Traditional Chinese medicine Qiliqiangxin attenuates phenylephrine-induced cardiac hypertrophy via upregulating PPARγ and PGC-1α
Introduction
Cardiac hypertrophy is a great risk factor in many cardiovascular diseases including heart failure, which is an intractable disease with high morbidity and mortality worldwide (1). Although the initial stage of cardiac hypertrophy is an adaptive response to increased workload (2-4), it eventually turns into maladaptive hypertrophy and causes cardiac dysfunction, such as diastolic dysfunction along with selective reduction in substance oxidation (5). In hypertrophic myocardium, the energy resource transits from fatty acid to glucose (5,6) and the efficiency of mitochondrial metabolism is also decreased which leads to less ATP production (7,8). The possible signaling pathways or alteration in gene expression has been investigated in detail in various cardiac diseases (8-10). But there is still no available strategy to terminate or reverse the pathological process.
Peroxisome proliferator-activated receptors (PPARs) play vital roles in regulating the fatty acid and glucose metabolism. Among the PPARs, PPARγ is lowly expressed in heart but occupies a vital role in cardiac development and heart diseases (8,9,11). Besides, previous studies reported that PPARγ was downregulated during cardiac hypertrophy and the cardiomyocytes-restricted PPARγ knockout mice developed spontaneous cardiac hypertrophy (12,13). Furthermore, PPARγ activation inhibits hypertrophic signals of cardiomyocytes in response to various pathological stimuli (14).
Apart from PPARγ, its coactivator PGC-1α also regulates the energy metabolic process. PGC-1α is highly expressed in heart tissue under physiological condition, while PGC-1α is decreased and the mitochondrial function is also reduced in pressure overload-induced hypertrophy (8). PGC-1α activation increased the mitochondrial number and oxidative capacity (15) through activating PPARγ in cardiomyocytes (10). Furthermore, mice with knockdown of PGC-1α developed into cardiac dysfunction with significantly impaired mitochondrial dysfunction (9,16).
A double-blind clinical study in patients with chronic heart failure has demonstrated that Chinese medicine Qiliqiangxin (QLQX) have beneficial effects on cardiac function, exercise tolerance and patient quality of life (17). And our previous studies have showed that the QLQX attenuates cardiac hypertrophy (18). Besides, we previously demonstrated that PPARγ was crucial for the protective effects of QLQX in cardiac remodeling after myocardial infarction (19). And we have identified that QLQX treatment improved mitochondrial biogenesis and increased mitochondrial metabolism by inducing the expression of PGC-1α in H9C2 cells under physiological condition (20). However, whether QLQX prevents cardiomyocytes from cardiac hypertrophy through regulating PGC-1α/PPARγ is still unclear.
Here we used phenylephrine (PE) to induce cardiac hypertrophy, and found that QLQX could prevent the cardiac hypertrophy. Moreover, we found that PPARγ and its coactivator PGC-1α were both downregulated during cardiac hypertrophy. Ultimately, we revealed that QLQX could prevent the cardiac hypertrophy through upregulating PGC-1α and PPARγ expression.
Methods
Animal experiments
This study was conducted under the guidelines on humane use and care of laboratory animals for biomedical research published by National Institutes of Health (No. 85-23, revised 1996). All animal experiments were approved by the ethical committees of the Nanjing Medical University.
Male C57BL/6 mice aged 7–8 weeks were purchased from Beijing Vital River Laboratory Animal Technology Corporation and housed in a room with normal receiving 12-hour dark/light cycle and free access to food. Osmotic mini-pumps (model 2004; Alzet Corp, Palo Alto, CA, USA) were implanted subcutaneously at the dorsum of the neck to infuse a pressor dose of PE (40 mg/kg/d) for 3 weeks. The control mice were treated with the same procedure except their pumps were filled with vehicle. At the same time, the Chinese medicine QLQX was administrated at a dose of 0.5 g/kg/d for 3 weeks.
Echocardiography
Echocardiography was performed on mice anesthetized with 2% isoflurane using a Vevo 2100 (Visual Sonics Inc., Toronto, Ontario, Canada). The following parameters were performed: ejection fraction% (EF%), interventricular septal (IVS) and left ventricular posterior wall (LVPW).
