Advanced glycation end products (AGEs) downregulate the miR-4429/PTEN axis to promote apoptosis of fibroblasts in pelvic organ prolapse
Introduction
Pelvic organ prolapse (POP) is defined as protrusions of the pelvic organs into or out of the vaginal canal; it has a relatively high prevalence worldwide and markedly diminishes the quality of life of older women (1). The prevalence of POP based on diagnosis according to symptoms is 3–6%; however, the prevalence according to physical examination is up to 50% (2). The most recent report has stated that the prevalence of symptomatic POP in China is 9.6% among women over 20 years old (3). It is a multifactorial disease, and the specific pathogenesis remains to be elucidated, which has impeded the development of treatment and prevention. Research is urgently needed to define the molecular process in the pathogenesis of POP.
Advanced glycation end products (AGEs) are produced by the nonenzymatic modifications of macromolecules, including proteins, lipids, and nucleic acids. The endogenous formation of AGEs is a long-term physiological process, but the process accelerates if glucose concentration or reactive oxygen species (ROS) are elevated (4,5). A previous study showed that the level of AGEs in prolapsed tissues was higher and the collagen I level was lower than that of control tissues (6). Further, we reported that AGEs accelerated the degeneration of collagen I by upregulating the expression of matrix metallopeptidase-1 (MMP-1) and by activating the mitogen-activated protein kinase (MAPK)/nuclear factor-kappa B (NF-Kb) pathway in human vaginal fibroblasts (7). In addition to results from other previous studies (8,9), our previous findings support the notion that the increased level of AGEs in POP tissues could contribute to the pathogenesis of POP. However, the molecular mechanism of AGEs in the pathophysiology of POP remains to be further explored.
Fibroblasts regulate the processes of extracellular matrix (ECM) formation and remodeling (10,11), and cellular functional alterations of fibroblasts have been the research focus in exploring POP pathogenesis. As the primary functional cell type in pelvic supportive tissues, fibroblasts exhibit impaired behaviors and functions in prolapsed tissues, resulting in decreased collagen fibers and insufficient mechanical support to the pelvic organs (12-15). For this reason, many studies have tried to find new therapeutic avenues for POP by promoting the functions of fibroblasts (16,17). Several studies have reported increased fibroblast apoptosis in POP (18,19), but few studies have focused on the underlying mechanism. The AGEs have been investigated mainly in the pathophysiology of diabetes mellitus (20); few studies have reported the effects and the related mechanisms of AGEs on fibroblasts in POP. The increased level of AGEs in pelvic floor supportive tissues in patients with POP suggests that exploring the molecular processes underlying AGEs-induced functional alterations of fibroblasts is critical to understanding POP pathophysiology and developing novel treatments by inhibiting AGEs formation.
Phosphatase and tensin homolog (PTEN) has been investigated as a classical tumor suppressor because it antagonizes phosphoinositide 3-kinase (PI3K) (21). This inactivates downstream protein kinases, most notably protein kinase B (Akt), which influences downstream proteins and alters cellular functions (22). It has been investigated as a regulator of cell apoptosis (23), and PTEN has been found to induce apoptosis by antagonizing the PI3K-Akt pathway in physiologic and pathologic conditions (24-26). Studies have also reported that the expression of PTEN could be regulated by microRNAs (miRNAs) (27,28). In the present study, we searched for miRNAs that potentially target PTEN in databases and found that miR-4429 was the regulator of PTEN in AGEs-induced fibroblast apoptosis. We chose miR-4429 for further investigation because previous studies had reported that miR-4429 regulated cell apoptosis and prevented cancer progression (29,30).
Our investigation aimed to explore the molecular mechanisms underlying the phenomenon that AGEs promoted apoptosis of uterosacral ligament fibroblasts. We reported that fibroblasts from the POP group exhibited a higher apoptosis rate than those from the non-POP group. Considering the accumulated AGEs in POP tissues, we treated fibroblasts with AGEs in vitro and found increased apoptosis rates in POP and non-POP groups. Further, we detected the expression of PTEN and miR-4429 in POP and non-POP groups with or without AGEs treatment. Then, we analyzed the apoptosis rates of fibroblasts by overexpressing miR-4429 or silencing the expression of PTEN. These results demonstrated that AGEs promoted fibroblast apoptosis by influencing the miR-4429/PTEN/PI3K/Akt pathway. This study provides evidence for the molecular effects of accumulated AGEs in POP. We present the following article in accordance with the Material Design Analysis Reporting (MDAR) reporting checklist (available at https://atm.amegroups.com/article/view/10.21037/atm-22-628/rc).
Methods
Patients and sample collection
Tissue samples were obtained from patients who had POP or other diseases and required a hysterectomy at the Obstetrics and Gynecology Hospital of Fudan University. The study was conducted in accordance with the Declaration of Helsinki (as revised in 2013). The study was approved by the Ethics Committee of the Obstetrics and Gynecology Hospital of Fudan University (No. 2021-234), and informed consent was provided by all participants. A total of 10 participants with stage III or IV POP (according to the pelvic organ prolapsed quantification; POP-Q) and 10 participants who underwent hysterectomy for diseases including hysteromyoma, adenomyosis, and high-grade squamous intraepithelial lesion, were respectively distributed to the POP group and the non-POP group. Donors did not have histories of pelvic operations, pelvic inflammation, serious systemic diseases, and malignant diseases. The clinical characteristics, including age, body mass index, parity, and menopausal status, were matched between the two groups (Table 1).
Table 1
Characteristic | POP (n=10) | Non-POP (n=10) | P value |
---|---|---|---|
Age, mean ± SD | 61.4±5.70 | 53.6±8.62 | NS‡ |
BMI, mean ± SD | 23.83±6.02 | 23.9±3.62 | NS† |
Parity, median [range] | 1 [1–3] | 1 [1–2] | NS‡ |
Menopause, n (%) | 8 (80.0) | 3 (30.0) | NS§ |
POP stage, median [range] | 3 [3–4] | 0 | <0.05‡ |
†, t-test; ‡, Mann-Whitney test; §, Fisher’s exact test. POP, pelvic organ prolapse; SD, standard deviation; NS, not significant; BMI, body mass index.
