Prediction models to advance individualized resuscitation in trauma hemorrhage and acute traumatic coagulopathy (ATC): even the longest journey starts with first steps—Lao-Tzu (Chinese philosopher)
Uncontrolled hemorrhage together with acute traumatic coagulopathy (ATC) are still the principle causes for preventable death following severe injury with one out of four patients admitted to the hospital bleeding with variable signs of laboratory coagulopathy (1-4). Deterioration and death under these conditions usually occur early within 6–8 hours after injury with mortality rates from massive transfusion up to 70 percent even in advanced trauma facilities (5). Meanwhile, ATC is recognized as an own clinical entity with considerable impact on both morbidity and mortality after injury (6). There has been much debate about the potential mechanisms underlying ATC over the past decade but much of the data continues to be rather correlative than causative with robust links and support for diagnosis, prediction and clinical decision making still lacking (7).
ATC is complex and still incompletely characterized: research priority!
As consequence, current resuscitation practices center around non-targeted approaches to restore the coagulation cascade including its effector protein thrombin via administration of poorly characterized fresh frozen plasma (FFP) concentrates, conventional blood products [packed red blood cell (pRBC)] concentrates and platelet concentrates either in large quantities or empiric predefined ratios (8,9) (Figure 1). These approaches, on one hand side, consider early and aggressive management to reduce mortality but, one the other hand side, at least in part, ignore the risk of both over- and undertreatment contributing to morbidity and poorer outcomes in patients with hemorrhagic shock (10). Optimum ratios are still not defined, no universal standards for these transfusion packages have yet been established and longer storage periods also considerably affect the hemostatic power of these products. On the functional level, these blunt approaches may also not be sufficient to correct either hypoperfusion or ATC during the acute phase of trauma hemorrhage (11,12). What further adds to uncertainty in the treatment of bleeding trauma patients and ATC is our blunt diagnostic arsenal for clinical decision support which likewise suffers from absence of rapid accessible and patient-specific coagulation measurements. In the era in which precision medicine and individualized therapies gain more and more importance, first steps in this direction have been undertaken with some beneficial effect but no consensus has yet been established on types and amounts of coagulation factor supplementation (9). The identification and characterization of the different phenotypes and mechanisms of ATC, their clinical manifestations and how they can be rapidly identified have been defined as top priorities for trauma critical care research in the next 10 years (13).
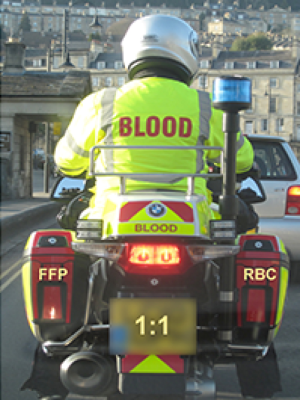
First steps towards targeted coagulation control through a thrombin dynamics model
In their fine work, Menezes and co-workers, initiate first steps in the direction of future targeted interventions that consider control of trauma coagulation in vivo thereby enabling the supplementation of blood coagulation factors in model-determined concentrations as effective clinical intervention to therapeutically tackle ATC (14). As an alternative to current and yet blunt treatment approaches, laboratory tests with long turn-around times, and purely mechanistic model simulations, this group suggests a simple and biologically meaningful model that, on one hand side, rapidly forecasts the principle driver of downstream coagulation, e.g., thrombin concentration after tissue factor (TF) stimulation, by considering timely concentration changes of blood protein factors, and, on the other hand side, assesses the quantity of additional coagulation factors necessary to correct the predicted thrombin dynamics. Computational models may serve as mediators between biological coagulation kinetics and individual molecular and physiological measures to promote a mechanistic idea of blood clotting in trauma and thereby to better inform innovative treatment approaches. To date, existing models still suffer from substantial limitations, as they are not respecting the entire spectrum of the yet identified physiology, nor is there any guidance on how to tailor their ingredients to the individual needs of the bleeding trauma patient. More complex models attempting to capture larger numbers of physiological parameters affecting coagulation become subsequently very large in size which limits their clinical applicability due to increased simulation duration, complexity and uncertainty (15).
