Exogenous H2S promotes ion channel reconstruction to regulate colonic motility in rats with dinitrobenzene sulfonic acid-induced colitis
Introduction
Hydrogen sulfide (H2S) is a type of noxious gas. Exposure to high concentrations of H2S results in identified respiratory, cardiovascular, metabolic, and neurological diseases (1). H2S is produced in many tissues of the human body, such as the gastrointestinal tract, which is a major organ for H2S generation (2,3). Normal plasma concentrations of H2S range from 30–100 µmol/L (4). At present, there is extensive evidence that H2S is a crucial molecule for humans, and plays a crucial role in physiology and pathophysiology in humans, especially in the gastrointestinal tract (1). In addition to carbon monoxide (CO) and nitric oxide (NO), H2S is also regarded as the 3rd gasotransmitter (5).
H2S is implicated in gut motility, inflammation, ulcer healing, oxidative stress, and many other vital biological functions (5,6). Recently, considerable research has been conducted to identify the molecular mechanisms involved in the anti-inflammatory effect of H2S. There are diametrically opposed views on the role of H2S in inflammatory diseases, but it has been recognized for centuries that H2S can reduce pain and inflammation (7). Many early studies reported that elevated H2S levels generated within the colon lumen by bacteria result in colitis or even colon cancer (8,9). However, there is increasing evidence that H2S plays an important role in preventing tissue damage, reducing inflammation, and promoting repair (4,7). The balance of evidence reveals that at a physiological concentration, H2S exerts an anti-inflammatory effect.
In the context of intestinal inflammation, H2S could be used therapeutically for colitis, functioning to reduce dysbiosis and helping mucus layer reconstitution (10,11). There is evidence showed that intestinal inflammation results in gut microbiota dysbiosis and barrier function damage. H2S acts as an important regulator of mucosal homeostasis, and mediates microbiota biofilm and the mucus barrier repair functions, further promoting the resolution of colonic inflammation (12). The study revealed that events that fragmented microbiota biofilms and reduced production of mucus granule were occurred during the development of colitis (12). Thus, we can take advantage of endogenous production of H2S to enhance the establishment of microbiota biofilms and the production of colonic mucus. There are evidences suggesting that therapeutic delivery of exogenous H2S into the colon have good curative effects of reducing inflammation, restoring the microbiota biofilm, and increasing the production of mucus granules. Besides, study findings showed that H2S not only promotes biofilm formation, but also reduces the growth of planktonic bacteria in ex vivo human microbiota (12). Given that H2S has a number of beneficial properties that can be useful in the treatment of all kinds of diseases, including end organ ischemia (13), diabetes (14), gastrointestinal disorders (15) and so on. Thus, H2S can be a target of pharmacotherapy and the pharmacological effects are focus on exploiting the potent anti-inflammatory and cytoprotective actions (15). Many companies have developed H2S-based drugs to reduce the gastrointestinal ulceration that is normally caused by non-steroidal anti-inflammatory drugs (NSAIDs). The lead drug (ATB-346), a naproxen derivative, which is used for osteoarthritis treatment, is going through the development stage and has completed Phase I clinical trials in healthy volunteers (15,16). Moreover, the lead drug, GIC-1001 is developed by GIcare Pharma company to reduce visceral pain (17). GIC-1001 is a salt that is the counter-ions being thiobenzamide and trimebutine, and this drug has been used to treat various of gastrointestinal conditions. The preliminary test results showed GIC-1001 is safe in a Phase I clinical trial (ClinicalTrials.gov identifier: NCT01738425), and this drug is now in Phase II clinical trials as a pre-colonoscopy analgesic (ClinicalTrials.gov identifier: NCT01926444 and NCT02276768) (15).
Inflammatory bowel disease (IBD) is a chronic gastrointestinal inflammatory disease, which includes ulcerative colitis and Crohn’s disease (18). Damaged colon motility is a major issue of IBD (19). Colonic motor neuromuscular dysfunction, such as rapid transit and increased propulsive activity, may be induced by pathological disorders, including colonic diverticular disease, irritable bowel syndrome, and even low-grade inflammation (20). Experimental results with H2S donor sodium hydrosulfide (NaHS) have generally shown that in addition to inducing the concentration-dependent relaxation of smooth muscle contractility, H2S has a suppressive effect on gastrointestinal motility (21,22). In the colon smooth muscle cells of rats, H2S has been shown to have an inhibitory effect on both large conductance Ca2+-activated K+ (BKCa) channels and L-type calcium channels (23). However, the role of H2S in calcium channels is not yet clear.