A 0.5 cm2 piece of uterosacral ligament tissue was collected from the surgical margin of the free uterus after hysterectomy. The sample tissues were kept under aseptic conditions and prepared for cell culture.
Cell culture
Human uterosacral ligament fibroblasts were isolated and cultured as a previously indicated (7). Fresh uterosacral ligament specimens were washed 3 times with phosphate-buffered saline (PBS) (containing 100 IU/mL streptomycin-penicillin-amphotericin B) (Genom, Jiaxing, China) and cut into small pieces. The fragments of tissues were digested at 37 ℃ for 2 hours in PBS containing 2% collagenase type I (Sigma-Aldrich, St. Louis, MO, USA) until the tissue blocks disappeared. After separation by centrifuge, the cells were resuspended and cultured in Dulbecco’s modified Eagle medium (DMEM) nutrient solution with 10% fetal bovine serum (FBS) and 100 IU/mL streptomycin-penicillin-amphotericin B at 37 ℃ in a 5% CO2 atmosphere. The medium was replaced by a fresh complete culture medium every 2–3 days, and cells were passaged when they reached an 80% confluence.
Cell identification
The fibroblasts were seeded on coverslips prepared in 6-well plates. After 12 hours of culture, cells grew on the glass coverslips. Then, 4% paraformaldehyde (Servicebio, Wuhan, China) was added to the 6-well plates for cell fixation. For cell immunochemistry, drops of 3% bovine serum albumin (BSA) were added onto the coverslips and kept at room temperature for 30 minutes. The liquid was discarded. The cells were then incubated with the diluted primary antibodies at 4 ℃ for more than 10 hours and with the secondary antibody (G1213, Servicebio) for 1 hour at room temperature. The primary antibodies included anti-Cytokeratin antibody (GB11197, Servicebio) and anti-Vimentin antibody [5741, Cell Signaling Technology (CST), Danvers, MA, USA]. After being washed 3 times in PBS, slides were stained with 3,3’-diaminobenzidine (DAB) color developing solution (Servicebio) and then with hematoxylin stain solution (Servicebio) to counterstain the nucleus. Images were observed under a light microscope (Olympus, Tokyo, Japan).
Cell treatments
The AGEs used in the study were diluted with 10 mg/mL BSA-AGE, which was purchased from Abcam (Cambridge, MA, USA). The AGE-modified BSA was produced by reacting BSA with glycolaldehyde under sterile conditions, followed by extensive dialysis and purification steps. Fluorescence of AGEs was confirmed by fluorescence spectrophotometry with Ex./Em. =370/440 nm. Glycated BSA showed a 7,000% increase in fluorescence compared to control BSA. The 3–5 passage fibroblasts were cultured and serum-starved for 12 hours. Then, fibroblasts from both the POP and non-POP groups were exposed to 50 µg/mL BSA (Sigma-Aldrich) or 50 µg/mL BSA-AGE for 24 hours, according to our previous report (7).
Quantitative polymerase chain reaction
The total RNA of cultured cells was extracted using an RNA purification kit (EZBioscience, Roseville, CA, USA). The extracted RNA was converted into complementary DNA (cDNA) through reverse transcription using a cDNA synthesis kit (TaKaRa, Tokyo, Japan). The expressions of target genes at the messenger RNA (mRNA) level were measured through a real-time polymerase chain reaction (RT-PCR) analysis using the SYBR® Green qPCR master mix (TaKaRa). The miRNA cDNAs were synthesized using a microRNA reverse transcription kit (EZBioscience), and miRNA were relatively quantified using a quantitative (q)PCR mix for microRNA (EZBioscience). The qPCR of miRNAs and mRNA were done by the QuantStudio 6 and 7 Flex Real-Time PCR System (Thermo Fisher Scientific, Waltham, MA, USA). The reference genes for mRNA and miRNA were respectively glyceraldehyde 3-phosphate dehydrogenase (GAPDH) and U6. Moreover, results were normalized by the 2−ΔΔCT method. The primer sequences in this study are listed in Table 2.
Table 2
Name | Sequence (5' to 3') |
---|---|
Caspase-3 forward | CCAAAGATCATACATGGAAGCG |
Caspase-3 reverse | CTGAATGTTTCCCTGAGGTTTG |
PTEN forward | TTTTGAAGACCATAACCCAC |
PTEN reverse | TATCATTACACCAGTTCGTC |
snRNA U6 | CCTGCTTCGGCAGCACA |
miR-23b-3p | CACATTGCCAGGGATTACCA |
miR-542 | GGGCGTGACAGATTGATAACTG |
miR-4429 | CAAAAGCTGGGCTGAGAGG |
miR-216b-5p | AAATCTCTCCAGGCAAATGTGA |
miR-4429 mimics | AAAAGCUGGGCUGAGAGGCG |
mimics-NC | UCACAACCUCCUAGAAAGAGUAGA |
PTEN-siRNA 1 | AGCUAAAGGUGAAGAUAUATT |
PTEN-siRNA 2 | CAGAUAAUGACAAGGAAUATT |
PTEN-siRNA 3 | CAAAUAAAGACAAAGCCAATT |
siRNA, small interfering RNA; PTEN, phosphatase and tensin homolog; snRNA U6, small nuclear RNA U6; NC, negative control.
