Overcoming resistance to BRAF inhibitors
Introduction
Before the discovery of activating mutations in the BRAF gene in melanoma, metastatic melanoma was considered a cancer with the direst prognosis. Classical chemotherapy regimens and the prodrug dacarbazine provided little therapeutic opportunities for clinicians trying to manage this deadly form of skin cancer. In 2002, a seminal study performed by the Cancer Genome Project at the Sanger Institute identified BRAF mutations in over 60% of melanomas (1). This groundbreaking discovery initiated great scientific efforts to dissect the role of BRAF and the MAPK-pathway in melanoma initiation and progression. Today we know that BRAF is mutated in around 50% of melanomas (2) and, by activating the MAPK-pathway, is the main driver of melanoma development. The substitution of a valine for a glutamic acid at position 600 is the most common mutation found in BRAF (2,3). BRAFV600E strongly activates the MAPK-pathway in melanocytes in culture (4), where it eventually induces senescence (5). Intriguingly, this mutation is also found in up to 80% of human benign moles or nevi (6), which are considered senescent clones of melanocytes. In conjunction with INK4A inactivation BRAFV600E transforms melanocytes in vitro (4). Targeted expression of BRAFV600E in the melanocyte lineage in vivo induces melanoma formation, and its onset can be accelerated by INK4 or PTEN deficiency or concomitant UV exposure (7-9).
While the detection of BRAF mutations majorly influenced the approach to melanoma therapy, the recognition of the importance of the MAPK-pathway for melanoma biology actually preceded the discovery of BRAF as an oncogene. Indeed, studies into cell signaling in a fish model for melanoma (10,11) and the use of early-stage MEK inhibitors such as PD908059 and U0126 (12,13) demonstrated the early activation of the MAPK-pathway during melanoma development in vivo, as well as its relevance for melanoma cell proliferation and its potential as therapeutic target. In 2002, Cohen et al. reported constitutive ERK-phosphorylation in >20% of benign nevi and >80% of primary melanoma (14), and hence confirmed activation of MAPK-signaling as an early event in human melanoma development. Pre-clinical studies developed over the following 15 years have underscored the importance of the BRAF-MAPK pathway in a multitude of processes involved in melanoma development and progression and BRAFV600E has been established as central for the control of melanoma cell proliferation and cell cycle progression, apoptosis, migration, invasion, glucose metabolism, adaptation to hypoxia or angiogenesis (3,15-22). Indeed it has now become well accepted that melanomas harboring BRAF mutations are addicted to the MAPK-pathway, as BRAFV600E cells show significant sensibility towards either genetic or chemical inhibition of this pathway.
BRAF inhibitors as single agent
A few years after the seminal study by the Sanger institute the serine/threonine (S/T) kinase inhibitor sorafenib (BAY 43-9006), which possesses activity against CRAF (23) was trialed in solid tumors including melanoma (24). Dose limiting toxicity and probably due to its low specificity towards mutant BRAF, limited clinical effect, and led to sorafenib being discarded as an anti-melanoma treatment although its application has shown certain success against other neoplastic diseases such as renal cancer (25,26). The first drug developed to specifically target BRAFV600E was Vemurafenib (PLX4032). Vemurafenib is a small molecule reversible inhibitor with specific affinity for the ATP-binding pocket of the constitutively active mutant form of BRAFV600E, where it binds to the active site of the kinase domain in its “DGF-in” conformation to block access to ATP (27,28). In early phase I and II trials (BRIM-1 and BRIM-2) vemurafenib showed unprecedented clinical responses (56% and 53%, respectively) and complete response in up to 6% of cases with 15.9 months of median overall survival (OS) and a median progression-free survival (PFS) of 6.7 months (29,30). BRIM-3, a phase III trial of vemurafenib extended to patients with mutations V600E, V600K and V600D demonstrated a median OS and PFS of 13.6 and 6.9 months respectively (31). More recently another potent inhibitor against mutated BRAF, Dabrafenib (GSK2118436), was shown to mirror the effects observed with vemurafenib (32). The unprecedented efficacy of these drugs changed the way clinicians managed melanoma patients, but soon the long-term suitability of these drugs to treat melanomas harboring activating mutations in the BRAF gene was questioned. Apart from the appearance of a wide range of adverse effects, the more concerning fact was the appearance of skin-related effects within the first 3 months that included the development of squamous cell carcinomas and keratoacanthomas in 10–20% of melanoma patients treated with BRAF inhibitors (29-31,33-35). These secondary cancers were subsequently found to be induced by the “paradoxical activation” of CRAF by BRAF inhibitors in pre-existing keratinocytic lesions harboring wild-type BRAF, but activated RAS (36,37). While clinically manageable, these discoveries prompted further studies, which found that indeed, BRAF inhibition can accelerate the development of pre-existing RAS mutant malignancies including NRAS mutant leukemias or K-RAS mutant pancreatic or colon adenocarcinomas (38-41).
Despite great initial responses the main problem regarding the use of BRAF inhibitors remains the fact they are short lived because within a year the majority of patients no longer respond to treatment and relapse (31,42). In addition, ~20% of patients harboring activating mutations in BRAF present intrinsic resistance and do not respond to BRAF inhibitors (30,31,43), which limits the effectiveness of this therapeutic approach.
Mechanisms of resistance to BRAF inhibitors
Work into understanding the mechanisms by which melanoma cells evade BRAF inhibitor therapies revealed that the majority of resistances are centered around the reactivation of the MAPK-pathway (Figure 1), which further highlights the central role played by this signaling cascade in BRAF mutated melanomas. Alternatively, mechanisms of escaping BRAF inhibitor induced cytotoxicity have been described that are independent of re-activation of the MAPK-pathway (Figure 1).
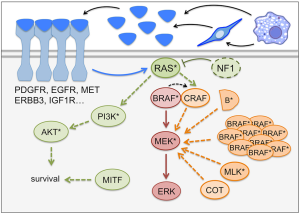
MAPK pathway reactivation
The detailed analysis of melanomas from relapsed patients found that in 70% of cases a plethora of different mechanisms allow melanoma cells to bypass BRAF inhibition by maintaining MEK activation in a BRAF independent manner, which will ultimately restore ERK dependent signaling (44,45).
Upstream of BRAF the overexpression of NRAS, activating mutations in the NRAS protein or loss of the GTPase-activating protein NF1 leads to BRAF independent MEK activation (Figure 1) (46,47). Furthermore, increased activity of MEK emanating from several receptor tyrosine kinases (RTKs) such as EGFR, IGF-1R, or PDGFR has also been found to be present in relapsed melanomas (Figure 1) (46,48,49). At the level of the RAF kinases, CRAF overexpression, BRAF gene amplification and the presence of BRAF truncations (as a consequence of alternative splicing) permit maintenance of ERK activation (50,51), while downstream of BRAF, overexpression or mutation of MEK itself or its activators, COT/TPL2/MAP3K8 or MLKs, have also been described to reactivate the pathway (Figure 1) (52-57).
