How to be young at heart? miR-22 as a potential therapeutic target to boost autophagy and protect the old myocardium
Cardiovascular diseases remain the most common cause of death in Western countries and their prevalence in the general population increases progressively with age, reaching more than 60% in subjects older than 60 years (1). This is at least in part due to a progressive impairment of the cellular processes regulating cardiac and vascular homeostasis, finally leading to the development of cardiovascular abnormalities (2). In addition, the molecular mechanisms that protect the heart against stress are downregulated by aging, making the myocardium more susceptible to injury (2). It is therefore important to find new therapeutic targets to reduce cardiovascular aging and protect the aged myocardium from stress.
Autophagy is a mechanism of intracellular degradation through lysosomes characterized by the presence of double membrane vesicles called autophagosomes (2,3). Increasing lines of evidence suggest that autophagy plays an important role in maintaining cardiac homeostasis. Autophagy is downregulated in the heart during aging, and downregulation of autophagy plays a causative role in the progression of aging in the heart (2,4). Furthermore, we and others have shown that downregulation of autophagy during pressure overload (5,6), post-myocardial infarction cardiac remodeling (7) and metabolic syndrome (8,9) contributes to the progression of cardiac dysfunction and ischemic injury. On the other hand, excessive activation of autophagy during the acute phase of myocardial infarction or ischemia/reperfusion may be detrimental (10). Thus, identifying molecular interventions to control the activity of autophagy is important and may lead to the development of a novel treatment for heart disease, especially in elderly patients.
Using high-throughput screening based on the fluorescence-activated cell sorting-based detection of GFP-LC3 protein and library screening, Gupta et al. identified miR-22 as a strong inhibitor of autophagy in cardiomyocytes (11). The authors showed that miR-22 inhibits the reduction in GFP-LC3 signal in response to starvation, an established stimulus for autophagy. This screening method is based on the fact that the GFP-LC3 signal is attenuated when GFP-LC3 autophagosomes move from the cytosol to lysosomes (12). Thus, it is a convenient and reliable method to evaluate the level of autophagic flux from autophagosome formation to autolysosome formation. A cautionary note, however, is that the results of this method are easily affected by conditions that alter the intensity of the GFP signal through autophagy-independent mechanisms. Thus, the authors could have conducted more extensive validation regarding how miR-22 affects autophagic flux in cardiomyocytes throughout the study. Nevertheless, this is a fascinating way to identify effective modifiers of autophagy in cardiomyocytes and, by extending this approach to small molecules, it may be possible to identify novel interventions for heart failure patients.
Gupta et al. demonstrated that miR-22 expression progressively increases in the mouse heart during aging (11). miR-22 inhibition by locked nucleic acid-based anti-miR-22 (LNA-anti-miR-22) significantly attenuated cardiac remodeling in old mice subjected to permanent coronary artery ligation, reducing left ventricular dilation and improving systolic function. In contrast, the beneficial effects of miR-22 inhibition were not obvious in young mice with chronic myocardial infarction. Thus, miR-22 upregulation in the aged heart appears to impair adaptation to chronic myocardial infarction. miR-22 inhibition limits senescence-induced cardiomyocyte hypertrophy in vitro. Therefore, the cardioprotective effects of pharmacological miR-22 inhibition in the mouse heart may be dependent on its protective actions in cardiomyocytes. However, since miR-22 also induces senescence in cardiac fibroblasts, increasing their migratory capacity (13), it is possible that miR-22 inhibition may also elicit beneficial functions through the other cell types in the heart.
miR-22 appeared to be responsible for the reduction of autophagy in the old myocardium. The increase in cardiac miR-22 levels during aging was paralleled by a progressive decline in myocardial autophagy. Importantly, miR-22 inhibition appears to rescue autophagy in aging hearts (11). miR-22 may have autophagy-independent actions, and thus, the causative involvement of autophagy in mediating the salutary actions of miR-22 inhibition remains to be demonstrated. Nevertheless, the fact that miR-22 inhibition can stimulate autophagy in the aging heart is attractive. Restoring the level of autophagy has been shown to be salutary in some cardiovascular conditions. For example, genetic inhibition of the serine-threonine kinase MST1, a stress activated kinase known to inhibit autophagy, reduces remodeling caused by chronic ischemia through the activation of autophagy (7). In addition, administration of spermidine, a natural polyamine compound, extended life span in mice and reduced cardiac hypertrophy and diastolic dysfunction induced by aging. These effects were found to be dependent on the capacity of spermidine to activate autophagy through the inhibition of the histone acetyltransferase p300. In fact, the cardioprotective effects of spermidine were lost in mice with genetic disruption of autophagy (4).