Flow cytometric analysis
The apoptosis of fibroblasts was detected using a fluorescein isothiocyanate (FITC) Annexin V/propidium iodide (PI) kit [Becton, Dickinson, and Company (BD) Biosciences, San Jose, CA, USA] with flow cytometric analysis. Fibroblasts were detached and washed with PBS supplemented with 2% FBS after the indicated treatment. Fibroblasts were simultaneously stained with FITC-Annexin V and PI for 15 and 5 minutes in the dark at room temperature. After being washed twice, cells were resuspended in 300 mL buffer and passed through a 100-mm nylon sieve. A flow cytometry (BD, Franklin Lakes, NJ, USA) was used to analyze the cell samples, by which the normal cells (FITC−/PI−), early to mid-apoptotic cells (FITC+/PI−), and late apoptotic cells (FITC+/PI+) were distinguished. Data were analyzed using FlowJo version X.0.7 (FlowJo, BD, Ashland, OR, USA). Positive cells were identified according to the fluorescence values of the unstained cells, which were set as negative controls (NC).
Transfection of miR-4429 mimics and PTEN-siRNA
Fibroblasts were seeded in 6-well culture plates with the optimum density in DMEM solution with 10% FBS. Once achieving 50–60% confluence, the medium was replaced by 1.5 mL DMEM per well without FBS. Then, miR-4429 mimics or NC mimics were diluted by Opti-MEM (GIBCO BRL, Grand Island, NY, USA) and mixed with diluted Lipofectamine 2000 (Thermo Fisher Scientific, USA) (5 mL lipo2000 in 250 mL Opti-MEM). The mixture was carefully transferred to DMEM solutions without FBS to achieve a final concentration of mimics of 200 nM. The transfection method of small interfering (si)PTEN was the same as miR-4429 mimics, except that the final concentration of siPTEN was 50 nM. After 6 hours of incubation, the culture medium was changed into DMEM with 10% FBS and continued to culture for 24–48 hours. The sequences of miR-4429 mimics, mimics-NC, and PTEN-siRNAs (Table 2) were synthesized by the Public Protein/Plasmid Library (Nanjing, China).
miRNAs screening
To collect miRNAs that potentially interacted with PTEN, we searched the following miRNAs databases: starBase (starbase.sysu.edu.cn/starbase2/), TargetScan (www.targetscan.org/vert_71/), miRDB (mirdb.org), and miRWalk (miRWalk.umm.uni-heidelberg.de). A total of 376 miRNAs were selected from miRDB, 104 from starBase, 1,165 from TargetScan, and 1,589 from miRWalk (Table S1). Then, 24 miRNAs were screened out by taking the intersection of the 4 datasets (Table S2).
Western blot analysis
Protein expressions were semi-quantitatively analyzed by western blot. Radioimmunoprecipitation assay (RIPA) lysis buffer (Beyotime, Shanghai, China) was added to cell cultures, and proteins were collected to be prepared for gel electrophoresis. The loading buffer and the reagents for preparing gels were purchased from Beyotime. Then, proteins were isolated by gel electrophoresis and transferred to the polyvinylidene fluoride (PVDF) membranes, which were blocked by being incubated with 5% BSA (Sigma-Aldrich) or 5% nonfat-dried milk/tris-buffered saline with Tween 20 (TBST) for 1 hour at room temperature. Successively, PVDF membranes were gently shaken with the primary antibodies at 4 ℃ for more than 10 hours and with the secondary antibody for 1 hour at room temperature. The antibodies included anti-pan-Akt antibody, anti-phosphorylated Akt (S473) antibody (CST), anti-PI3K antibody, and anti-GAPDH antibody (Abcam). After washing the membranes 3 times with TBST in the interval of 2 incubations, the membranes were incubated with the chemiluminescence reagent (Merck Millipore, Billerica, MA, USA).
Luciferase reporter assay
A DNA fragment of the miR-4429 potential binding site in the 3’untranslated region (3’UTR) of PTEN was cloned into a psiCHECK-2 vector (Hanbio Biotechnology, Shanghai, China), named PTEN-wild-type (PTEN-WT). A 500 bp mutational type of all the 5 miR-4429 potential binding sites in 3’UTR of PTEN was cloned into a psiCHECK-2 vector, named PTEN-mutant (PTEN-Mut). A 500 bp DNA fragment of the miR-4429 potential binding site in 3’UTR of PTEN was cloned in a psiCHECK-2 vector, named PTEN-mutant1 (PTEN-Mut1). The 293T cell line was purchased from the Shanghai Branch of the Chinese Academy of Science. Cells were transferred into a 96-well plate and prepared for transfection once achieving 50–70% confluence. Then, 0.16 µg plasmid, 5 pmol miR-4429 mimics or NC mimics, and transfection reagent (Hanbio Biotechnology) were mixed and added to each well. After 48 hours of incubation, cells were collected, and the luciferase activities of each group were detected by a Dual-Luciferase Reporter Assay kit (Promega, Madison, WI, USA).
Statistical analysis
Data were presented as the mean ± standard deviation (SD). The student’s t-test was used to determine the significant difference between 2 groups when the data were normally distributed with equal SDs. The Mann-Whitney test was used for the 2-group comparison when the data were not normally distributed. Data were subjected to analysis of variance (ANOVA) when determining the significant difference between 3 or more groups, and the Tukey test was used to separate the means. Differences between groups were considered statistically significant when P<0.05. All tests involved in the study were performed by the GraphPad Prism software (version 8.0.2; GraphPad Software Inc., San Diego, CA, USA).