Hematoxylin-Eosin (HE) staining
The isolated hearts were fixed with 4% paraformaldehyde and then performed the HE staining. We used the Nikon Eclipse microscope with NIS Elements software to capture the images. Image J software was used to evaluate the cross-sectional area.
Neonatal rat ventricular cardiomyocytes (NRVMs) isolation, culture and treatment
The primary NRVMs were isolated and digested as previous described (18). After the digestion, the cell suspension was centrifuged and purified by Percoll gradient centrifugation. Subsequently, the NRVMs were re-suspended in high-glucose Dulbecco’s modified Eagle’s medium (DMEM) (Gibco, Pasadena, CA, USA) with 5% fetal bovine serum (FBS) (Gibco, Pasadena, CA, USA), 10% horse serum (HS) (Gibco, Pasadena, CA, USA), 1% penicillin-streptomycin and then plated to different culture dishes.
To induce the hypertrophy, NRVMs are firstly starved for 8 h in serum-free DMEM and exposed to 50 µM PE (Sigma, St. Louis, MO, USA) for 48 h. To investigate the roles of QLQX in PE-induced cardiac hypertrophy, NRVMs were pretreated with QLQX (0.5 µg/mL) (Shijiazhuang Yiling Pharmaceutical Co., Hebei, China) for 48 h before the PE stimulation.
To investigate whether QLQX protects NRVMs from cardiac hypertrophy via PPARγ and its coactivator PGC-1α, the NRVMs were treated with QLQX together with 1 µM PPARγ agonist (Rosiglitazone) or 1 µM PPARγ inhibitor (T0070907) for 48 h, followed by the exposure to PE. Similarly, after NRVMs treated with QLQX, PGC-1α siRNAs (75 nM) were transfected using Lipofectamine 3000 (Invitrogen, MA, USA). Six to eight hours after the siRNA transfection, NRVMs were treated with 50 µM PE for the next 48 h.
Immunofluorescent staining for α-actinin and cell size analysis
The NRVMs were respectively fixed in 4% paraformaldehyde and permeabilized with 0.2% Triton X-100 for 20 min at room temperature. Then the cells were blocked with 10% goat serum in PBS for 1 h at room temperature and incubated with primary antibody α-actinin overnight (1:200) (Sigma-Aldrich, St, MO, USA). Subsequently, the cells were washed three times for 5 min each and incubated with secondary antibody for 2 h at room temperature. Finally, the cells were stained with DAPI and the images were captured by the inverted fluorescent microscope (Carl Zeiss).
Western blot analysis
The NRVMs or heart samples were lysed using RIPA buffer (Beyotime, Nantong, China), and the concentration of protein samples was evaluated by BCA protein assay kit (Beyotime, Nantong, China). Equal amounts of protein were separated in SDS-PAGE and transferred into PVDF membranes. The primary antibodies were used as follow: PPARγ (1:1,000, Cell Signaling Technology), PGC-1α (1:1,000, NOVUS), and GAPDH (1:5,000, Santacruz) was used as a loading control.
Quantitative real time PCR (qRT-PCR)
Total RNA was extracted from NRVMs or heart samples by using TRIZOL RNA extraction kit (Invitrogen). The reverse transcription was carried out using Bio-Rad iScriptTM cDNA Synthesis Kit (Bio-Rad, Hercules, CA, USA) according to the quantity (400 ng). The expression of related genes was detected by using Bio-Rad SYBR qPCR (Bio-Rad, Hercules, CA, USA) on ABI-7900 Real-Time PCR Detection System (7900HT, Applied Biosystems, CA, USA). GAPDH was used as an internal control. The primers for qRT-PCR were listed in Table 1.
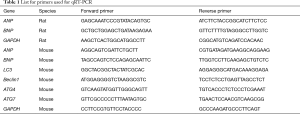
Full table
Statistical analysis
All data were expressed as mean ± SEM. The statistical significance was assessed using an independent-sample t-test for two-group comparison or one-way ANOVA for multiple-group comparison followed by Bonferroni's post hoc test. A value of P<0.05 was considered significant.
