated haemolytic anaemia. Br J Haematol 2000;111:924-33. [PubMed]
- Ciepiela O, Adamowicz-Salach A, Bystrzycka W, et al. Mean corpuscular volume of control red blood cells determines the interpretation of eosin-5'-maleimide (EMA) test result in infants aged less than 6 months. Ann Hematol 2015;94:1277-83. [Crossref] [PubMed]
- Agarwal AM, Liew MA, Nussenzveig RH, et al. Improved harmonization of eosin-5-maleimide binding test across different instruments and age groups. Cytometry B Clin Cytom 2016;90:512-6. [Crossref] [PubMed]
- Crisp RL, Solari L, Gammella D, et al. Use of capillary blood to diagnose hereditary spherocytosis. Pediatr Blood Cancer 2012;59:1299-301. [Crossref] [PubMed]
- Ciepiela O, Kotula I, Gorska E, et al. Delay in the measurement of eosin-5'-maleimide (EMA) binding does not affect the test result for the diagnosis of hereditary spherocytosis. Clin Chem Lab Med 2013;51:817-23. [Crossref] [PubMed]
- Girodon F, Garcon L, Bergoin E, et al. Usefulness of the eosin-5'-maleimide cytometric method as a first-line screening test for the diagnosis of hereditary spherocytosis: comparison with ektacytometry and protein electrophoresis. Br J Haematol 2008;140:468-70. [Crossref] [PubMed]
- Mayeur-Rousse C, Gentil M, Botton J, et al. Testing for hereditary spherocytosis: a French experience. Haematologica 2012;97:e48-9; author reply e52.
- Kar R, Mishra P, Pati HP. Evaluation of eosin-5-maleimide flow cytometric test in diagnosis of hereditary spherocytosis. Int J Lab Hematol 2010;32:8-16. [Crossref] [PubMed]
- Stoya G, Gruhn B, Vogelsang H, et al. Flow cytometry as a diagnostic tool for hereditary spherocytosis. Acta Haematol 2006;116:186-91. [Crossref] [PubMed]
- Shah S, Vega R. Hereditary spherocytosis. Pediatr Rev 2004;25:168-72. [Crossref] [PubMed]
- Cheli E, Roze J, Garrot T, et al. Usefulness of the EMA flow cytometric test in the diagnosis of hereditary spherocytosis post-transfusion. Br J Haematol 2017;178:180. [Crossref] [PubMed]
- Debaugnies F, Cotton F, Boutique C, et al. Erythrocyte membrane protein analysis by sodium dodecyl sulphate-capillary gel electrophoresis in the diagnosis of hereditary spherocytosis. Clin Chem Lab Med 2011;49:485-92. [Crossref] [PubMed]
- King MJ, Zanella A. Hereditary red cell membrane disorders and laboratory diagnostic testing. Int J Lab Hematol 2013;35:237-43. [Crossref] [PubMed]
- Shibuya A, Kawashima H, Tanaka M. Analysis of erythrocyte membrane proteins in patients with hereditary spherocytosis and other types of haemolytic anaemia. Hematology 2018.1-7. [Crossref] [PubMed]
- Peker S, Akar N, Demiralp DO. Proteomic identification of erythrocyte membrane protein deficiency in hereditary spherocytosis. Mol Biol Rep 2012;39:3161-7. [Crossref] [PubMed]
- Demiralp DO, Peker S, Turgut B, et al. Comprehensive identification of erythrocyte membrane protein deficiency by 2D gel electrophoresis based proteomic analysis in hereditary elliptocytosis and spherocytosis. Proteomics Clin Appl 2012;6:403-11. [Crossref] [PubMed]
- Bogusławska DM, Heger E, Listowski M, et al. A novel L1340P mutation in the ANK1 gene is associated with hereditary spherocytosis? Br J Haematol 2014;167:269-71. [Crossref] [PubMed]
- Han JH, Kim S, Jang H, et al. Identification of a novel p.Q1772X ANK1 mutation in a Korean family with hereditary spherocytosis. PLoS One 2015;10. [Crossref] [PubMed]
- Kalfa TA, Connor JA, Begtrup AH. EPB42-Related Hereditary Spherocytosis. In: Adam MP, Ardinger HH, Pagon RA, et al. editors. Seattle: GeneReviews®, 1993.
- Liu S, Jiang H, Huang LY, et al. A de novo ankyrin mutation (ANK1 Q109X) causing severe hereditary spherocytosis from preterm neonatal period. Ann Hematol 2017;96:1067-8. [Crossref] [PubMed]
- Nussenzveig RH, Christensen RD, Prchal JT, et al. Novel alpha-spectrin mutation in trans with alpha-spectrin causing severe neonatal jaundice from hereditary spherocytosis. Neonatology 2014;106:355-7. [Crossref] [PubMed]
- Wang X, Mao L, Shen N, et al. An ANK1 IVS3-2A>C mutation causes exon 4 skipping in two patients from a Chinese family with hereditary spherocytosis. Oncotarget 2017;8:113282-6. [Crossref] [PubMed]
- Bogardus H, Schulz VP, Maksimova Y, et al. Severe nondominant hereditary spherocytosis due to uniparental isodisomy at the SPTA1 locus. Haematologica 2014;99:e168-70. [Crossref] [PubMed]
- Berardi A, Lugli L, Ferrari F, et al. Kernicterus associated with hereditary spherocytosis and UGT1A1 promoter polymorphism. Biol Neonate 2006;90:243-6. [Crossref] [PubMed]
- Hassoun H, Vassiliadis JN, Murray J, et al. Characterization of the underlying molecular defect in hereditary spherocytosis associated with spectrin deficiency. Blood 1997;90:398-406. [PubMed]
- Gao Y, Zhang B, Song Y, et al. Diagnosis of Hereditary Spherocytosis and Secondary Hemochromatosis in a Patient with Jaundice. Acta Haematol 2018;139:168-70. [Crossref] [PubMed]
- Deng Z, Liao L, Yang W, et al. Misdiagnosis of two cases of hereditary spherocytosis in a family and review of published reports. Clin Chim Acta 2015;441:6-9. [Crossref] [PubMed]
- Forde DG, Cope A, Stone B. Acute parvovirus B19 infection in identical twins unmasking previously unidentified hereditary spherocytosis. BMJ Case Rep 2014;2014.