Results
AGEs promoted fibroblast apoptosis in the POP and the non-POP groups
Primary cultured fibroblasts were identified by the positive expression of vimentin and the negative expression of keratin (Figure 1). The apoptosis rate was higher in fibroblasts from the POP group than in the non-POP group (Figure 2A). An increased gene expression of caspase-3 was observed in the POP group compared with the non-POP group (Figure 2B). The apoptosis rate was significantly increased when fibroblasts from the non-POP group were treated with AGEs compared with the untreated cells (Figure 2C). To further verify that the increase of fibroblast apoptosis in POP and AGEs promote apoptosis of fibroblasts, the mRNA expression of caspase-3 was relatively quantified. The relative expression of caspase-3 was upregulated in non-POP fibroblasts treated with AGEs compared to those untreated cells (Figure 2D). Similarly, the apoptosis rate was increased significantly when fibroblasts from the POP group were treated with AGEs compared with the untreated cells (Figure 2E). Further, the expression of caspase-3 was upregulated in POP fibroblasts treated with AGEs compared to those untreated cells (Figure 2F).
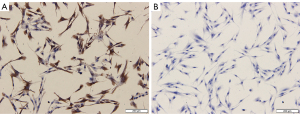
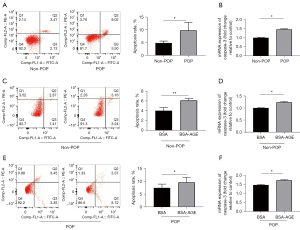
AGEs upregulated the expression of PTEN in fibroblasts from the POP and the non-POP groups
The mRNA expression level (Figure 3A) and the protein expression level of PTEN (Figure 3B) were higher in fibroblasts from the POP group than in the non-POP group. In fibroblasts from the non-POP groups, the mRNA expression level (Figure 3C) and the protein expression level of PTEN (Figure 3D) were upregulated when treated with AGEs. Likewise, in fibroblasts from the POP groups, the mRNA expression level (Figure 3E) and the protein expression level of PTEN (Figure 3F) were upregulated when treated with AGEs. Further, the western blot analysis demonstrated a reduction in the protein level of PI3K and phosphorylated Akt in fibroblasts treated by AGEs (Figure 3G), indicating that AGEs inhibited the PI3K/Akt pathway.
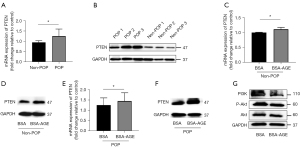
MiR-4429 directly bound to PTEN and inhibited the expression of PTEN
To further investigate the mechanism of AGE upregulating the expression of PTEN, we found 24 potential miRNAs that had binding sites on the 3’UTR of PTEN by taking the intersection of 4 recognized online databases (Figure 4A). The miRNAs analyzed in the study can be found in Tables S1,S2. Among the 24 potential miRNAs, the expression of miR-542, miR-4429, miR-216, and miR-23b was verified by qPCR, and the down-regulation effect of AGEs on the expression of miR-4429 was the most significant among the 4 miRNAs (Figure 4B).
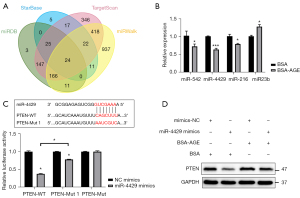
The luciferase activity of the PTEN-WT reporter was lower in the miR-4429 mimics group than in the NC mimics group. The luciferase activity of the PTEN-Mut 1 reporter was lower in the miR-4429 mimics group than that in the NC mimics group, but it was higher in the miR-4429 mimics group than that in the PTEN-WT + miR-4429 mimics group. Further, there was no difference in luciferase activities of PTEN-Mut reporter between the miR-4429 mimics group, and the NC mimics group (Figure 4C). These results confirmed that miR-4429 could bind to the 3’UTR of PTEN and downregulate the expression of PTEN, but the binding site shown in Figure 3C was not the only binding site between miR-4429 and mRNA of PTEN.
The western blotting analysis showed that overexpression of miR-4429 in fibroblasts reduced the protein expression of PTEN (Figure 4D). The AGEs treatment on fibroblasts increased the protein expression of PTEN, which could be attenuated by overexpression of miR-4429 in fibroblasts (Figure 4D). These results suggested that miR-4429 inhibited the expression of PTEN, and AGEs upregulated the expression of PTEN by miR-4429.
AGEs reduced the expression of miR-4429 both in the non-POP and the POP groups
The expressions of miR-4429 were measured in 10 POP and 10 non-POP-derived uterosacral ligament fibroblasts, and the expression level was lower in the POP group than in the non-POP group (Figure 5A). Following the treatment of AGEs, fibroblasts exhibited a reduced miR-4429 expression in the POP (Figure 5B) and the non-POP (Figure 5C) groups compared with the control cells treated with BSA.
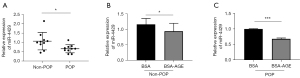
AGEs promoted fibroblast apoptosis by downregulating the miR-4429/PTEN axis
In light of the reduced expression of miR-4429 and the increased expression of PTEN in fibroblasts treated with AGEs, we then verified that AGEs promoted fibroblast apoptosis by downregulating the miR-4429/PTEN axis. The results showed that cells transfected with siPTEN had a reduced protein level of PTEN (Figure 6A), indicating that the cell transfection had succeeded. The expression of miR-4429 was not influenced by the cell transfection with siPTEN (Figure 6B). The apoptosis analysis revealed that overexpression of miR-4429 decreased the fibroblast apoptosis rate and inhibited the apoptosis-promoting effect of AGEs on fibroblasts (Figure 6C). In the same way, transfection of siPTEN decreased the fibroblast apoptosis rate and inhibited the apoptosis-promoting effect of AGEs on fibroblasts (Figure 6D). These results suggested that AGEs promoted fibroblast apoptosis by downregulating the expression of miR-4429 and upregulating the expression of PTEN. To further verify the results, the expression of apoptosis-related gene caspase-3 was detected by qPCR. Further, we found that the overexpression of miR-4429 could reduce the elevated expression of caspase-3 induced by AGEs on fibroblasts (Figure 6E). Similarly, transfection of siPTEN could reduce the elevated expression of caspase-3 induced by AGEs on fibroblasts (Figure 6F).