Results
QLQX attenuates cardiac hypertrophy in mice
Continuous 3-week infusion of PE caused cardiac hypertrophy in mice, revealed by the echocardiography examination (Figure 1A,B), HW/BW ratio (Figure 1C), HE staining (Figure 1D), ANP and BNP expression (Figure 1E). QLQX treatment attenuated cardiac hypertrophy and the increased expression of ANP, BNP induced by PE (Figure 1A,B,C,D,E). These data indicated that QLQX attenuates cardiac hypertrophy in PE-treated mice.
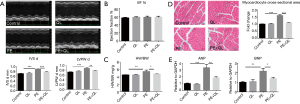
Both PPARγ and PGC1-α are decreased in PE-induced hypertrophy in NRVMs
In addition, the existing pressure overload induced by PE increases the cardiac workload, which leads to the imbalance in energy supply and demand. At present, improving the energy metabolism has been a new strategy to treat heart diseases. PPARγ and PGC-1α have been reported to be crucial for energy metabolism (21,22). Thus, we detected the expression changes during cardiac hypertrophy. NRVMs were exposed to 50 µM PE to induce cardiomyocytes hypertrophy. PE treatment increased the expression of ANP and BNP (Figure 2A), as well as cardiomyocytes size (Figure 2B). Subsequently, we detected the expression of PPARγ and PGC-1α by western blot and the results showed the decrease of PPARγ and PGC-1α during PE-induced cardiac hypertrophy (Figure 2C), indicating that PPARγ and PGC-1α might suppress cardiac hypertrophy.
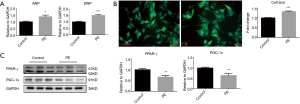
QLQX prevents cardiomyocytes hypertrophy and activates PPARγ and PGC-1α
Clinical study has proved that QLQX has a protective effect on heart failure (17). In our study, we showed QLQX protected the cardiomyocytes against the increase of ANP and BNP expression and cell size induced by PE (Figure 3A,B). Importantly, we identified that OLQX increased the expression of PPARγ and PGC-1α in NRVMs during PE stimulation (Figure 3C). Moreover, we also demonstrated that OLQX increased PPARγ and PGC-1α expression in the mice model of cardiac hypertrophy (Figure 3D). These results inspired us that PPARγ and PGC-1α might be the targets of QLQX in hypertrophic progression.
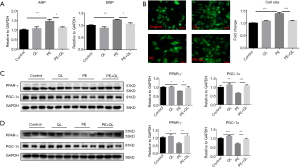
QLQX attenuates the cardiomyocytes hypertrophy via increasing PPARγ and PGC-1α expression.
To further confirm that QLQX can prevent hypertrophy via PPARγ and PGC-1α, we performed rescue experiments. Firstly, we efficiently repressed the expression of PGC-1α (Figure 4A) and found that knockdown of PGC-1α blocked the protective effects of QLQX in NRVMs stimulated by PE (Figure 4B,C,D). Secondly, we exposed the cells to PPARγ inhibitor (T0070907) or PPARγ agonist (Rosiglitazone). The protective effects of QLQX on hypertrophic cardiomyocytes were also abrogated by PPARγ inhibitors (Figure 4E,F,H). In addition, PPARγ agonist rosiglitazone did not further aggravate the protective effects of QLQX. Taken together, our results revealed that QLQX prevents cardiomyocytes hypertrophy via increasing PGC-1α and PPARγ expression.
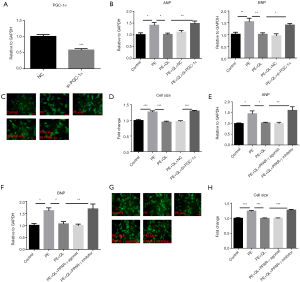
Discussion
Hypertrophy is a compensative response to increased cardiac workload, mainly resulting in cardiomegaly and increased protein content (2,4,23). Hypertrophy is classified as physiological or pathological, which are induced by different stimuli (24,25). In abnormal condition, hypertrophy induces downregulation of PGC-1α signaling and the cognate target PPARs, causing the shift of energy metabolism to resembling the fetal heart metabolism (26,27). Our study demonstrated that both PPARγ and PGC-1α were downregulated in PE-induced hypertrophy, and the Chinese medicine QLQX could attenuate cardiac hypertrophy through preventing the decrease of PPARγ and PGC-1α expression.