MAPK-reactivation independent mechanisms of resistance to BRAF inhibitors
Apart from reactivation of ERK through the RAS-CRAF-MEK-ERK route, RAS dependent parallel activation of the PI3K/AKT pathway downstream of IGF-R or MET receptors can also contribute to limit cytotoxicity resulting from RAF inhibition (49,58). Moreover mutations in this pathway, notably activating mutations in AKT and loss of function mutations in PTEN (observed in 6–7% of melanomas and leading to PI3K activation) have been described in melanomas resistant to BRAF inhibitors (Figure 1) (44,59). Upon BRAF inhibitor treatment, ERBB3 receptor hyper-phosphorylation also promotes survival of BRAF mutated melanoma in an AKT dependent manner (60,61). Indeed the activation of the PI3K pathway seems to act as a cell death-evading mechanism through regulation of expression of apoptosis regulators such as BCL2 or BIM (58,62,63).
Another regulator of anti-apoptotic survival genes such as BCL2A1 and BCL2 is MITF, the master regulator of melanocytes and melanoma biology (17,64). High MITF levels allow melanoma cells to evade cell death triggered by BRAF (and MEK) inhibitors even when the MAPK pathway appears fully blocked (65,66). Accordingly, MITF gene amplification is found in a small percentage of progressed melanomas (45). Furthermore, MITF is upregulated by BRAF/MEK-inhibitor treatments through a MAPK-dependent re-wiring of the transcriptional control of MITF expression (67). This rewiring appears to happen in the initial phases of patient treatment, because ~80% of melanomas show a significant induction of MITF expression, and this allows melanoma cells to enter a drug-tolerance phase in the early steps of MAPK inhibitor treatment (67).
Another type of resistance is linked to increased RTK signaling, which is correlated with high expression of the RTK AXL (65,68). While RTK dependent melanoma cells are less frequently found before treatment with BRAF inhibitors, high expression of AXL is observed in ~50% of relapsed melanomas (69). Intriguingly, high AXL expression in melanoma cells is accompanied with low expression of MITF thus defining an AXLhigh/MITFlow phenotype. This AXLhigh/MITFlowphenotype does not only display innate resistance to BRAF inhibitors and increased invasiveness, but also represents a more de-dedifferentiated state (65). Thus the role of MITF in the response of melanoma cells to MAPK inhibitors is complex: the presence of MITF is a marker for responsiveness to treatment, but when MITF expression is upregulated, it confers resistance to MAPK inhibitors. On the other hand very low levels of MITF when co-existing with high levels of the RTK AXL protect melanoma cells from BRAF inhibitor induced cytotoxicity. Taken together it seems that the responsiveness of melanoma cells to BRAF inhibitors lies between two different “MITF-states” with the plasticity of tumors cells to switch from one state to the other being a determining factor to adapt to MAPK pathway inhibitors (64). It would be of interest to assess in patient samples whether the relative proportion of AXLhigh/MITFlow and AXLlow/MITFhigh might have predictive value towards the determination of patients responsiveness to BRAF inhibitor based therapies.
Non-cell autonomous derived resistance to BRAF inhibitors
Tumors are heterogeneous and not only harbor cancer cells, but also stromal cells that can both positively and negatively impact on tumor growth, metastatic potential and importantly, on response to therapies. As such fibroblasts can protect melanoma cells from BRAF inhibition. Thereby, Hepatocyte Growth Factor (HGF) secreted from stromal fibroblast can restore ERK activity through CRAF by stimulating the MET tyrosine kinase receptor (58). Tumor associated fibroblasts can also provide resistance by modifying the extracellular matrix (ECM), which induces integrin mediated Focal Adhesion kinase activation (70). Apart from fibroblasts, macrophages by secreting Tumor Necrosis Factor, can activate NFkB signaling in melanoma cells to upregulate the expression of MITF and allow melanoma cells to evade MAPK inhibitor induced cytotoxicity (71). Macrophages have also been shown to produce VEGF, which can reactivate ERK in the presence of BRAF inhibitors (72). Macrophages and fibroblasts can also act together in “inflammatory niches”, whereby macrophages secret IL1, which stimulates the production of GRO by fibroblasts. GRO activates CXCR2 signaling in melanoma cells, which overcomes BRAF inhibition as well as BRAF/MEK combination therapies (73). BRAF inhibition shows also efficacy in brain metastases (74), but it has been suggested that ERK- and PI3K-activating extrinsic factors contained in cerebrospinal fluid might contribute to BRAF inhibitor resistance in this setting (75). Finally, upon BRAF inhibitor treatment, specific soluble factors can sustain the proliferation of innate resistant cells that normally present as slow cycling cells in therapy naïve tumors (76,77).
The observed side effects and most prominently, the plethora of intrinsic/innate and acquired resistance mechanisms described above limit the efficacy of BRAF inhibitor based therapies in melanoma patients.
Combinatorial BRAF and MEK inhibitor therapies
Prior and during the development of BRAF inhibitors, MEK, acknowledged as the only effector of BRAF had attracted interest as therapeutic target. Soon it was realized that melanomas harboring mutations in BRAF where significantly more sensitive to MEK inhibition than melanomas expressing mutant NRAS, which resulted in PI3K activation providing pro-survival signals (78,79). Indeed MEK inhibitors such as selumetinib (AZD6244), binimetinib (MEK162) or trametinib have shown clinical efficacy as single agents in melanomas with BRAF mutations (80-85). Nevertheless insufficient efficacy has hindered the application of MEK inhibitors as single agents. On the other hand the combination of BRAF and MEK inhibitors has shown great success in BRAF mutant patients and as a result, between 2014 and 2015 the FDA approved the use of trametinib/dabrafenib and cobimetinib/vemurafenib as standard of care for BRAF-mutant advanced melanoma. In these trials it was seen that in patients who previously developed resistance to dabrafenib the follow-up treatment with a dabrafenib/trametinib showed limited clinical efficacy with an overall response rate (ORR) of around 10% and modest increases in OS and PFS, possibly dependent on the duration of previous BRAF inhibitor exposure (86). On the other hand, in BRAF inhibitor naïve patients BRAF/MEK-inhibitor combinations increase both overall OS and PFS when compared with the clinical effect of BRAF inhibitors only. Indeed, the cobimetinib/vemurafenib combination increased median OS, which at the final analysis was 22.3 months (95% CI, 20.3–not estimable) for cobimetinib and vemurafenib versus 17.4 months (95% CI, 15.0–19.8) for placebo and vemurafenib (HR 0.70, 95% CI, 0.55–0.90; P=0.005) (87-89). Similar improvements were also observed in patients treated with trametinib/dabrafenib, and treatment with dabrafenib 150 mg twice daily plus trametinib 2 mg daily achieved a median OS of 27.4 months (95% CI, 12.9 to not reached), with an OS at 1, 2, and 3 years of 72%, 60%, and 47%, respectively (42,90,91). Despite slight differences on clinical endpoints both combinations provide equally evident benefit, and as previously mentioned have now become the standard of care for BRAF mutant melanomas (92,93). Combinations of BRAF and MEK targeting drugs provide further clinical benefits as the occurrence of cutaneous adverse events such as squamous cell carcinoma or keratoacanthoma is significantly diminished (94), positively impacting on patient’s health-related quality of life (95,96).