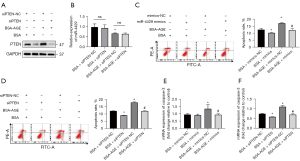
Discussion
This study demonstrated that AGEs promoted uterosacral ligament fibroblast apoptosis by downregulating miR-4429 which interacted with PTEN mRNA to inhibit the protein expression. In addition, we speculated that the increased PTEN induced by AGEs might promote cell apoptosis by suppressing the function of the PI3K/Akt pathway. The study revealed a possible mechanism through which AGEs induced fibroblast apoptosis in POP (Figure 7). Our findings provide evidence that the accumulation of AGEs contributes to the development of POP; AGEs could be a latent treatment point of future therapy for POP.
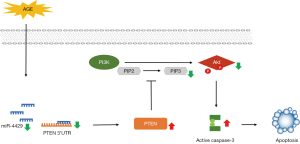
In this study, we verified our previous conclusion that the content of AGEs was increased in prolapse tissues compared to controls (Figure S1). The methods can be found in the Appendix 1. Although studies had shown that AGEs accumulated in prolapse tissues (8,9), the cause of this phenomenon was unknown. Apart from the hyperglycemic environment, the formation process of AGEs also accelerates under chronic inflammation or oxidative stress conditions (31,32). Previous studies have reported that ROS increase in POP (33,34), interfering with multiple cellular processes and favoring POP development. Thus, we speculate that the high level of AGEs in the prolapse tissues could result from increased ROS in POP because the formation of AGEs accelerates under the elevated ROS level in prolapse tissues (35).
Our previous results showed that AGEs increased the apoptosis rate in fibroblasts from the prolapsed vaginal wall (36), but the underlying mechanism was not studied. In the present study, we found that the cell apoptosis rate and caspase-3 (37) expression of fibroblasts from the POP group were higher than in the non-POP group. Caspase-3 is a recognised biochemical hallmark for apoptosis induced by different apoptotic signals (38). Previous studies have reported the elevated cell apoptosis rate of the uterosacral ligament in POP by immunohistochemical or terminal deoxynucleotidyl transferase Dutp nick end labeling (TUNEL) staining (19,33,39), consistent with the present results. However, few studies have analyzed the apoptosis of cultured fibroblasts from POP and non-POP patients. These results suggested that the aggravated fibroblast apoptosis was a pathological alteration in POP and embodied the clinical significance of investigating fibroblast apoptosis. Fibroblasts are responsible for the secretion and remodeling of ECM in ECM-rich pelvic connective tissues that primarily support the pelvic organs. The disfunction of fibroblasts resulted in a disordered metabolism of collagen and other ECM proteins (40-42). Therefore, the increased fibroblast apoptosis could result in reduced collagen expression in POP and contribute to the weakness of supportive tissues in POP (40,43). In the present study, AGEs promoted the apoptosis of fibroblasts from the non-POP group and aggregated the apoptosis of fibroblasts from the POP group, suggesting that AGEs might be a pathogenesis factor that induced the fibroblast apoptosis in POP.
In this study, we began with a finding that AGEs promoted fibroblast apoptosis. Then, we focused on PTEN, which is recognized as a regulator of cell apoptosis (23) and was previously unreported in the molecular alterations in POP. Our results suggested that the expression of PTEN increased in POP as opposed to non-POP fibroblasts, which was consistent with cell apoptosis results. Further, the increased apoptosis rate was alleviated after silencing the expression of PTEN. These results suggested that PTEN played an essential role in fibroblast apoptosis in POP. In addition, the effect of PTEN on apoptosis in this study was consistent with the previous reports. They all showed that the changing trend of PTEN expression was in line with the apoptosis rate (44,45).
miRNAs are small non-coding RNAs that regulate gene expression at the post-transcriptional stage by interacting with target mRNAs at 3’UTR (46). Many researchers have previously reported that miRNAs might be implicated in developing AGEs-related diseases and interfere with the AGE/receptor for IAGE signaling. For instance, the expression levels among a series of miRNAs were reported to be regulated by AGEs (47-49). Moreover, Piperi et al. (50) reviewed miRNAs interacting with the AGE/RAGE signaling and the diabetic complications. To determine whether AGEs upregulated the expression of PTEN by miRNAs, we screened out 24 miRNAs with potential binding sites to PTEN using bioinformatics prediction tools and verified 4 of the 24 miRNAs. The results showed that the expression level of miR-4429 was lower in the POP group than in the non-POP group, and AGEs downregulated the expression of miR-4429 both in POP and non-POP fibroblasts.
Moreover, miR-4429 was shown to combine with the 3'UTR of PTEN directly and downregulate the expression of PTEN. The function of miR-4429 was previously mainly explored in cancer cells and was reported to prevent the progression of several cancers (30,51). However, no study has reported that AGEs could regulate miR-4429, and no study has shown miR-4429 could bind with mRNA of PTEN and downregulate the expression of PTEN. The AGEs induced apoptosis of fibroblasts, but this could be alleviated by overexpression of miR-4429, which could also almost be neutralized by silencing PTEN expression. Further, the expression of miR-4429 was only influenced by AGEs exposure but not by the transfection of siPTEN. Taken together, we showed that AGEs induced fibroblast apoptosis through the miR-4429/PTEN axis.
Further, the protein expression of total Akt was not changed, while the phosphorylated Akt was downregulated when fibroblasts were treated with AGEs. This result demonstrated that AGEs-induced upregulation of PTEN indeed antagonized the PI3K/Akt pathway, in line with the well-known function of PTEN (52). As a lipid phosphatase, PTEN negatively regulated phosphatidylinositol-3,4,5-triphosphate-dependent Akt signaling to inhibit growth, protein synthesis, cell cycle progression, metabolism, and apoptosis (53). The only difference was that the expression of PI3K was reduced under the treatment of AGEs, which implied that the inhibition of AGEs on the PI3K/Akt pathway was not only regulated by the miR-4429/PTEN axis. The PI3K/Akt pathway regulated cell viability and proliferation (54,55), and the inhibition of this pathway logically promoted cell apoptosis (56,57). Further, several studies have reported that upregulated miRNAs targeted PTEN and activated the PI3K/Akt pathway to promote cell proliferation and inhibit apoptosis (58,59).