PGC-1α, firstly identified as a coactivator of PPARγ, serves as an activator in FA metabolism through PPARs. Activation of PGC-1α promoted the mitochondrial biogenesis and prevented the diabetic cardiomyopathy progression (28). Furthermore, the greater LV hypertrophy and dysfunction were presented in PGC-1α-/- mice versus the wild type (9,14,26). Increase of PGC1-α has been shown in QLQX-treated spontaneously hypertensive rats (29) and pressure overload heart failure rats (30). In addition, QLQX induced PGC-1α to promote the mitochondrial biogenesis and mtDNA transcription and replication in H9C2 cardiomyocytes (20). However, during PE-induced cardiomyocytes hypertrophy, the relationship between QLQX and PGC1-α is still unclear. Our results clearly showed that QLQX attenuates PE-induced cardiomyocytes hypertrophy through activating PGC1-α.
PPARγ is also a well-known crucial regulator during cardiac hypertrophy. And PPARγ can interact specifically with PGC-1α by forming a complex (8-10). It has been reported that PPARγ agonists suppress the hypertrophy of cultured NRVMs induced by mechanical stress (31). Importantly, pressure overload-induced cardiac hypertrophy in heterozygous PPARγ-deficient mice is slightly more pronounced than that in wild-type mice (13). Moreover, cardiomyocytes-specific deletion of PPARγ in mice ultimately developed into cardiac hypertrophy in mice (12). Mechanically, deletion of PPARγ increased the expression of cardiac embryonic genes (atrial natriuretic peptide and beta-myosin heavy chain), elevated the nuclear factor kappaB activity while suppressed in the heart (12). Consistent with previous studies, our work also showed that PPARγ acted as a suppressor for cardiomyocytes hypertrophy. PPARγ plays a key role in the protective effects of QLQX during cardiac remodeling after acute myocardial infarction (19,32). To date, little is known about whether PPARγ mediated the protective roles of QLQX in protecting against cardiac hypertrophy. Importantly, by performing rescue experiments, our results demonstrated for the first time that QLQX protects against PE-induced cardiac hypertrophy via increasing PPARγ.
Multiple studies have shown that QLQX attenuates pressure overload-induced cardiac hypertrophy through increasing the expression of ErbB2 and CBP/p300-interacting transactivator (33). Besides, QLQX protects against PE-induced cardiac hypertrophy via reducing microRNA-199a-5p expression (18). The important roles of PGC-1α and PPARγ have been seen in various models of cardiac hypertrophy and heart failure (10). Present study provides PGC-1α and PPARγ as novel downstream genes of QLQX in preventing from cardiac hypertrophy.
However, there are still limitations in our study. We investigated the QLQX prevented the cardiac hypertrophy by activating PPARγ and PGC-1α. Owing to the complexity in cardiac remodeling, the apoptosis, fibrosis and the oxidative stress were not taken into research though pressure overload often caused. The relationship was also not considered in detail between PPARγ and PGC-1α in our research, although their connection has been reported clearly (8-10,27,34). Based on these findings, further investigations are highly required to demonstrate the relationship between PPARγ and PGC-1α, as well as the detailed mechanisms of QLQX in preventing the hypertrophy.
Conclusions
In conclusion, the present study reveals that QLQX prevents the PE induced cardiomyocytes hypertrophy. PPARγ and PGC-1α are decreased in cardiomyocytes hypertrophy, whereas increased by QLQX treatment. PPARγ and PGC-1α activation are required for the protective roles of QLQX against cardiomyocytes hypertrophy. The novel mechanism for the protective effects of QLQX against cardiomyocytes hypertrophy sheds light on further investigation, and provides a promising prescription for the treatment of cardiac hypertrophy and dysfunction.
Acknowledgements
Funding: This work was supported by the grants from National Natural Science Foundation of China (81730106 and 81670347 to XL Li, 81770396 to HF Zhang, and 81503283 to WM Yao), National key research and development program (2017YFC1700505 and 2017YFC1700401) and the Priority Academic Program Development of Jiangsu Higher Education Institutions (PAPD20102013 to XL Li).
Footnote
Conflicts of Interest: Dr. XL received research grants from Shijiazhuang Yiling Pharmaceutical Co., Ltd. The other authors have no conflicts of interest to declare.