Drug tolerance, acquired resistance and scheduling
Despite the immediate and, sometimes, long-lasting results achieved with combinatorial targeted therapies (42,88,97), most patients treated with combination therapies still relapse due to the development of resistance mechanism similar to those described above. The appearance of BRAF amplifications, BRAF splice variants and mutations in MEK1/2 have been described as mechanisms of cross-resistance to both mono and combination therapy that involve reactivation of the MAPK-pathway (98,99), while MITF overexpression, known to confer resistance to both BRAF and MEK inhibitors is found in ~80% of patients on treatment with BRAF/MEK-inhibitor (67). A common view of the phenomenon of acquired resistance is that initially the presence of BRAF and MEK inhibitors induces a rewiring of the MAPK pathway allowing the tumors to develop drug tolerance (100,101). Following on from this, the responsiveness of the tumor to the kinase inhibitors eventually changes towards a permanent resistance program through either the selection for mutant clones or the establishment of new mutations in response to the signaling pressure exerted by the BRAF/MEK-inhibitor mediated MAPK blockade.
Recent studies suggest that by modulating the schedule of inhibitor administration in a way that prevents MAPK-pathway rewiring tumor responsiveness to BRAF and MEK inhibitors over time is maintained. Pre-clinical studies propose an on-off schedule or drug-holiday to break the rewiring of the MAPK pathway (102,103), and this concept has been taken on board in the currently ongoing study GEM015 (https://www.clinicaltrialsregister.eu/ctr-search/trial/2014-005277-36/ES). The theory of a long-term on-off scheduling is further supported by the exciting outcome of a phase II trial in patients that had progressed on BRAF inhibitor and had been taken off drugs for at least 12 weeks. Thirty percent of these patients had partial responses and 40% continued with stable disease (104). Intriguingly, while off BRAF inhibitors, all the patients in the trial had been treated with immunotherapies but had progressed, suggesting the potential of combining an on-off BRAF/MEK-inhibitor treatment with anti-PD-1 or PD-L1 antibodies (104).
Potential for alternative combination therapies
As previously mentioned ~70% of mechanisms of resistance involve reactivation of the MAPK pathway and of these ~37% occur in a RAS-dependent manner (44). In addition, over 20% of patients relapse with activated PI3K signaling, be it through RAS or mutations in the PI3K pathway (44,45). Thus, in progressed tumors MAPK pathway activation is dependent on CRAF and not BRAF, and PI3K signaling is activated and provides pro-survival signals. With this in mind researchers have explored several approaches to prevent the development of resistance and/or provide therapeutic alternatives to progressed patients.
While in the post-sorafenib era the main goal had been to gain specificity towards mutated BRAF lately some effort has been put towards the development of small molecules directed to both BRAF and CRAF and capable to interfere with isoform hetero- and homo-dimerization thus preventing paradoxical activation of CRAF by BRAF inhibitors (72,105-110). While this approach has provided promising results in pre-clinical models, their efficacy and the risk of adverse events will have to be tested in early phase trials (NCT02607813, www.clinicaltrials.gov). Another possibility to target RAF kinases is by means of interfering with the protein stability by using chaperone inhibitors. Heat Shock Protein 90 (HSP-90) regulates the stability and degradation of both BRAF V600E and CRAF (111,112) and HSP-90 inhibitors have been postulated as candidates to prevent BRAF inhibitor resistance (56,113,114). At present several trials are underway, which assess these compounds in combination with BRAF and MEK inhibitors in melanoma patients (NCT02097225, NCT02721459, NCT01657591, www.clinicaltrials.gov).
In line with the view of developing multi-targeted combination treatments there is a growing number of pre-clinical and even clinical studies underway testing the potential of broad spectrum RTKs and S/T kinase inhibitors as single agent or in combination with BRAF and/or MEK inhibitors. The rationale of these approaches is to prevent or overcome MAPK inhibitor dependent resistance mechanisms, although the appearance of systemic toxicities remains one of the main concerns when combining several small molecules (115-119). Other groups have attempted to tackle mechanisms of resistance related to the appearance of mutations in MEK1/2 and novel MEK inhibitors active in some of these mutants show interesting potential (120). An avenue of action expected to draw increasing attention is the use of ERK inhibitors (121-123). Whether in combination with BRAF or MEK inhibitors, small molecule inhibitors such as SCH772984 and GDC-0994 are being tested at present.
The central role of the PI3K signaling not only in melanoma development, but also in the innate and acquired resistance to MAPK inhibitors makes the PI3K/AKT/mTOR axis the second most attractive target in melanoma (2,44,45). While in vitro studies clearly show the potential of using AKT, pan-PI3K or dual PI3K/mTOR inhibitors in combination with BRAF/MEK inhibitor, the translation into the clinical setting is troublesome due to enhanced systemic toxicities (124-128). The PI3K family of proteins is relevant in many physiological processes and malignant conditions but nevertheless this interest on the pathway is producing encouraging data advancing in the understanding of melanoma specific PI3K-signaling (129) and lately PI3Kβ isoform specific inhibitors are being developed and trialed in the context of PTEN mutant melanoma patients (130-132). Interestingly targeting the PI3K has not only been proposed as a strategy to overcome resistance to BRAF/MEK inhibitors, but also with the therapeutic goal of preventing the onset of MEK1/2 inhibitor resistance in BRAF-mutated melanoma (133).
The potential of extending the efficacy of MAPK-inhibitor based therapies by targeting other melanoma relevant proteins is shown in a recent study (67), where in the above mentioned “drug-tolerance phase” when acquired resistance has not yet arisen, up-regulation of MITF contributes to increased survival signals. As previously mentioned in patients on BRAF/MEK inhibitor treatment MITF expression is upregulated, and this occurs through a transcriptional process in which PAX3 takes over the regulation of MITF transcription (66,67). A screen using FDA-approved drugs identified the HIV protease inhibitor Nelfinavir as inhibitor of MITF up-expression on treatment, and consequently Nelfinavir synergizes with BRAF and MEK inhibitors, suggesting that this combination could improve the efficacy and extend the clinical use of these drugs in BRAF mutant melanoma cells. Most importantly this study provides evidence suggesting the potential use of Nelfinavir mesylate in combination with MEK inhibitors in NRAS mutant cells and, importantly, in melanoma cells that progressed on BRAF/MEK inhibitors due to the appearance of activating mutations in NRAS (67). This study emphasizes that a greater understanding of the biology of melanoma cells during the early response to MAPK inhibitors should still provide us with clues for a better use of these drugs.
Conclusions
Despite its limitations, the application of targeted therapies to melanoma has been a historic milestone in cancer therapeutics. Indeed the unprecedented responses observed in BRAF mutant patients and the existence of a significant number of long-term responder highlights the success, but also reflects the paramount importance that the MAPK pathway plays in cancer in general and in melanoma in particular. Nevertheless the early appearance of resistance mechanisms once again demonstrates the relentless ability of cancer cells to adapt to cytotoxic challenges directly targeting driving signaling pathways. More recently the advent of immune-checkpoint therapies has opened an extremely promising therapeutic opportunity for melanoma patients, but this should not lead to a dismissal of targeted therapy approaches. Indeed, the recent “re-treatment” trial and continuous reports of pre-clinical studies describing novel combination approaches demonstrate that there is clearly room to improve the efficacy of the current BRAF/MEK targeting therapies.