Other miRs also regulate autophagy in the heart. Thum’s group elegantly showed that miRNA-212/132 inhibits autophagy in cardiomyocytes by targeting FoxO3a. Pharmacological inhibition of miR-132 reduced cardiac hypertrophy and heart failure induced by pressure overload (14). In addition, exosome-mediated miR-145 administration was found to reduce ischemic injury by activating autophagy (15). Thus, targeting these miRNAs could represent a new avenue of exploration in the development of novel interventions to treat heart failure in elderly patients.
miR-22a plays a dual role in the heart, performing both physiological and maladaptive functions. Mice with either systemic or cardiac-specific miR-22 gene deletion do not develop compensatory hypertrophy in response to isoproterenol treatment and display increased cardiac dilation and dysfunction with respect to control mice (16). Similarly, systemic miR-22 knockout mice were found to develop cardiac dilation and dysfunction in response to pressure overload (17). These effects were associated with a downregulation of Serca2a expression and with sarcomere disarray. Interestingly, however, miR-22 gain of function also appears to be maladaptive. Mice with cardiac-specific miR-22 overexpression develop cardiac hypertrophy and dysfunction, and these deleterious effects are associated with impaired calcium handling and reduced expression of PPARα, SIRT1 and PGC-1α (18). Thus, it is likely that proper cardiac function requires that miR-22 expression levels remain within a relatively narrow physiological range.
The molecular mechanism by which miR-22 inhibits autophagy has not yet been fully elucidated. miR-22 was found to inhibit autophagy in cardiomyocytes by reducing the expression of PPARα (11). However, other mechanisms may also be involved in the inhibitory effects of miR-22 on autophagy. It was previously demonstrated that miR-22 reduces the expression of PTEN, a negative regulator of the AKT pathway, in cardiomyocytes (19). This suggests that miR-22 may also suppress autophagy through activation of AKT, which may, in turn, activate the kinase mTOR, a negative regulator of the autophagic process (20). Since miR-22 affects cardiac function through multiple mechanisms, it is also possible that the inhibition of autophagy is indirectly mediated through other functional targets of miR-22, such as the mitochondrial dysfunction and metabolic remodeling observed in hearts following myocardial infarction. Future studies are warranted to test this possibility.
Aside from autophagy, other mechanisms may also underlie the beneficial cardiac effects of pharmacological miR-22 inhibition (Figure 1). Inhibition of the AKT/mTOR pathway may be one of these mechanisms, since there is a large body of evidence demonstrating that chronic and deregulated activation of mTOR is detrimental during cardiac stress, whereas its pharmacological inhibition is protective (20). SIRT1 was also found to be a target of miR-22 (18), so that activation of SIRT1 may represent another mechanism through which miR-22 inhibition may reduce age-related cardiac diseases. We previously found that SIRT1 reduces cardiac aging and confers resistance to oxidative stress (21), and SIRT1 has also been shown to limit ischemic injury (22). Finally, miR-22 inhibition may upregulate PGC-1α, another miR-22 target. PGC-1α controls mitochondrial biogenesis and its upregulation may favor mitochondrial turnover and proper mitochondrial function, which are usually impaired in the old heart (2). In this regard, mice with PGC-1α gene deletion display an impaired energy state and develop contractile dysfunction during aging (23).
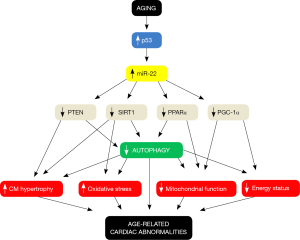
One of the most remarkable results of the study by Gupta et al. is the demonstration that miR-22 dysregulation is relevant to human disease. In fact, the authors found that high circulating levels of miR-22 are associated with a higher cardiovascular mortality in patients affected by heart failure (11). Future studies are warranted to investigate whether miR-22 can be used as a biomarker to monitor the efficacy of pharmacological therapy in heart failure patients. It will be important to evaluate whether the pharmacological inhibition of miR-22 ameliorates symptoms and outcomes in these subjects. Of note, atorvastatin was previously seen to reduce the expression of miR-22 in cardiomyocytes (24) and was also shown to ameliorate heart failure in patients (25).
In conclusion, Gupta et al. provided compelling results indicating that miR-22 upregulation in the heart is responsible for the reduction of autophagy and the increased susceptibility to stress during aging. miR-22 may be considered as a potential therapeutic target for the treatment of age-related diseases (Figure 1).
Acknowledgements
We thank Daniela Zablocki for critical reading of the manuscript.
Funding: This work was supported in part by U.S. Public Health Service Grants HL67724, HL91469, HL102738, HL112330 and AG23039 (J Sadoshima) and by the Leducq Foundation Transatlantic Network of Excellence (J Sadoshima). This work was also supported by a grant from the Italian Ministry of Health (GR-201302355401) to S Sciarretta.
Footnote
Conflicts of Interest: The authors have no conflicts of interest to declare.