Model based upon the calibrated automated thrombogram (CAT) assay
The model suggested by Menezes and co-workers is based upon the so-called CAT, an assay to assess the dynamical system of thrombin in a fashion to be useful for control-theoretic analysis. The thrombogram assesses the concentration of thrombin in clotting plasma and represents, therefore, a general function test of the thrombotic hemostatic system. The CAT test measures the time span of thrombin generation in blood, for example in a blood sample from a bleeding trauma patient with presumed ATC, exposed to additionally added TF (16). Why is thrombin measurement a promising target? It is considered as the end-product of the coagulation process responsible for the conversion of fibrinogen into stable fibrin to form the clot. The multitude of (nested) feedback loops within the coagulation system all either directly or indirectly affected by the emerging thrombin concentration makes this protein in amount and temporal pattern an attractive target to assess the full picture of coagulability in both directions, hyper- and hypocoagulability. But as with other tests, a major drawback of the assay is still its duration and even early termination after 10 minutes is not quick enough to assist the treating clinician in decision making in the rapidly changing environment of acute and bleeding trauma care. As the CAT assay is also restricted to the end-product it does not provide information about specific supplementation targets in the context of patient-specific therapies and, in combination with other variables and experience, can only serve as an educated guess with respect to potential future clotting behaviour. But the idea behind the model presented was to create an in-silico dynamical system that timely and adequately mimics CAT thrombin concentration output from rapid quantification of blood protein factor concentrations which may increase CAT’s current clinical meaning by delivering model-based dynamic information.
A multiple step development of the model
Blood samples with protein factors within reference ranges and from severely injured trauma patients treated in a single US trauma center were used to assess the thrombin dynamical system for model determination. Increasing individual protein concentrations and assessing the corresponding CATs informed on the effect of protein factor “actuators” in controlling coagulation. At the end, the model accurately predicted CAT dynamics from initial protein factor concentrations which ultimately led to the question of desirable dynamic changes by adding selected factors to the sample to achieve a CAT trajectory including prediction. In this context, model prediction translates into control of the thrombin dynamical system which is presented in two steps. First, increasing factor concentrations in samples proves an accurate model prediction of changed dynamics which actually serves as model validation. Second, the prediction of concentration additions that achieves a desirable coagulation outcome taking the linearity of the model’s equation into account. The patient-specific model, as it finally stands, includes the unique dynamics of thrombin generation through a single-input, e.g., TF concentration, single output, e.g., thrombin concentration, linear time-invariant dynamical system, which implies dynamics that are related to quickly accessible blood protein factor concentrations in both normal and trauma patients. This control-orientated dynamical model to trauma coagulation, if further refined and validated, truely holds potential to assist individualized resuscitation in the context of clinical trauma care with substantial bleeding and ATC.
Caveats need to be considered
Menezes and co-workers are congratulated for their fine work which is definitively following a promising avenue of opportunity and which should be further persued and explored. However, a number of caveats need to be considered in context to the presented model. The authors claim the tuning effect of the thrombin dynamical system with added coagulation factor concentrations. But in fact, only three factors, e.g., factors II, VIII, and X, were tested due to “practical reasons” leaving the potential effects of factor concentrations V, VII, IX in this model scarce. This is noteworthy as rFVIIa is known to initiate thrombin formation by binding with exposed TF (17). This also leaves the overall control authority of thrombin tuning range and capability based upon the full spectrum of coagulation factor concentrations yet to be identified. There also appears to exist an age-dependancy of thrombin generation by means of CAT measurement which has not been addressed at all in the present model (18). Altogether, the given approach is highly worthwhile but should be considered as a successful “proof-of-concept” in the absence of any guidelines for either desirable CAT trajectories or sequences to be achieved via targeted coagulation control. The delicate balance between hyper- and hypocoagulation needs further attention in order to avoid detrimental effects potentially arising from prothrombotic states leading to ischemia and infarction. Last but not least and as with all in vitro models in general, their translation into the clinical arena including validation and confirmation for the in vitro readout to mean a real benefit for the bleeding trauma patient with ATC remains a challenge.