Nevertheless, there is still need to explore alternative avenues of action to provide new treatments based on targeted therapies tackling mechanisms of resistance to BRAF/MEK inhibitors. Considering this, there are aspects of melanoma biology that require attention from the scientific community to better use MAPK targeted therapies, but also to gain insight into yet unknown therapeutic opportunities. For instance there is a very thought-provoking report suggesting patient’s age as a factor influencing both responsiveness to BRAF inhibitor and metastatic potential (134). In this context, the role of the tumor stroma of melanoma response to targeted therapies is still understudied as it is the effect of targeted therapies in the metastatic niches in which secondary tumor arise: why should a pulmonary melanoma metastasis respond equally as a liver or brain metastasis? A more defined study of visceral metastases and the role of specific anatomic location-dependent stromal characteristics might provide useful insight into innate and acquired mechanisms of resistance/sensibility to BRAF/MEK inhibitors. The interplay between cancer cell metabolism and response to targeted therapies too warrants further research. A growing number of reports are linking nutrient metabolism (glucose, glutamine) and melanoma cells responsiveness to BRAF inhibition while at the same time it is clear that the MAPK pathway regulates glycolysis and oxidative phosphorylation (16,135-141). All these gaps in our understanding of melanoma biology and the potential of managing MAPK inhibitor schedules with drug-holidays or combination with immunotherapies shows that there is room for improvement to better manage advanced melanoma.
Acknowledgements
Funding: C Wellbrock is supported by Cancer Research UK (C11591/A16416); I Arozarena is supported by a Miguel Servet fellowship (CP15/00176) from the Instituto de Salud Carlos III of the Spanish Ministry of Economy.
Footnote
Conflicts of Interest: The authors have no conflicts of interest to declare.
References
- Davies H, Bignell GR, Cox C, et al. Mutations of the BRAF gene in human cancer. Nature 2002;417:949-54. [Crossref] [PubMed]
- CancerGenomeAtlasNetwork. Genomic Classification of Cutaneous Melanoma. Cell 2015;161:1681-96. [Crossref] [PubMed]
- Wellbrock C, Karasarides M, Marais R. The RAF proteins take centre stage. Nat Rev Mol Cell Biol 2004;5:875-85. [Crossref] [PubMed]
- Wellbrock C, Ogilvie L, Hedley D, et al. V599EB-RAF is an oncogene in melanocytes. Cancer Res 2004;64:2338-42. [Crossref] [PubMed]
- Michaloglou C, Vredeveld LC, Soengas MS, et al. BRAFE600-associated senescence-like cell cycle arrest of human naevi. Nature 2005;436:720-4. [Crossref] [PubMed]
- Pollock PM, Harper UL, Hansen KS, et al. High frequency of BRAF mutations in nevi. Nat Genet 2003;33:19-20. [Crossref] [PubMed]
- Dankort D, Curley DP, Cartlidge RA, et al. Braf(V600E) cooperates with Pten loss to induce metastatic melanoma. Nat Genet 2009;41:544-52. [Crossref] [PubMed]
- Dhomen N, Reis-Filho JS, da Rocha Dias S, et al. Oncogenic Braf induces melanocyte senescence and melanoma in mice. Cancer Cell 2009;15:294-303. [Crossref] [PubMed]
- Viros A, Sanchez-Laorden B, Pedersen M, et al. Ultraviolet radiation accelerates BRAF-driven melanomagenesis by targeting TP53. Nature 2014;511:478-82. [Crossref] [PubMed]
- Wellbrock C, Gomez A, Schartl M. Melanoma development and pigment cell transformation in xiphophorus. Microsc Res Tech 2002;58:456-63. [Crossref] [PubMed]
- Wellbrock C, Schartl M. Multiple binding sites in the growth factor receptor Xmrk mediate binding to p59fyn, GRB2 and Shc. Eur J Biochem 1999;260:275-83. [Crossref] [PubMed]
- Kortylewski M, Heinrich PC, Kauffmann ME, et al. Mitogen-activated protein kinases control p27/Kip1 expression and growth of human melanoma cells. Biochem J 2001;357:297-303. [Crossref] [PubMed]
- Li G, Schaider H, Satyamoorthy K, et al. Downregulation of E-cadherin and Desmoglein 1 by autocrine hepatocyte growth factor during melanoma development. Oncogene 2001;20:8125-35. [Crossref] [PubMed]
- Cohen C, Zavala-Pompa A, Sequeira JH, et al. Mitogen-actived protein kinase activation is an early event in melanoma progression. Clin Cancer Res 2002;8:3728-33. [PubMed]
- Gaggioli C, Robert G, Bertolotto C, et al. Tumor-derived fibronectin is involved in melanoma cell invasion and regulated by V600E B-Raf signaling pathway. J Invest Dermatol 2007;127:400-10. [Crossref] [PubMed]
- Haq R, Fisher DE, Widlund HR. Molecular pathways: BRAF induces bioenergetic adaptation by attenuating oxidative phosphorylation. Clin Cancer Res 2014;20:2257-63. [Crossref] [PubMed]
- Haq R, Yokoyama S, Hawryluk EB, et al. BCL2A1 is a lineage-specific antiapoptotic melanoma oncogene that confers resistance to BRAF inhibition. Proc Natl Acad Sci U S A 2013;110:4321-6. [Crossref] [PubMed]
- Huntington JT, Shields JM, Der CJ, et al. Overexpression of collagenase 1 (MMP-1) is mediated by the ERK pathway in invasive melanoma cells: role of BRAF mutation and fibroblast growth factor signaling. J Biol Chem 2004;279:33168-76. [Crossref] [PubMed]
- Johansson CC, Egyhazi S, Masucci G, et al. Prognostic significance of tumor iNOS and COX-2 in stage III malignant cutaneous melanoma. Cancer Immunol Immunother 2009;58:1085-94. [Crossref] [PubMed]
- Karasarides M, Chiloeches A, Hayward R, et al. B-RAF is a therapeutic target in melanoma. Oncogene 2004;23:6292-8. [Crossref] [PubMed]
- Klein RM, Spofford LS, Abel EV, et al. B-RAF regulation of Rnd3 participates in actin cytoskeletal and focal adhesion organization. Mol Biol Cell 2008;19:498-508. [Crossref] [PubMed]
- Kumar SM, Yu H, Edwards R, et al. Mutant V600E BRAF increases hypoxia inducible factor-1alpha expression in melanoma. Cancer Res 2007;67:3177-84. [Crossref] [PubMed]
- Lyons JF, Wilhelm S, Hibner B, et al. Discovery of a novel Raf kinase inhibitor. Endocr Relat Cancer 2001;8:219-25. [Crossref] [PubMed]
- Eisen T, Ahmad T, Flaherty KT, et al. Sorafenib in advanced melanoma: a Phase II randomised discontinuation trial analysis. Br J Cancer 2006;95:581-6. [Crossref] [PubMed]
- Larkin JM, Eisen T. Renal cell carcinoma and the use of sorafenib. Ther Clin Risk Manag 2006;2:87-98. [PubMed]
- Mangana J, Levesque MP, Karpova MB, et al. Sorafenib in melanoma. Expert Opin Investig Drugs 2012;21:557-68. [Crossref] [PubMed]
- Bollag G, Hirth P, Tsai J, et al. Clinical efficacy of a RAF inhibitor needs broad target blockade in BRAF-mutant melanoma. Nature 2010;467:596-9. [Crossref] [PubMed]