In conclusion, we demonstrated that AGEs induced fibroblast apoptosis by regulating the miR-4429/PTEN/PI3K/Akt pathway in POP. The AGEs reduced the expression of miR-4429, thereby increasing the expression of PTEN and promoting fibroblast apoptosis. In addition, PI3K/Akt was inhibited by AGEs, which caused increased cell apoptosis.
Acknowledgments
We acknowledge the work of Peng Li and Suna Tian from Hubei Institute of Fine Arts, who contributed to optimizing the images.
Funding: This work was supported by the National Natural Science Foundation of China (No. 81671439 to YC), the Science and Technology Commission of Shanghai Municipality 2018 YIXUEYINGDAO project (No. 18401902200 to LW), the Science and Technology Innovation Action Plan of Shanghai Natural Science (No. 20ZR1409100 to LW), and the Chinese Association of Integration of Traditional and Western Medicine special foundation for Obstetrics and Gynecology-PuZheng Pharmaceutical Foundation (No. FCK-PZ-08 to LW).
Footnote
Reporting Checklist: The authors have completed the MDAR reporting checklist. Available at https://atm.amegroups.com/article/view/10.21037/atm-22-628/rc
Data Sharing Statement: Available at https://atm.amegroups.com/article/view/10.21037/atm-22-628/dss
Peer Review File: Available at https://atm.amegroups.com/article/view/10.21037/atm-22-628/prf
Conflicts of Interest: All authors have completed the ICMJE uniform disclosure form (available at https://atm.amegroups.com/article/view/10.21037/atm-22-628/coif). The authors have no conflicts of interest to declare.
Ethical Statement: The authors are accountable for all aspects of the work in ensuring that questions related to the accuracy or integrity of any part of the work are appropriately investigated and resolved. The study was conducted in accordance with the Declaration of Helsinki (as revised in 2013). The study was approved by the Ethics Committee of the Obstetrics and Gynecology Hospital of Fudan University (No. 2021-234) and informed consent was taken from all the patients.
Open Access Statement: This is an Open Access article distributed in accordance with the Creative Commons Attribution-NonCommercial-NoDerivs 4.0 International License (CC BY-NC-ND 4.0), which permits the non-commercial replication and distribution of the article with the strict proviso that no changes or edits are made and the original work is properly cited (including links to both the formal publication through the relevant DOI and the license). See: https://creativecommons.org/licenses/by-nc-nd/4.0/.
References
- Swift S, Woodman P. Pelvic Organ Support Study (POSST): the distribution, clinical definition, and epidemiologic condition of pelvic organ support defects. Am J Obstet Gynecol 2005;192:795-806. [Crossref] [PubMed]
- Wu JM, Vaughan CP, Goode PS, et al. Prevalence and trends of symptomatic pelvic floor disorders in U.S. women. Obstet Gynecol 2014;123:141-8. [Crossref] [PubMed]
- Pang H, Zhang L, Han S, et al. A nationwide population-based survey on the prevalence and risk factors of symptomatic pelvic organ prolapse in adult women in Chi–a - a pelvic organ prolapse quantification system-based study. BJOG 2021;128:1313-23. [Crossref] [PubMed]
- Wang Y, Luo W, Han J, et al. MD2 activation by direct AGE interaction drives inflammatory diabetic cardiomyopathy. Nat Commun 2020;11:2148. [Crossref] [PubMed]
- Uribarri J, del Castillo MD, de la Maza MP, et al. Dietary advanced glycation end products and their role in health and disease. Adv Nutr 2015;6:461-73. [Crossref] [PubMed]
- Chen Y, Huang J, Hu C, et al. Relationship of advanced glycation end products and their receptor to pelvic organ prolapse. Int J Clin Exp Pathol 2015;8:2288-99. [PubMed]
- Chen YS, Wang XJ, Feng W, et al. Advanced glycation end products decrease collagen I levels in fibroblasts from the vaginal wall of patients with POP via the RAGE, MAPK and nf-κB pathways. Int J Mol Med 2017;40:987-98. [Crossref] [PubMed]
- Sferra R, Pompili S. Neurovascular alterations of muscularis propria in the human anterior vaginal wall in pelvic organ prolapse. J Anat 2019;235:281-8. [Crossref] [PubMed]
- Vetuschi A, Pompili S, Gallone A, et al. Immunolocalization of Advanced Glycation End Products, Mitogen Activated Protein Kinases, and Transforming Growth Factor-β/Smads in Pelvic Organ Prolapse. J Histochem Cytochem 2018;66:673-86. [Crossref] [PubMed]
- Lu P, Takai K, Weaver VM, et al. Extracellular matrix degradation and remodeling in development and disease. Cold Spring Harb Perspect Biol 2011;3:a005058. [Crossref] [PubMed]
- Plikus MV, Wang X, Sinha S, et al. Fibroblasts: Origins, definitions, and functions in health and disease. Cell 2021;184:3852-72. [Crossref] [PubMed]