Ethical Statement: This study was conducted under the guidelines on humane use and care of laboratory animals for biomedical research published by National Institutes of Health (No. 85-23, revised 1996). All animal experiments were approved by the ethical committees of the Nanjing Medical University.
References
- Heineke J, Molkentin JD. Regulation of cardiac hypertrophy by intracellular signalling pathways. Nat Rev Mol Cell Biol 2006;7:589-600. [Crossref] [PubMed]
- Frey N, Olson EN. Cardiac hypertrophy: the good, the bad, and the ugly. Annu Rev Physiol 2003;65:45-79. [Crossref] [PubMed]
- Bei Y, Xu T, Lv D, et al. Exercise-induced circulating extracellular vesicles protect against cardiac ischemia-reperfusion injury. Basic Res Cardiol 2017;112:38. [Crossref] [PubMed]
- Shi J, Bei Y, Kong X, et al. miR-17-3p contributes to exercise-induced cardiac growth and protects against myocardial ischemia-reperfusion injury. Theranostics 2017;7:664-76. [Crossref] [PubMed]
- Mori J, Basu R, McLean BA, et al. Agonist-induced hypertrophy and diastolic dysfunction are associated with selective reduction in glucose oxidation: a metabolic contribution to heart failure with normal ejection fraction. Circ Heart Fail 2012;5:493-503. [Crossref] [PubMed]
- Santalucía T, Sánchez-Feutrie M, Felkin LE, et al. Phenylephrine requires the TATA box to activate transcription of GLUT1 in neonatal rat cardiac myocytes. J Mol Cell Cardiol 2005;38:677-84. [Crossref] [PubMed]
- Stuck BJ, Lenski M, Böhm M, et al. Metabolic switch and hypertrophy of cardiomyocytes following treatment with angiotensin II are prevented by AMP-activated protein kinase. J Biol Chem 2008;283:32562-9. [Crossref] [PubMed]
- Huss JM, Kelly DP. Nuclear receptor signaling and cardiac energetics. Circ Res 2004;95:568-78. [Crossref] [PubMed]
- Rowe GC, Jiang A, Arany Z. PGC-1 coactivators in cardiac development and disease. Circ Res 2010;107:825-38. [Crossref] [PubMed]
- Riehle C, Abel ED. PGC-1 proteins and heart failure. Trends Cardiovasc Med 2012;22:98-105. [Crossref] [PubMed]
- Wang YX. PPARs: diverse regulators in energy metabolism and metabolic diseases. Cell Res 2010;20:124-37. [Crossref] [PubMed]
- Duan SZ, Ivashchenko CY, Russell MW, et al. Cardiomyocyte-specific knockout and agonist of peroxisome proliferator-activated receptor-gamma both induce cardiac hypertrophy in mice. Circ Res 2005;97:372-9. [Crossref] [PubMed]
- Asakawa M, Takano H, Nagai T, et al. Peroxisome proliferator-activated receptor gamma plays a critical role in inhibition of cardiac hypertrophy in vitro and in vivo. Circulation 2002;105:1240-6. [Crossref] [PubMed]
- Wang J, Song Y, Zhang Y, et al. Cardiomyocyte overexpression of miR-27b induces cardiac hypertrophy and dysfunction in mice. Cell Res 2012;22:516-27. [Crossref] [PubMed]
- LaGory EL, Wu C, Taniguchi CM, et al. Suppression of PGC-1α Is Critical for Reprogramming Oxidative Metabolism in Renal Cell Carcinoma. Cell Rep 2015;12:116-27. [Crossref] [PubMed]
- Xu L, Ma X, Bagattin A, et al. The transcriptional coactivator PGC1α protects against hyperthermic stress via cooperation with the heat shock factor HSF1. Cell Death Dis 2016;7:e2102. [Crossref] [PubMed]
- Li X, Zhang J, Huang J, et al. A multicenter, randomized, double-blind, parallel-group, placebo-controlled study of the effects of qili qiangxin capsules in patients with chronic heart failure. J Am Coll Cardiol 2013;62:1065-72. [Crossref] [PubMed]