- Waizenegger IC, Baum A, Steurer S, et al. A Novel RAF Kinase Inhibitor with DFG-Out-Binding Mode: High Efficacy in BRAF-Mutant Tumor Xenograft Models in the Absence of Normal Tissue Hyperproliferation. Mol Cancer Ther 2016;15:354-65. [Crossref] [PubMed]
- Flaherty KT, Puzanov I, Kim KB, et al. Inhibition of mutated, activated BRAF in metastatic melanoma. N Engl J Med 2010;363:809-19. [Crossref] [PubMed]
- Sosman JA, Kim KB, Schuchter L, et al. Survival in BRAF V600-mutant advanced melanoma treated with vemurafenib. N Engl J Med 2012;366:707-14. [Crossref] [PubMed]
- Hauschild A, Grob JJ, Demidov LV, et al. Dabrafenib in BRAF-mutated metastatic melanoma: a multicentre, open-label, phase 3 randomised controlled trial. Lancet 2012;380:358-65. [Crossref] [PubMed]
- Banzi M, De Blasio S, Lallas A, et al. Dabrafenib: a new opportunity for the treatment of BRAF V600-positive melanoma. Onco Targets Ther 2016;9:2725-33. [PubMed]
- Ascierto PA, Minor D, Ribas A, et al. Phase II trial (BREAK-2) of the BRAF inhibitor dabrafenib (GSK2118436) in patients with metastatic melanoma. J Clin Oncol 2013;31:3205-11. [Crossref] [PubMed]
- Chapman PB, Hauschild A, Robert C, et al. Improved survival with vemurafenib in melanoma with BRAF V600E mutation. N Engl J Med 2011;364:2507-16. [Crossref] [PubMed]
- McArthur GA, Chapman PB, Robert C, et al. Safety and efficacy of vemurafenib in BRAF(V600E) and BRAF(V600K) mutation-positive melanoma (BRIM-3): extended follow-up of a phase 3, randomised, open-label study. Lancet Oncol 2014;15:323-32. [Crossref] [PubMed]
- Heidorn SJ, Milagre C, Whittaker S, et al. Kinase-dead BRAF and oncogenic RAS cooperate to drive tumor progression through CRAF. Cell 2010;140:209-21. [Crossref] [PubMed]
- Su F, Viros A, Milagre C, et al. RAS mutations in cutaneous squamous-cell carcinomas in patients treated with BRAF inhibitors. N Engl J Med 2012;366:207-15. [Crossref] [PubMed]
- Andrews MC, Behren A, Chionh F, et al. BRAF inhibitor-driven tumor proliferation in a KRAS-mutated colon carcinoma is not overcome by MEK1/2 inhibition. J Clin Oncol 2013;31:e448-51. [Crossref] [PubMed]
- Boussemart L, Girault I, Malka-Mahieu H, et al. Secondary Tumors Arising in Patients Undergoing BRAF Inhibitor Therapy Exhibit Increased BRAF-CRAF Heterodimerization. Cancer Res 2016;76:1476-84. [Crossref] [PubMed]
- Callahan MK, Rampal R, Harding JJ, et al. Progression of RAS-mutant leukemia during RAF inhibitor treatment. N Engl J Med 2012;367:2316-21. [Crossref] [PubMed]
- Grey A, Cooper A, McNeil C, et al. Progression of KRAS mutant pancreatic adenocarcinoma during vemurafenib treatment in a patient with metastatic melanoma. Intern Med J 2014;44:597-600. [Crossref] [PubMed]
- Long GV, Weber JS, Infante JR, et al. Overall Survival and Durable Responses in Patients With BRAF V600-Mutant Metastatic Melanoma Receiving Dabrafenib Combined With Trametinib. J Clin Oncol 2016;34:871-8. [Crossref] [PubMed]
- Turajlic S, Furney SJ, Stamp G, et al. Whole-genome sequencing reveals complex mechanisms of intrinsic resistance to BRAF inhibition. Ann Oncol 2014;25:959-67. [Crossref] [PubMed]
- Shi H, Hugo W, Kong X, et al. Acquired resistance and clonal evolution in melanoma during BRAF inhibitor therapy. Cancer Discov 2014;4:80-93. [Crossref] [PubMed]
- Van Allen EM, Wagle N, Sucker A, et al. The genetic landscape of clinical resistance to RAF inhibition in metastatic melanoma. Cancer Discov 2014;4:94-109. [Crossref] [PubMed]
- Nazarian R, Shi H, Wang Q, et al. Melanomas acquire resistance to B-RAF(V600E) inhibition by RTK or N-RAS upregulation. Nature 2010;468:973-7. [Crossref] [PubMed]
- Whittaker SR, Theurillat JP, Van Allen E, et al. A genome-scale RNA interference screen implicates NF1 loss in resistance to RAF inhibition. Cancer Discov 2013;3:350-62. [Crossref] [PubMed]
- Girotti MR, Pedersen M, Sanchez-Laorden B, et al. Inhibiting EGF receptor or SRC family kinase signaling overcomes BRAF inhibitor resistance in melanoma. Cancer Discov 2013;3:158-67. [Crossref] [PubMed]
- Villanueva J, Vultur A, Lee JT, et al. Acquired resistance to BRAF inhibitors mediated by a RAF kinase switch in melanoma can be overcome by cotargeting MEK and IGF-1R/PI3K. Cancer Cell 2010;18:683-95. [Crossref] [PubMed]
- Poulikakos PI, Persaud Y, Janakiraman M, et al. RAF inhibitor resistance is mediated by dimerization of aberrantly spliced BRAF(V600E). Nature 2011;480:387-90. [Crossref] [PubMed]
- Shi H, Moriceau G, Kong X, et al. Melanoma whole-exome sequencing identifies (V600E)B-RAF amplification-mediated acquired B-RAF inhibitor resistance. Nat Commun 2012;3:724. [Crossref] [PubMed]
- Emery CM, Vijayendran KG, Zipser MC, et al. MEK1 mutations confer resistance to MEK and B-RAF inhibition. Proc Natl Acad Sci U S A 2009;106:20411-6. [Crossref] [PubMed]
- Johannessen CM, Boehm JS, Kim SY, et al. COT drives resistance to RAF inhibition through MAP kinase pathway reactivation. Nature 2010;468:968-72. [Crossref] [PubMed]
- Long GV, Fung C, Menzies AM, et al. Increased MAPK reactivation in early resistance to dabrafenib/trametinib combination therapy of BRAF-mutant metastatic melanoma. Nat Commun 2014;5:5694. [Crossref] [PubMed]
- Marusiak AA, Edwards ZC, Hugo W, et al. Mixed lineage kinases activate MEK independently of RAF to mediate resistance to RAF inhibitors. Nat Commun 2014;5:3901. [Crossref] [PubMed]
- Montagut C, Sharma SV, Shioda T, et al. Elevated CRAF as a potential mechanism of acquired resistance to BRAF inhibition in melanoma. Cancer Res 2008;68:4853-61. [Crossref] [PubMed]
- Wagle N, Emery C, Berger MF, et al. Dissecting therapeutic resistance to RAF inhibition in melanoma by tumor genomic profiling. J Clin Oncol 2011;29:3085-96. [Crossref] [PubMed]
- Straussman R, Morikawa T, Shee K, et al. Tumour micro-environment elicits innate resistance to RAF inhibitors through HGF secretion. Nature 2012;487:500-4. [Crossref] [PubMed]
- Shi H, Hong A, Kong X, et al. A novel AKT1 mutant amplifies an adaptive melanoma response to BRAF inhibition. Cancer Discov 2014;4:69-79. [Crossref] [PubMed]
- Abel EV, Basile KJ, Kugel CH 3rd, et al. Melanoma adapts to RAF/MEK inhibitors through FOXD3-mediated upregulation of ERBB3. J Clin Invest 2013;123:2155-68. [Crossref] [PubMed]
- Fattore L, Marra E, Pisanu ME, et al. Activation of an early feedback survival loop involving phospho-ErbB3 is a general response of melanoma cells to RAF/MEK inhibition and is abrogated by anti-ErbB3 antibodies. J Transl Med 2013;11:180. [Crossref] [PubMed]
- Paraiso KH, Xiang Y, Rebecca VW, et al. PTEN loss confers BRAF inhibitor resistance to melanoma cells through the suppression of BIM expression. Cancer Res 2011;71:2750-60. [Crossref] [PubMed]