Acknowledgements
None.
Footnote
Conflicts of Interest: M Maegele has received honoraria from Astra Zeneca, CSL Behring, LFB Biomedicaments France, TEM International, IL Werfen.
References
- Evans JA, Van Wessem KJ, McDougall D, et al. Epidemiology of traumatic deaths: comprehensive population-based assessment. World J Surg 2010;34:158-63. [Crossref] [PubMed]
- Schoeneberg C, Schilling M, Hussmann B, et al. Preventable and potentially preventable deaths in severely injured patients: a retrospective analysis including patterns of errors. Eur J Trauma Emerg Surg 2017;43:481-9. [Crossref] [PubMed]
- Brohi K, Singh J, Heron M, et al. Acute traumatic coagulopathy. J Trauma 2003;54:1127-30. [Crossref] [PubMed]
- Maegele M, Lefering R, Yucel N, et al. Early coagulopathy in multiple injury: an analysis from the German Trauma Registry on 8724 patients. Injury 2007;38:298-304. [Crossref] [PubMed]
- Sihler KC, Napolitano LM. Massive transfusion: new insights. Chest 2009;136:1654-67. [Crossref] [PubMed]
- S3 Guideline on Treatment of Patients with Severe and Multiple Injuries, English Version of the German Guideline S3 Leitlinie Polytrauma/Schwerverletzten-Behandlung AWMF Register-Nr. 012/019. Available online: https://zh.scribd.com/document/331611940/German-Guidelines-S3-Severe-and-Multiple-Injuries-2012-11
- Chang R, Cardenas JC, Wade CE, et al. Advances in the understanding of trauma-induced coagulopathy. Blood 2016;128:1043-9. [Crossref] [PubMed]
- Rossaint R, Bouillon B, Cerny V, et al. The European guideline on management of major bleeding and coagulopathy following trauma: fourth edition. Crit Care 2016;20:100.
- Briggs A, Askari R. Damage control resuscitation. Int J Surg 2016;33:218-21. [Crossref] [PubMed]
- Johnson JL, Moore EE, Kashuk JL, et al. Effect of blood product transfusion on the development of postinjury multiple organ failure. Arch Surg 2010;145:973-7. [Crossref] [PubMed]
- Khan S, Brohi K, Chana M, et al. Hemostatic resuscitation is neither hemostatic nor resuscitative in trauma hemorrhage. J Trauma Acute Care Surg 2014;76:561-7. [Crossref] [PubMed]
- Khan S, Davenport R, Raza I, et al. Damage control resuscitation using blood component therapy in standard doses has a limited effect on coagulopathy during trauma hemorrhage. Intensive Care Med 2015;41:239-47. [Crossref] [PubMed]
- Asehnoune K, Balogh Z, Citerio G, et al. The research agenda for trauma critical care. Intensive Care Med 2017. [Epub ahead of print]. [Crossref] [PubMed]
- Menezes AA, Vilardi RF, Arkin AP, et al. Targeted clinical control of trauma coagulation through a thrombin dynamics model. Sci Transl Med 2017;9:eaaf5045. [Crossref] [PubMed]
- Luan D, Szlam F, Tanaka KA, et al. Ensembles of uncertain mathematical models can identify network response to therapeutic intervention. Mol Biosyst 2010;6:2272-86. [Crossref] [PubMed]
- Hemker HC, Giesen P, AlDieri R, et al. The calibrated automated thrombogram (CAT): a universal routine test for hyper- and hypocoagulability. Pathophysiol Haemost Thromb 2002;32:249-53. [Crossref] [PubMed]
- Aljamali MN, Kjalke M, Hedner U, et al. Thrombin generation and platelet activation induced by rFVIIa (NovoSeven) and NN1731 in a reconstituted cell-based model mimicking haemophilia conditions. Haemophilia 2009;15:1318-26. [Crossref] [PubMed]
- Haindl H, Cimenti C, Leschnik B, et al. Age-dependency of thrombin generation measured by means of calibrated automated thrombography (CAT). Thromb Haemost 2006;95:772-5. [PubMed]