Although several studies have detailedly showed the complex relationship between inflammatory bowel diseases and gastrointestinal motility disorders, and the regulatory function of H2S on calcium channel in colitis, it is still unclear that in the context of intestinal inflammation, the effect of exogenous H2S on intestinal motility, which requires further exploration (3,24). Here, we investigated the mechanism of H2S on intestinal motility. In this study, a colitis model induced by dinitrobenzene sulfonic acid (DNBS) was used to further examine the function of H2S in intestinal motility and the mechanism underlying its participation in calcium channels. We present the following article on accordance with the ARRIVE reporting checklist (available at https://atm.amegroups.com/article/view/10.21037/atm-22-2126/rc).
Methods
Reagents and antibodies
NaHS (a donor of H2S) and DNBS were purchased from Sigma-Aldrich. Antibodies against KCND3, KCNMA1, and KCNJ8 were provided by Boster Biological Technology. The glyceraldehyde-3-phosphate dehydrogenase (GAPDH) antibody was obtained from Abcam. Enzyme-linked immunoassay (ELISA) kits for rat tumor necrosis factor alpha (TNF-α), interleukin (IL)-1β, and IL-6 were purchased from Nanjing Jiancheng Bioengineering Institute (Nanjing, China). Quantitative-reverse transcription polymerase chain reaction (qRT-PCR) primers were obtained from Invitrogen (Shanghai, China). TRIzol, complementary deoxyribonucleic acid (cDNA) synthesis kits, intracellular calcium detection kits, and Bestar™ qPCR-RT kits were purchased from Invitrogen.
Animals and ethical statements
A total of 30 adult male Wistar rats (weighing 200–250 g) from the Guilin Medical University Animal Center were accommodated in plastic cages and kept under controlled humidity of 60–70%, at a temperature of 20 ℃, on a light-dark light cycle of 12–12 hours, and fed with standard laboratory water and chow ad libitum. After adaption for 7 days, the rats were randomly separated into the following 3 groups (10 per group): (I) the control group; (II) the model group; and (III) the NaHS group. All the experimental procedures were approved by the Institutional Animal Ethics Committee of Guilin Medical University (No. GLMC202203270), in compliance with Guide for the Care and Use of Laboratory Animals, 8th edition. A protocol was prepared before the study without registration.
Induction and assessment of colitis
Colitis was induced in animals with DNBS in the model group and the NaHS group (25). DNBS was administered to the model group and NaHS group once in accordance with the following protocol. An 8-cm long thin plastic tube was slowly inserted 7 cm into the anus of the rat, and the drug (30% DNBS) was slowly pushed with the syringe connected to the plastic tube. After the plastic tube was slowly removed, the rat’s head was kept downward for 60 seconds. An enema with an equal volume of normal saline (NS) was used for the control group, and the other steps were the same as those detailed above. After 1 day of DNBS treatment, the NaHS group was given intraperitoneal injections daily of 1 mg/kg of NaHS, dissolved in 100 µL of sterile NS. In parallel, the other rats received intraperitoneal injections of NS (100 µL) daily. All the rats were sacrificed on the 8th day.
The severity and inflammation of colitis were assessed by examining weight loss, diarrhea and hematochezia. The disease activity index (DAI) was determined using the following equation: DAI = (score of weight loss rate + score of fecal property + score of hematochezia status)/3.
The body weight of each rat was determined using an electronic balance (n=8). Fecal property was graded as loose, semi-loose, or normal. Loose stools refer to watery stools that adhere to the anus; semi-loose stools refer to semi-formed stools or paste-shaped loose stools that do not adhere to the anus; and normal stools refer to well-formed stools. Hematochezia status was graded using the Modified EZ Detect Fecal Occult Blood Test Kits (26), as normal feces, feces with light occult blood (++), or feces with heavy occult blood (+++).