- Wilson TR, Fridlyand J, Yan Y, et al. Widespread potential for growth-factor-driven resistance to anticancer kinase inhibitors. Nature 2012;487:505-9. [Crossref] [PubMed]
- Wellbrock C, Arozarena I. Microphthalmia-associated transcription factor in melanoma development and MAP-kinase pathway targeted therapy. Pigment Cell Melanoma Res 2015;28:390-406. [Crossref] [PubMed]
- Müller J, Krijgsman O, Tsoi J, et al. Low MITF/AXL ratio predicts early resistance to multiple targeted drugs in melanoma. Nat Commun 2014;5:5712. [Crossref] [PubMed]
- Smith MP, Ferguson J, Arozarena I, et al. Effect of SMURF2 targeting on susceptibility to MEK inhibitors in melanoma. J Natl Cancer Inst 2013;105:33-46. [Crossref] [PubMed]
- Smith MP, Brunton H, Rowling EJ, et al. Inhibiting Drivers of Non-mutational Drug Tolerance Is a Salvage Strategy for Targeted Melanoma Therapy. Cancer Cell 2016;29:270-84. [Crossref] [PubMed]
- Konieczkowski DJ, Johannessen CM, Abudayyeh O, et al. A melanoma cell state distinction influences sensitivity to MAPK pathway inhibitors. Cancer Discov 2014;4:816-27. [Crossref] [PubMed]
- Tirosh I, Izar B, Prakadan SM, et al. Dissecting the multicellular ecosystem of metastatic melanoma by single-cell RNA-seq. Science 2016;352:189-96. [Crossref] [PubMed]
- Hirata E, Girotti MR, Viros A, et al. Intravital imaging reveals how BRAF inhibition generates drug-tolerant microenvironments with high integrin beta1/FAK signaling. Cancer Cell 2015;27:574-88. [Crossref] [PubMed]
- Smith MP, Sanchez-Laorden B, O'Brien K, et al. The immune microenvironment confers resistance to MAPK pathway inhibitors through macrophage-derived TNFalpha. Cancer Discov 2014;4:1214-29. [Crossref] [PubMed]
- Wang T, Xiao M, Ge Y, et al. BRAF Inhibition Stimulates Melanoma-Associated Macrophages to Drive Tumor Growth. Clin Cancer Res 2015;21:1652-64. [Crossref] [PubMed]
- Young HL, Rowling EJ, Bugatti M, et al. An adaptive signaling network in melanoma inflammatory niches confers tolerance to MAPK signaling inhibition. J Exp Med 2017;214:1691-710. [Crossref] [PubMed]
- Berghoff AS, Preusser M. Targeted Therapies for Melanoma Brain Metastases. Curr Treat Options Neurol 2017;19:13. [Crossref] [PubMed]
- Seifert H, Hirata E, Gore M, et al. Extrinsic factors can mediate resistance to BRAF inhibition in central nervous system melanoma metastases. Pigment Cell Melanoma Res 2016;29:92-100. [Crossref] [PubMed]
- Obenauf AC, Zou Y, Ji AL, et al. Therapy-induced tumour secretomes promote resistance and tumour progression. Nature 2015;520:368-72. [Crossref] [PubMed]
- Smith MP, Rowling EJ, Miskolczi Z, et al. Targeting endothelin receptor signalling overcomes heterogeneity driven therapy failure. EMBO Mol Med 2017;9:1011-29. [Crossref] [PubMed]
- Haass NK, Sproesser K, Nguyen TK, et al. The mitogen-activated protein/extracellular signal-regulated kinase kinase inhibitor AZD6244 (ARRY-142886) induces growth arrest in melanoma cells and tumor regression when combined with docetaxel. Clin Cancer Res 2008;14:230-9. [Crossref] [PubMed]
- Solit DB, Garraway LA, Pratilas CA, et al. BRAF mutation predicts sensitivity to MEK inhibition. Nature 2006;439:358-62. [Crossref] [PubMed]
- Ascierto PA, Schadendorf D, Berking C, et al. MEK162 for patients with advanced melanoma harbouring NRAS or Val600 BRAF mutations: a non-randomised, open-label phase 2 study. Lancet Oncol 2013;14:249-56. [Crossref] [PubMed]
- Catalanotti F, Solit DB, Pulitzer MP, et al. Phase II trial of MEK inhibitor selumetinib (AZD6244, ARRY-142886) in patients with BRAFV600E/K-mutated melanoma. Clin Cancer Res 2013;19:2257-64. [Crossref] [PubMed]
- Flaherty KT, Robert C, Hersey P, et al. Improved survival with MEK inhibition in BRAF-mutated melanoma. N Engl J Med 2012;367:107-14. [Crossref] [PubMed]
- Kirkwood JM, Bastholt L, Robert C, et al. Phase II, open-label, randomized trial of the MEK1/2 inhibitor selumetinib as monotherapy versus temozolomide in patients with advanced melanoma. Clin Cancer Res 2012;18:555-67. [Crossref] [PubMed]
- Patel SP, Lazar AJ, Papadopoulos NE, et al. Clinical responses to selumetinib (AZD6244; ARRY-142886)-based combination therapy stratified by gene mutations in patients with metastatic melanoma. Cancer 2013;119:799-805. [Crossref] [PubMed]
- Robert C, Dummer R, Gutzmer R, et al. Selumetinib plus dacarbazine versus placebo plus dacarbazine as first-line treatment for BRAF-mutant metastatic melanoma: a phase 2 double-blind randomised study. Lancet Oncol 2013;14:733-40. [Crossref] [PubMed]
- Johnson DB, Flaherty KT, Weber JS, et al. Combined BRAF (Dabrafenib) and MEK inhibition (Trametinib) in patients with BRAFV600-mutant melanoma experiencing progression with single-agent BRAF inhibitor. J Clin Oncol 2014;32:3697-704. [Crossref] [PubMed]
- Ascierto PA, McArthur GA, Dreno B, et al. Cobimetinib combined with vemurafenib in advanced BRAF(V600)-mutant melanoma (coBRIM): updated efficacy results from a randomised, double-blind, phase 3 trial. Lancet Oncol 2016;17:1248-60. [Crossref] [PubMed]
- Larkin J, Ascierto PA, Dreno B, et al. Combined vemurafenib and cobimetinib in BRAF-mutated melanoma. N Engl J Med 2014;371:1867-76. [Crossref] [PubMed]
- Ribas A, Gonzalez R, Pavlick A, et al. Combination of vemurafenib and cobimetinib in patients with advanced BRAF(V600)-mutated melanoma: a phase 1b study. Lancet Oncol 2014;15:954-65. [Crossref] [PubMed]
- Long GV, Stroyakovskiy D, Gogas H, et al. Dabrafenib and trametinib versus dabrafenib and placebo for Val600 BRAF-mutant melanoma: a multicentre, double-blind, phase 3 randomised controlled trial. Lancet 2015;386:444-51. [Crossref] [PubMed]
- Robert C, Karaszewska B, Schachter J, et al. Improved overall survival in melanoma with combined dabrafenib and trametinib. N Engl J Med 2015;372:30-9. [Crossref] [PubMed]
- Daud A, Gill J, Kamra S, et al. Indirect treatment comparison of dabrafenib plus trametinib versus vemurafenib plus cobimetinib in previously untreated metastatic melanoma patients. J Hematol Oncol 2017;10:3. [Crossref] [PubMed]
- Galván-Banqueri M, Ubago-Perez R, Molina-Lopez T. The relative clinical efficacy of trametinib-dabrafenib and cobimetinib-vemurafenib in advanced melanoma: an indirect comparison. J Clin Pharm Ther 2016;41:285-9. [Crossref] [PubMed]
- Sanlorenzo M, Choudhry A, Vujic I, et al. Comparative profile of cutaneous adverse events: BRAF/MEK inhibitor combination therapy versus BRAF monotherapy in melanoma. J Am Acad Dermatol 2014;71:1102-9. e1.