References
- Writing Group Members., Mozaffarian D, Benjamin EJ, et al. Heart Disease and Stroke Statistics-2016 Update: A Report From the American Heart Association. Circulation 2016;133:e38-360. [Crossref] [PubMed]
- Shirakabe A, Ikeda Y, Sciarretta S, et al. Aging and Autophagy in the Heart. Circ Res 2016;118:1563-76. [Crossref] [PubMed]
- Levine B, Kroemer G. Autophagy in the pathogenesis of disease. Cell 2008;132:27-42. [Crossref] [PubMed]
- Eisenberg T, Abdellatif M, Schroeder S, et al. Cardioprotection and lifespan extension by the natural polyamine spermidine. Nat Med 2016;22:1428-38. [Crossref] [PubMed]
- Nakai A, Yamaguchi O, Takeda T, et al. The role of autophagy in cardiomyocytes in the basal state and in response to hemodynamic stress. Nat Med 2007;13:619-24. [Crossref] [PubMed]
- Shirakabe A, Zhai P, Ikeda Y, et al. Drp1-Dependent Mitochondrial Autophagy Plays a Protective Role Against Pressure Overload-Induced Mitochondrial Dysfunction and Heart Failure. Circulation 2016;133:1249-63. [Crossref] [PubMed]
- Maejima Y, Kyoi S, Zhai P, et al. Mst1 inhibits autophagy by promoting the interaction between Beclin1 and Bcl-2. Nat Med 2013;19:1478-88. [Crossref] [PubMed]
- Sciarretta S, Boppana VS, Umapathi M, et al. Boosting autophagy in the diabetic heart: a translational perspective. Cardiovasc Diagn Ther 2015;5:394-402. [PubMed]
- Sciarretta S, Zhai P, Shao D, et al. Rheb is a critical regulator of autophagy during myocardial ischemia: pathophysiological implications in obesity and metabolic syndrome. Circulation 2012;125:1134-46. [Crossref] [PubMed]
- Matsui Y, Takagi H, Qu X, et al. Distinct roles of autophagy in the heart during ischemia and reperfusion: roles of AMP-activated protein kinase and Beclin 1 in mediating autophagy. Circ Res 2007;100:914-22. [Crossref] [PubMed]
- Gupta SK, Foinquinos A, Thum S, et al. Preclinical Development of a MicroRNA-Based Therapy for Elderly Patients With Myocardial Infarction. J Am Coll Cardiol 2016;68:1557-71. [Crossref] [PubMed]
- Shvets E, Fass E, Elazar Z. Utilizing flow cytometry to monitor autophagy in living mammalian cells. Autophagy 2008;4:621-8. [Crossref] [PubMed]
- Jazbutyte V, Fiedler J, Kneitz S, et al. MicroRNA-22 increases senescence and activates cardiac fibroblasts in the aging heart. Age (Dordr) 2013;35:747-62. [Crossref] [PubMed]
- Ucar A, Gupta SK, Fiedler J, et al. The miRNA-212/132 family regulates both cardiac hypertrophy and cardiomyocyte autophagy. Nat Commun 2012;3:1078. [Crossref] [PubMed]
- Higashi K, Yamada Y, Minatoguchi S, et al. MicroRNA-145 repairs infarcted myocardium by accelerating cardiomyocyte autophagy. Am J Physiol Heart Circ Physiol 2015;309:H1813-26. [Crossref] [PubMed]
- Huang ZP, Chen J, Seok HY, et al. MicroRNA-22 regulates cardiac hypertrophy and remodeling in response to stress. Circ Res 2013;112:1234-43. [Crossref] [PubMed]
- Gurha P, Abreu-Goodger C, Wang T, et al. Targeted deletion of microRNA-22 promotes stress-induced cardiac dilation and contractile dysfunction. Circulation 2012;125:2751-61. [Crossref] [PubMed]
- Gurha P, Wang T, Larimore AH, et al. microRNA-22 promotes heart failure through coordinate suppression of PPAR/ERR-nuclear hormone receptor transcription. PLoS One 2013;8:e75882. [Crossref] [PubMed]
- Xu XD, Song XW, Li Q, et al. Attenuation of microRNA-22 derepressed PTEN to effectively protect rat cardiomyocytes from hypertrophy. J Cell Physiol 2012;227:1391-8. [Crossref] [PubMed]
- Sciarretta S, Volpe M, Sadoshima J. Mammalian target of rapamycin signaling in cardiac physiology and disease. Circ Res 2014;114:549-64. [Crossref] [PubMed]
- Alcendor RR, Gao S, Zhai P, et al. Sirt1 regulates aging and resistance to oxidative stress in the heart. Circ Res 2007;100:1512-21. [Crossref] [PubMed]
- Hsu CP, Zhai P, Yamamoto T, et al. Silent information regulator 1 protects the heart from ischemia/reperfusion. Circulation 2010;122:2170-82. [Crossref] [PubMed]
- Arany Z, He H, Lin J, et al. Transcriptional coactivator PGC-1 alpha controls the energy state and contractile function of cardiac muscle. Cell Metab 2005;1:259-71. [Crossref] [PubMed]
- Tu Y, Wan L, Bu L, et al. MicroRNA-22 downregulation by atorvastatin in a mouse model of cardiac hypertrophy: a new mechanism for antihypertrophic intervention. Cell Physiol Biochem 2013;31:997-1008. [Crossref] [PubMed]
- Sola S, Mir MQ, Lerakis S, et al. Atorvastatin improves left ventricular systolic function and serum markers of inflammation in nonischemic heart failure. J Am Coll Cardiol 2006;47:332-7. [Crossref] [PubMed]