Gut motility determination
The protocol for gastrointestinal transit detection has been previously described (27,28). After the animals were fasted overnight, activated carbon ink (10 mL/kg) was administered orally. The 1st black feces defecation of the rats was recorded. Next, 0.5 h later, the rats were sacrificed by cervical dislocation. The colon was instantly and carefully excised without stretching, and the total length of the colon and the distance traveled by the ink was evaluated. The data are presented as the percentage (%) of the distance that the ink traveled along the full length of colon.
HE staining
After rats were sacrificed, the distal colon was harvested and washed, and then fixed by 4% paraformaldehyde overnight at 4 ℃. The tissues underwent a cleaning and dehydration step before being paraffin-embedded. After being sliced, the colonic tissues were exposed to standard HE staining to assess the integrity of the intestinal mucosal barrier and the inflammatory cells influx in the mucosal layers. A total of 3 regions, observed blindly by 3 independent researchers, were randomly selected from each sample.
ELISA
The whole blood from each rat was collected and left undisturbed for 15–30 min at room temperature to clot. The serum was separated by centrifuging the whole blood in a refrigerated centrifuge for 10 minutes at 1,000–2,000 ×g to remove the clot. Next, we determined TNF-α, IL-1β, and IL-6 levels in the serum of the rats using the appropriate ELISA kits in accordance with the instructions. The concentrations of the inflammatory factors were evaluated using a microplate reader according to the optical density values at 450 nm. The experiment was repeated 3 times.
Preparing dispersed smooth muscle cells
The rats were vertically abdominally incised after being euthanized with carbon dioxide inhalation. Next, the proximal colons were transferred in buffer containing 25 mM of N-2-hydroxy-ethylpiperazine-N’ 2-ethanesulfonic acid (HEPES), 14 mM of glucose, 2.0 mM of Calcium chloride (CaCl2), 2.6 mM of Potassium dihydrogen phosphate (KH2PO4), 0.6 mM of Magnesium Chloride (MgCl2), 4 mM of Potassium Chloride (KCl), 120 mM of Sodium Chloride (NaCl), and 2.1% basic Eagle Medium (essential amino mixture, pH 7.4). The mucosal layer was scraped gently from the muscle layer, and the successive enzymatic digestion of muscle strips, filtration, and centrifugation were performed to separate the smooth muscle cells. The muscle layer strips were treated in a digestive solution, containing trypsin inhibitor (0.1%), collagenase II (0.15%), and bovine serum albumin (0.25%) in a calcium-free physiological saline solution (PSS) at 37 ℃ for 20–30 min. During digestion, 100% oxygen was applied to bubble the tissues mildly. Next, collagenase-free medium was used to rinse the partially digested tissues twice. The smooth muscle cells were allowed to disperse in the collagenase-free medium spontaneously. Subsequently, the dispersed smooth muscle cells were filtered with 500 µm of Nitex and suspended in HEPES medium (29,30).
Total RNA extraction and RT-PCR analysis
The total RNA extraction was performed using the following protocol. TRIzol (1.0 mL) was added to the smooth muscle cells for 5 min at 4℃, after which 0.2 mL of chloroform was added. After being oscillated for 15 s, the mixture was incubated for 3 min at room temperature before being centrifuged at 12,000 rpm for 15 min at 4 ℃. The upper aqueous phase was removed to a new tube before the addition of an equal volume of isopropanol. The mixture was treated and centrifuged at 12,000 rpm for 15 min at 4 ℃. The supernatant was removed and 1 mL of cold diethylpyrocarbonate (DEPC)-treated ethanol (75%) was used to wash the pellet. They were again centrifuged at 8,000 rpm at 4 ℃ for 15 min. After collection, they were air-dried for 15 min at room temperature. After being dissolved into 30 µL of 0.1% DEPC-treated water, the RNA was measured with an ultra-micro ultraviolet analyzer, and then stored at –80℃ awaiting use.