- Grob JJ, Amonkar MM, Karaszewska B, et al. Comparison of dabrafenib and trametinib combination therapy with vemurafenib monotherapy on health-related quality of life in patients with unresectable or metastatic cutaneous BRAF Val600-mutation-positive melanoma (COMBI-v): results of a phase 3, open-label, randomised trial. Lancet Oncol 2015;16:1389-98. [Crossref] [PubMed]
- Schadendorf D, Amonkar MM, Stroyakovskiy D, et al. Health-related quality of life impact in a randomised phase III study of the combination of dabrafenib and trametinib versus dabrafenib monotherapy in patients with BRAF V600 metastatic melanoma. Eur J Cancer 2015;51:833-40. [Crossref] [PubMed]
- Puzanov I, Amaravadi RK, McArthur GA, et al. Long-term outcome in BRAF(V600E) melanoma patients treated with vemurafenib: Patterns of disease progression and clinical management of limited progression. Eur J Cancer 2015;51:1435-43. [Crossref] [PubMed]
- Villanueva J, Infante JR, Krepler C, et al. Concurrent MEK2 mutation and BRAF amplification confer resistance to BRAF and MEK inhibitors in melanoma. Cell Rep 2013;4:1090-9. [Crossref] [PubMed]
- Wagle N, Van Allen EM, Treacy DJ, et al. MAP kinase pathway alterations in BRAF-mutant melanoma patients with acquired resistance to combined RAF/MEK inhibition. Cancer Discov 2014;4:61-8. [Crossref] [PubMed]
- Lito P, Pratilas CA, Joseph EW, et al. Relief of profound feedback inhibition of mitogenic signaling by RAF inhibitors attenuates their activity in BRAFV600E melanomas. Cancer Cell 2012;22:668-82. [Crossref] [PubMed]
- Smith MP, Wellbrock C. Molecular Pathways: Maintaining MAPK Inhibitor Sensitivity by Targeting Nonmutational Tolerance. Clin Cancer Res 2016;22:5966-70. [Crossref] [PubMed]
- Abdel-Wahab O, Klimek VM, Gaskell AA, et al. Efficacy of intermittent combined RAF and MEK inhibition in a patient with concurrent BRAF- and NRAS-mutant malignancies. Cancer Discov 2014;4:538-45. [Crossref] [PubMed]
- Das Thakur M, Salangsang F, Landman AS, et al. Modelling vemurafenib resistance in melanoma reveals a strategy to forestall drug resistance. Nature 2013;494:251-5. [Crossref] [PubMed]
- Schreuer M, Jansen Y, Planken S, et al. Combination of dabrafenib plus trametinib for BRAF and MEK inhibitor pretreated patients with advanced BRAFV600-mutant melanoma: an open-label, single arm, dual-centre, phase 2 clinical trial. Lancet Oncol 2017;18:464-72. [Crossref] [PubMed]
- Atefi M, Titz B, Avramis E, et al. Combination of pan-RAF and MEK inhibitors in NRAS mutant melanoma. Mol Cancer 2015;14:27. [Crossref] [PubMed]
- Girotti MR, Lopes F, Preece N, et al. Paradox-breaking RAF inhibitors that also target SRC are effective in drug-resistant BRAF mutant melanoma. Cancer Cell 2015;27:85-96. [Crossref] [PubMed]
- Nakamura A, Arita T, Tsuchiya S, et al. Antitumor activity of the selective pan-RAF inhibitor TAK-632 in BRAF inhibitor-resistant melanoma. Cancer Res 2013;73:7043-55. [Crossref] [PubMed]
- Peng SB, Henry JR, Kaufman MD, et al. Inhibition of RAF Isoforms and Active Dimers by LY3009120 Leads to Anti-tumor Activities in RAS or BRAF Mutant Cancers. Cancer Cell 2015;28:384-98. [Crossref] [PubMed]
- Yao Z, Torres NM, Tao A, et al. BRAF Mutants Evade ERK-Dependent Feedback by Different Mechanisms that Determine Their Sensitivity to Pharmacologic Inhibition. Cancer Cell 2015;28:370-83. [Crossref] [PubMed]
- Zhang C, Spevak W, Zhang Y, et al. RAF inhibitors that evade paradoxical MAPK pathway activation. Nature 2015;526:583-6. [Crossref] [PubMed]
- da Rocha Dias S, Friedlos F, Light Y, et al. Activated B-RAF is an Hsp90 client protein that is targeted by the anticancer drug 17-allylamino-17-demethoxygeldanamycin. Cancer Res 2005;65:10686-91. [Crossref] [PubMed]
- Grbovic OM, Basso AD, Sawai A, et al. V600E B-Raf requires the Hsp90 chaperone for stability and is degraded in response to Hsp90 inhibitors. Proc Natl Acad Sci U S A 2006;103:57-62. [Crossref] [PubMed]
- Acquaviva J, Smith DL, Jimenez JP, et al. Overcoming acquired BRAF inhibitor resistance in melanoma via targeted inhibition of Hsp90 with ganetespib. Mol Cancer Ther 2014;13:353-63. [Crossref] [PubMed]
- Paraiso KH, Haarberg HE, Wood E, et al. The HSP90 inhibitor XL888 overcomes BRAF inhibitor resistance mediated through diverse mechanisms. Clin Cancer Res 2012;18:2502-14. [Crossref] [PubMed]
- Cheng H, Chang Y, Zhang L, et al. Identification and optimization of new dual inhibitors of B-Raf and epidermal growth factor receptor kinases for overcoming resistance against vemurafenib. J Med Chem 2014;57:2692-703. [Crossref] [PubMed]
- Langdon CG, Held MA, Platt JT, et al. The broad-spectrum receptor tyrosine kinase inhibitor dovitinib suppresses growth of BRAF-mutant melanoma cells in combination with other signaling pathway inhibitors. Pigment Cell Melanoma Res 2015;28:417-30. [Crossref] [PubMed]
- Phadke MS, Sini P, Smalley KS. The Novel ATP-Competitive MEK/Aurora Kinase Inhibitor BI-847325 Overcomes Acquired BRAF Inhibitor Resistance through Suppression of Mcl-1 and MEK Expression. Mol Cancer Ther 2015;14:1354-64. [Crossref] [PubMed]