- Zhang H, Li S, Zhou Q, et al. Qiliqiangxin Attenuates Phenylephrine-Induced Cardiac Hypertrophy through Downregulation of MiR-199a-5p. Cell Physiol Biochem 2016;38:1743-51. [Crossref] [PubMed]
- Shen S, Jiang H, Bei Y, et al. Qiliqiangxin Attenuates Adverse Cardiac Remodeling after Myocardial Infarction in Ovariectomized Mice via Activation of PPARγ. Cell Physiol Biochem 2017;42:876-88. [Crossref] [PubMed]
- Lin S, Wu X, Tao L, et al. The Metabolic Effects of Traditional Chinese Medication Qiliqiangxin on H9C2 Cardiomyocytes. Cell Physiol Biochem 2015;37:2246-56. [Crossref] [PubMed]
- Song MY, Jung HW, Kang SY, et al. Atractylenolide III Enhances Energy Metabolism by Increasing the SIRT-1 and PGC1α Expression with AMPK Phosphorylation in C2C12 Mouse Skeletal Muscle Cells. Biol Pharm Bull 2017;40:339-44. [Crossref] [PubMed]
- Qiang L, Wang L, Kon N, et al. Brown remodeling of white adipose tissue by SirT1-dependent deacetylation of Pparγ. Cell 2012;150:620-32. [Crossref] [PubMed]
- Shioi T, Kang PM, Douglas PS, et al. The conserved phosphoinositide 3-kinase pathway determines heart size in mice. EMBO J 2000;19:2537-48. [Crossref] [PubMed]
- Liu X, Xiao J, Zhu H, et al. miR-222 is necessary for exercise-induced cardiac growth and protects against pathological cardiac remodeling. Cell Metab 2015;21:584-95. [Crossref] [PubMed]
- van Berlo JH, Elrod JW, Aronow BJ, et al. Serine 105 phosphorylation of transcription factor GATA4 is necessary for stress-induced cardiac hypertrophy in vivo. Proc Natl Acad Sci U S A 2011;108:12331-6. [Crossref] [PubMed]
- Lu Z, Xu X, Hu X, et al. PGC-1 alpha regulates expression of myocardial mitochondrial antioxidants and myocardial oxidative stress after chronic systolic overload. Antioxid Redox Signal 2010;13:1011-22. [Crossref] [PubMed]
- Zhang Y, Ba Y, Liu C, et al. PGC-1alpha induces apoptosis in human epithelial ovarian cancer cells through a PPARgamma-dependent pathway. Cell Res 2007;17:363-73. [Crossref] [PubMed]
- Wang H, Bei Y, Lu Y, et al. Exercise Prevents Cardiac Injury and Improves Mitochondrial Biogenesis in Advanced Diabetic Cardiomyopathy with PGC-1α and Akt Activation. Cell Physiol Biochem 2015;35:2159-68. [Crossref] [PubMed]
- Wang J, Li Z, Wang Y, et al. Qiliqiangxin Enhances Cardiac Glucose Metabolism and Improves Diastolic Function in Spontaneously Hypertensive Rats. Evid Based Complement Alternat Med 2017;2017:3197320. [PubMed]
- Zhang J, Wei C, Wang H, et al. Protective effect of qiliqiangxin capsule on energy metabolism and myocardial mitochondria in pressure overload heart failure rats. Evid Based Complement Alternat Med 2013;2013:378298. [PubMed]
- Yamamoto K, Ohki R, Lee RT, et al. Peroxisome proliferator-activated receptor gamma activators inhibit cardiac hypertrophy in cardiac myocytes. Circulation 2001;104:1670-5. [Crossref] [PubMed]
- Tao L, Shen S, Fu S, et al. Traditional Chinese Medication Qiliqiangxin attenuates cardiac remodeling after acute myocardial infarction in mice. Sci Rep 2015;5:8374. [Crossref] [PubMed]
- Zou Y, Lin L, Ye Y, et al. Qiliqiangxin inhibits the development of cardiac hypertrophy, remodeling, and dysfunction during 4 weeks of pressure overload in mice. J Cardiovasc Pharmacol 2012;59:268-80. [Crossref] [PubMed]
- Butterick TA, Hocum Stone L, Duffy C, et al. Pioglitazone increases PGC1-α signaling within chronically ischemic myocardium. Basic Res Cardiol 2016;111:37. [Crossref] [PubMed]