- Wang S, Zhang Z, Lü D, et al. Effects of mechanical stretching on the morphology and cytoskeleton of vaginal fibroblasts from women with pelvic organ prolapse. Int J Mol Sci 2015;16:9406-19. [Crossref] [PubMed]
- Ruiz-Zapata AM, Kerkhof MH, Zandieh-Doulabi B, et al. Functional characteristics of vaginal fibroblastic cells from premenopausal women with pelvic organ prolapse. Mol Hum Reprod 2014;20:1135-43. [Crossref] [PubMed]
- Ruiz-Zapata AM, Kerkhof MH, Ghazanfari S, et al. Vaginal Fibroblastic Cells from Women with Pelvic Organ Prolapse Produce Matrices with Increased Stiffness and Collagen Content. Sci Rep 2016;6:22971. [Crossref] [PubMed]
- Guler Z, Roovers JP. Role of Fibroblasts and Myofibroblasts on the Pathogenesis and Treatment of Pelvic Organ Prolapse. Biomolecules 2022;12:94. [Crossref] [PubMed]
- Wang XQ, He RJ, Xiao BB, et al. Therapeutic Effects of 17β-Estradiol on Pelvic Organ Prolapse by Inhibiting Mfn2 Expression: An In Vitro Study. Front Endocrinol (Lausanne) 2020;11:586242. [Crossref] [PubMed]
- Diedrich CM, Roovers JP, Smit TH, et al. Fully absorbable poly-4-hydroxybutyrate implants exhibit more favorable cell-matrix interactions than polypropylene. Mater Sci Eng C Mater Biol Appl 2021;120:111702. [Crossref] [PubMed]
- Li BS, Guo WJ, Hong L, et al. Role of mechanical strain-activated PI3K/Akt signaling pathway in pelvic organ prolapse. Mol Med Rep 2016;14:243-53. [Crossref] [PubMed]
- Zhao X, Ma C, Li R, et al. Hypoxia Induces Apoptosis through HIF-1α Signaling Pathway in Human Uterosacral Ligaments of Pelvic Organ Prolapse. Biomed Res Int 2017;2017:8316094. [Crossref] [PubMed]
- Alikhani M, Maclellan CM, Raptis M, et al. Advanced glycation end products induce apoptosis in fibroblasts through activation of ROS, MAP kinases, and the FOXO1 transcription factor. Am J Physiol Cell Physiol 2007;292:C850-6. [Crossref] [PubMed]
- Maehama T, Dixon JE. The tumor suppressor, PTEN/MMAC1, dephosphorylates the lipid second messenger, phosphatidylinositol 3,4,5-trisphosphate. J Biol Chem 1998;273:13375-8. [Crossref] [PubMed]
- Leslie NR, Downes CP. PTEN: The down side of PI 3-kinase signalling. Cell Signal 2002;14:285-95. [Crossref] [PubMed]
- Yamada KM, Araki M. Tumor suppressor PTEN: modulator of cell signaling, growth, migration and apoptosis. J Cell Sci 2001;114:2375-82. [Crossref] [PubMed]
- Qi Y, Liu J, Saadat S, et al. PTEN induces apoptosis and cavitation via HIF-2-dependent Bnip3 upregulation during epithelial lumen formation. Cell Death Differ 2015;22:875-84. [Crossref] [PubMed]
- Ma L, He H, Jiang K, et al. FAM46C inhibits cell proliferation and cell cycle progression and promotes apoptosis through PTEN/AKT signaling pathway and is associated with chemosensitivity in prostate cancer. Aging (Albany NY) 2020;12:6352-69. [Crossref] [PubMed]
- Zhao Y, Li A. miR-19b-3p relieves intervertebral disc degeneration through modulating PTEN/PI3K/AKT/MTOR signaling pathway. Aging (Albany NY) 2021;13:22459-73. [Crossref] [PubMed]
- Wen Z, Mai Z, Zhu X, et al. Mesenchymal stem cell-derived exosomes ameliorate cardiomyocyte apoptosis in hypoxic conditions through microRNA144 by targeting the PTEN/AKT pathway. Stem Cell Res Ther 2020;11:36. [Crossref] [PubMed]
- Wu L, Chen Y, Chen Y, et al. Effect of HIF-1α/miR-10b-5p/PTEN on Hypoxia-Induced Cardiomyocyte Apoptosis. J Am Heart Assoc 2019;8:e011948. [Crossref] [PubMed]
- Liang L, Zheng YW, Wang YL. miR-4429 Regulates the Proliferation, Migration, Invasion, and Epithelial-Mesenchymal Transition of Cervical Cancer by Targeting FOXM1. Cancer Manag Res 2020;12:5301-12. [Crossref] [PubMed]
- He H, Wu W, Sun Z, et al. MiR-4429 prevented gastric cancer progression through targeting METTL3 to inhibit m6A-caused stabilization of SEC62. Biochem Biophys Res Commun 2019;517:581-7. [Crossref] [PubMed]
- Moldogazieva NT, Mokhosoev IM. Oxidative Stress and Advanced Lipoxidation and Glycation End Products (ALEs and AGEs) in Aging and Age-Related Diseases. Oxid Med Cell Longev 2019;2019:3085756. [Crossref] [PubMed]
- Negre-Salvayre A, Coatrieux C, Ingueneau C, et al. Advanced lipid peroxidation end products in oxidative damage to proteins. Potential role in diseases and therapeutic prospects for the inhibitors. Br J Pharmacol 2008;153:6-20. [Crossref] [PubMed]
- Kim EJ, Chung N, Park SH, et al. Involvement of oxidative stress and mitochondrial apoptosis in the pathogenesis of pelvic organ prolapse. J Urol 2013;189:588-94. [Crossref] [PubMed]
- Fang G, Hong L, Liu C, et al. Oxidative status of cardinal ligament in pelvic organ prolapse. Exp Ther Med 2018;16:3293-302. [Crossref] [PubMed]
- Marcu RD, Mischianu DLD, Iorga L, et al. Oxidative Stress: A Possible Trigger for Pelvic Organ Prolapse. J Immunol Res 2020;2020:3791934. [Crossref] [PubMed]