For the cDNA synthesis, 2 µg of RNA in a final volume of 10 µL reverse transcription reaction system were used. The reverse transcription reaction was performed in accordance with the manufacturer’s instructions using a BestarTM qPCR-RT kit (DBI Bioscience, China). qRT-qPCR was carried out on a Stratagene Mx3000p Real-Time PCR machine (Agilent Technologies, CA, USA), and was done in a final volume of 20 µL, containing 10 µL of Bestar® SybrGreen qPCR master mix, 0.5 µL of 10-µM forward primer, 0.5 µL of 10-µM reverse primer, 1.0 µL of cDNA, and 8.0 µL of DNase/RNase-free water. The primer sequences and expected product length of KCND3, KCNMA1, and KCNJ8 in the rats are shown in Table 1 (31). The conditions were 2 min of initial denaturation at 95 ℃, 20 s at 94 ℃, 20 s at 58 ℃, and 20 s at 72 ℃ (40 cycles) with a final extension step of 5 min at 72 ℃. The products of PCR were separated by electrophoresis in a 1.2% agarose gel at 100 V in the presence of ethidium bromide. Ultraviolet fluorescence and a Bio-Rad Gel Doc EZ Imager were used to visualize and record the bands. The relative expression evaluated from cycle thresholds by the individual standard amplification curves of each transcript was standardized to GAPDH mean expression.
Table 1
Gene | Sequence (5'-3') | Product length (bp) |
---|---|---|
GAPDH | F: CCTCGTCTCATAGACAAGATGGT | 169 |
R: GGGTAGAGTCATACTGGAACATG | ||
KCND3 | F: GCAAGACCACGTCACTCATC | 116 |
R: GGTGGAGGTTCGTACAGACA | ||
KCNJ8 | F: AGAACATCCGAGAGCAAGGT | 135 |
R: GCCACCACATGATAGCGAAG | ||
KCNMA1 | F: TCCATCACAACCGGAGTCAA | 223 |
R: CTGTCACCAGGGTCCGTATT |
KCND3, potassium voltage gated channel subfamily D member 3; KCNMA1, maxi potassium channel alpha; KCNJ8, potassium inwardly rectifying channel J8.
Western blot analysis
The smooth muscle cells were solubilized in Triton X-100-based lysis buffer plus protease and phosphatase inhibitors. The lysates were centrifuged at 20,000 rpm for 10 min at 4 ℃ and a Bicinchoninic Acid (BCA) Protein Assay kit (Thermo, #23227) was used to measure supernatant protein concentrations. The proteins were separated with Sodium dodecyl sulfate-polyacrylamide gel electrophoresis (SDS-PAGE) and removed to Polyvinylidene fluoride (PVDF, Millipore, IPVH00010) membranes. They were treated with antibodies to KCND3 (Boster, PB0226, 1:500), KCNMA1 (Boster, PB0227, 1:500), and KCNJ8 (Boster, A04950-1, 1:500) for 12 h at 4 ℃ before incubation with a secondary antibody conjugated with horseradish peroxidase for 1 h. Enhanced chemiluminescence was used to visualize the protein bands.
Statistical analyses
The statistical analyses were conducted with SPSS 17.0 (SPSS Inc., Chicago, IL, USA). The results are presented as the mean ± standard deviation. The differences between groups were compared by a 1-way analysis of variance (ANOVA) followed by a Tukey’s post-hoc test. A P value <0.05 was considered statistically significant.
Results
Colonic motility and ion channels were altered by DNBS-induced colitis in rats
To explore the anti-inflammatory activity of H2S, we adopted a DNBS-induced colitis model. DNBS (30%) was directly injected into the rat colon. Compared to the control group, an insignificant decline in body weight was observed in the model group (Figure 1A). The number of stools in the model group was significantly reduced after the 2nd day of the observation period (Figure 1B). The histological assessment of the colonic specimens revealed crypt deformation, goblet cell loss, inflammatory cell infiltration, and mucosal tissue damage (Figure 1C). The DAI of the rats was also calculated. Its level in the model group was obviously higher than that in the control group (Figure 1D).