- Shi H, Kong X, Ribas A, et al. Combinatorial treatments that overcome PDGFRbeta-driven resistance of melanoma cells to V600EB-RAF inhibition. Cancer Res 2011;71:5067-74. [Crossref] [PubMed]
- Turner MC, Rossfeld K, Salama AK, et al. Can binimetinib, encorafenib and masitinib be more efficacious than currently available mutation-based targeted therapies for melanoma treatment? Expert Opin Pharmacother 2017;18:487-95. [Crossref] [PubMed]
- Narita Y, Okamoto K, Kawada MI, et al. Novel ATP-competitive MEK inhibitor E6201 is effective against vemurafenib-resistant melanoma harboring the MEK1-C121S mutation in a preclinical model. Mol Cancer Ther 2014;13:823-32. [Crossref] [PubMed]
- Hatzivassiliou G, Liu B, O'Brien C, et al. ERK inhibition overcomes acquired resistance to MEK inhibitors. Mol Cancer Ther 2012;11:1143-54. [Crossref] [PubMed]
- Herrero A, Pinto A, Colon-Bolea P, et al. Small Molecule Inhibition of ERK Dimerization Prevents Tumorigenesis by RAS-ERK Pathway Oncogenes. Cancer Cell 2015;28:170-82. [Crossref] [PubMed]
- Morris EJ, Jha S, Restaino CR, et al. Discovery of a novel ERK inhibitor with activity in models of acquired resistance to BRAF and MEK inhibitors. Cancer Discov 2013;3:742-50. [Crossref] [PubMed]
- Atefi M, von Euw E, Attar N, et al. Reversing melanoma cross-resistance to BRAF and MEK inhibitors by co-targeting the AKT/mTOR pathway. PLoS One 2011;6:e28973. [Crossref] [PubMed]
- Bedard PL, Tabernero J, Janku F, et al. A phase Ib dose-escalation study of the oral pan-PI3K inhibitor buparlisib (BKM120) in combination with the oral MEK1/2 inhibitor trametinib (GSK1120212) in patients with selected advanced solid tumors. Clin Cancer Res 2015;21:730-8. [Crossref] [PubMed]
- Greger JG, Eastman SD, Zhang V, et al. Combinations of BRAF, MEK, and PI3K/mTOR inhibitors overcome acquired resistance to the BRAF inhibitor GSK2118436 dabrafenib, mediated by NRAS or MEK mutations. Mol Cancer Ther 2012;11:909-20. [Crossref] [PubMed]
- Sweetlove M, Wrightson E, Kolekar S, et al. Inhibitors of pan-PI3K Signaling Synergize with BRAF or MEK Inhibitors to Prevent BRAF-Mutant Melanoma Cell Growth. Front Oncol 2015;5:135. [Crossref] [PubMed]
- Tolcher AW, Patnaik A, Papadopoulos KP, et al. Phase I study of the MEK inhibitor trametinib in combination with the AKT inhibitor afuresertib in patients with solid tumors and multiple myeloma. Cancer Chemother Pharmacol 2015;75:183-9. [Crossref] [PubMed]
- Kwong LN, Davies MA. Navigating the therapeutic complexity of PI3K pathway inhibition in melanoma. Clin Cancer Res 2013;19:5310-9. [Crossref] [PubMed]
- Bonnevaux H, Lemaitre O, Vincent L, et al. Concomitant Inhibition of PI3Kbeta and BRAF or MEK in PTEN-Deficient/BRAF-Mutant Melanoma Treatment: Preclinical Assessment of SAR260301 Oral PI3Kbeta-Selective Inhibitor. Mol Cancer Ther 2016;15:1460-71. [Crossref] [PubMed]
- Certal V, Carry JC, Halley F, et al. Discovery and optimization of pyrimidone indoline amide PI3Kbeta inhibitors for the treatment of phosphatase and tensin homologue (PTEN)-deficient cancers. J Med Chem 2014;57:903-20. [Crossref] [PubMed]
- Herkert B, Kauffmann A, Molle S, et al. Maximizing the Efficacy of MAPK-Targeted Treatment in PTENLOF/BRAFMUT Melanoma through PI3K and IGF1R Inhibition. Cancer Res 2016;76:390-402. [Crossref] [PubMed]
- Deuker MM, Marsh Durban V, Phillips WA, et al. PI3'-kinase inhibition forestalls the onset of MEK1/2 inhibitor resistance in BRAF-mutated melanoma. Cancer Discov 2015;5:143-53. [Crossref] [PubMed]
- Kaur A, Webster MR, Marchbank K, et al. sFRP2 in the aged microenvironment drives melanoma metastasis and therapy resistance. Nature 2016;532:250-4. [Crossref] [PubMed]
- Baenke F, Chaneton B, Smith M, et al. Resistance to BRAF inhibitors induces glutamine dependency in melanoma cells. Mol Oncol 2016;10:73-84. [Crossref] [PubMed]
- Delgado-Goni T, Miniotis MF, Wantuch S, et al. The BRAF Inhibitor Vemurafenib Activates Mitochondrial Metabolism and Inhibits Hyperpolarized Pyruvate-Lactate Exchange in BRAF-Mutant Human Melanoma Cells. Mol Cancer Ther 2016;15:2987-99. [Crossref] [PubMed]
- Falletta P, Sanchez-Del-Campo L, Chauhan J, et al. Translation reprogramming is an evolutionarily conserved driver of phenotypic plasticity and therapeutic resistance in melanoma. Genes Dev 2017;31:18-33. [Crossref] [PubMed]
- Ferguson J, Smith M, Zudaire I, et al. Glucose availability controls ATF4-mediated MITF suppression to drive melanoma cell growth. Oncotarget 2017. [Crossref] [PubMed]
- Hall A, Meyle KD, Lange MK, et al. Dysfunctional oxidative phosphorylation makes malignant melanoma cells addicted to glycolysis driven by the (V600E)BRAF oncogene. Oncotarget 2013;4:584-99. [Crossref] [PubMed]
- Hardeman KN, Peng C, Paudel BB, et al. Dependence On Glycolysis Sensitizes BRAF-mutated Melanomas For Increased Response To Targeted BRAF Inhibition. Sci Rep 2017;7:42604. [Crossref] [PubMed]
- Parmenter TJ, Kleinschmidt M, Kinross KM, et al. Response of BRAF-mutant melanoma to BRAF inhibition is mediated by a network of transcriptional regulators of glycolysis. Cancer Discov 2014;4:423-33. [Crossref] [PubMed]