- Li L, Sima Y, Wang Y, et al. The cytotoxicity of advanced glycation end products was attenuated by UCMSCs in human vaginal wall fibroblasts by inhibition of an inflammatory response and activation of PI3K/AKT/PTEN. Biosci Trends 2020;14:263-70. [Crossref] [PubMed]
- Zhu X, Luo C, Lin K, et al. Overexpression of DJ-1 enhances colorectal cancer cell proliferation through the cyclin-D1/MDM2-p53 signaling pathway. Biosci Trends 2020;14:83-95. [Crossref] [PubMed]
- Mazumder S, Plesca D, Almasan A. Caspase-3 activation is a critical determinant of genotoxic stress-induced apoptosis. Methods Mol Biol 2008;414:13-21. [Crossref] [PubMed]
- Zhu YP, Xie T, Guo T, et al. Evaluation of extracellular matrix protein expression and apoptosis in the uterosacral ligaments of patients with or without pelvic organ prolapse. Int Urogynecol J 2021;32:2273-81. [Crossref] [PubMed]
- Zhu Y, Li L, Xie T, et al. Mechanical stress influences the morphology and function of human uterosacral ligament fibroblasts and activates the p38 MAPK pathway. Int Urogynecol J 2022;33:2203-12. [Crossref] [PubMed]
- Zhang L, Dai F, Chen G, et al. Molecular mechanism of extracellular matrix disorder in pelvic organ prolapses. Mol Med Rep 2020;22:4611-8. [Crossref] [PubMed]
- Hong S, Hong L, Li B, et al. The role of GPX1 in the pathogenesis of female pelvic organ prolapse. PLoS One 2017;12:e0181896. [Crossref] [PubMed]
- Zeng W, Li Y, Li B, et al. Mechanical Stretching induces the apoptosis of parametrial ligament Fibroblasts via the Actin Cytoskeleton/Nr4a1 signalling pathway. Int J Med Sci 2020;17:1491-8. [Crossref] [PubMed]
- Wei L, Zhou Q, Tian H, et al. Integrin β3 promotes cardiomyocyte proliferation and attenuates hypoxia-induced apoptosis via regulating the PTEN/AKT/MTOR and ERK1/2 pathways. Int J Biol Sci 2020;16:644-54. [Crossref] [PubMed]
- Miao Z, Miao Z, Wang S, et al. Quercetin antagonizes imidacloprid-induced mitochondrial apoptosis through PTEN/PI3K/AKT in grass carp hepatocytes. Environ Pollut 2021;290:118036. [Crossref] [PubMed]
- Saliminejad K, Khorram Khorshid HR, Soleymani Fard S, et al. An overview of microRNAs: Biology, functions, therapeutics, and analysis methods. J Cell Physiol 2019;234:5451-65. [Crossref] [PubMed]
- Wang LP, Geng JN, Sun B, et al. MiR-92b-3p is Induced by Advanced Glycation End Products and Involved in the Pathogenesis of Diabetic Nephropathy. Evid Based Complement Alternat Med 2020;2020:6050874. [Crossref] [PubMed]
- Pan Y, Liang H, Liu H, et al. Platelet-secreted microRNA-223 promotes endothelial cell apoptosis induced by advanced glycation end products via targeting the insulin-like growth factor 1 receptor. J Immunol 2014;192:437-46. [Crossref] [PubMed]
- Li Y, Zhou Q, Pei C, et al. Hyperglycemia and Advanced Glycation End Products Regulate miR-126 Expression in Endothelial Progenitor Cells. J Vasc Res 2016;53:94-104. [Crossref] [PubMed]
- Piperi C, Goumenos A, Adamopoulos C, et al. AGE/RAGE signalling regulation by miRNAs: associations with diabetic complications and therapeutic potential. Int J Biochem Cell Biol 2015;60:197-201. [Crossref] [PubMed]
- Pan H, Hong Y, Yu B, et al. miR-4429 Inhibits Tumor Progression and Epithelial-Mesenchymal Transition Via Targeting CDK6 in Clear Cell Renal Cell Carcinoma. Cancer Biother Radiopharm 2019;34:334-41. [Crossref] [PubMed]
- Worby CA, Dixon JE. PTEN. Annu Rev Biochem 2014;83:641-69. [Crossref] [PubMed]
- Carnero A, Blanco-Aparicio C, Renner O, et al. The PTEN/PI3K/AKT signalling pathway in cancer, therapeutic implications. Curr Cancer Drug Targets 2008;8:187-98. [Crossref] [PubMed]
- Li Y, Xia J, Jiang N, et al. Corin protects H2O2-induced apoptosis through PI3K/AKT and nf-κB pathway in cardiomyocytes. Biomed Pharmacother 2018;97:594-9. [Crossref] [PubMed]
- Song M, Yang Q, Zhang F, et al. Hyodeoxycholic acid (HDCA) suppresses intestinal epithelial cell proliferation through FXR-PI3K/AKT pathway, accompanied by alteration of bile acids metabolism profiles induced by gut bacteria. FASEB J 2020;34:7103-17. [Crossref] [PubMed]
- Liu R, Chen Y, Liu G, et al. PI3K/AKT pathway as a key link modulates the multidrug resistance of cancers. Cell Death Dis 2020;11:797. [Crossref] [PubMed]
- Zhang S, Lu Y, Li H, et al. A steroidal saponin form Paris vietnamensis (Takht.) reverses temozolomide resistance in glioblastoma cells via inducing apoptosis through ROS/PI3K/Akt pathway. Biosci Trends 2020;14:123-33. [Crossref] [PubMed]
- Liu HY, Zhang YY, Zhu BL, et al. miR-21 regulates the proliferation and apoptosis of ovarian cancer cells through PTEN/PI3K/AKT. Eur Rev Med Pharmacol Sci 2019;23:4149-55. [PubMed]
- Haddadi N, Lin Y, Travis G, et al. PTEN/PTENP‘: 'Regulating the regulator of RTK-dependent PI3K/Akt signall’ng', new targets for cancer therapy. Mol Cancer 2018;17:37. [Crossref] [PubMed]