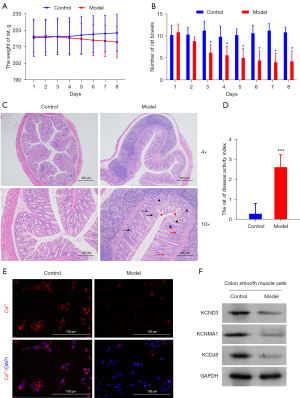
To further investigate alterations in the calcium-ion channel of rat colitis induced by DNBS, the distribution of calcium ion was imaged with a fluorescence-inverted microscope. Cellular calcium exists in some special organelles, such as mitochondria, sarcoplasmic reticulum, nucleus, and plasma membrane. We observed a more concentrated distribution of calcium ions in the model group than the control group (Figure 1E). To confirm that the anti-inflammatory effect of H2S is involved in the calcium ion-related channel, protein expression of KCND3, KCNMA1, and KCNJ8 genes in the smooth cells were analyzed using Western blot techniques. The protein expression levels of each target gene expressed in the model group were higher than those of the control group (Figure 1F). Our results confirmed that DNBS-induced colitis altered the colonic motility and ion channels in the colonic epithelial tissues of the rats.
H2S prevents DNBS-induced colonic inflammation in rats
To investigate the anti-inflammatory activity of H2S, NaHS was applied to the rats with DNBS-induced colitis. Compared to the control and model groups, partial weight recovery was observed in the NaHS group, but there was no significant difference in comparison to the model group (Figure 2A). The DAI of the rats was obviously reduced in the NaHS group (Figure 2B), and the pathological alterations were inhibited in the NaHS group (Figure 2C). These results suggest that H2S ameliorates DNBS-induced colitis in rats.
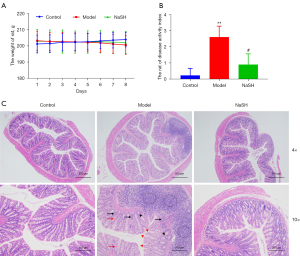
H2S improved rat intestinal motility and reduced serum inflammatory factors
After DNBS-induced colitis, the bowels were significantly reduced in the model group, but no changes were observed in the NaHS group (Figure 3A). We also observed a similar shortening of the colon in both the model and NaHS groups, and the length difference was not statistically significant. The carbon powder traveled further in the rat colons of the rats in the NaHS group than in the rat colons of the rats in the model group; however, the length of the colons in the NaHS group was still shorter than the length of the colons in the control group (Figure 3B,3C). Pivotal pro-inflammatory cytokines, including IL-1β, TNF-α, and IL-6, are involved in colitis. In the model and NaHS groups, the expressions of IL-1β, IL-6, and TNF-α were significantly higher than those in the control group, while those in the NaHS group were significantly lower in comparison to the model group (Figure 3D-3F). These results suggest that H2S ameliorates DNBS-induced colitis in rats and show that H2S protects intestinal motility from DNBS damage.
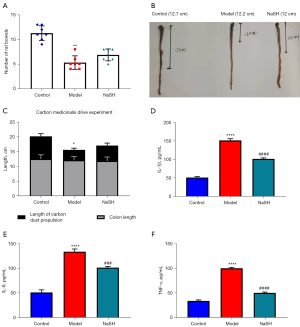
H2S affected the distribution of Ca2+ in smooth muscle cells
Colitis contributes to the electrical and structural remodeling of smooth muscle cells (32). A previous study has reported that the calcium-ion channel is involved in the anti-inflammatory effect of H2S on colitis (1). After NaHS was administered to the rats with DNBS-induced colitis, cellular calcium was observed in some special organelles, such as the mitochondria, sarcoplasmic reticulum, nucleus, and plasma membrane. We observed a normal distribution in the NaHS group compared to that of the control and model groups (Figure 4). The images indicated that anti-inflammatory effect of H2S may involves in calcium ion related channel by affecting cellular calcium.
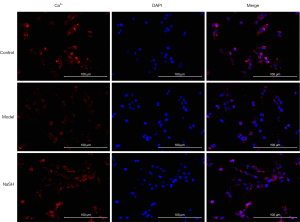
H2S unregulated the expression of KCND3, KCNMA1 and KCNJ8 genes in smooth muscle cells
The protein expression of KCND3, KCNMA1, and KCNJ8 genes are important for the calcium ion (Ca2+)-activated K+ channel or the inwardly rectifying K+ channels (33). To confirm that the anti-inflammatory effect of H2S involves in calcium-ion related channel by affecting cellular calcium, the mRNA and protein expression levels of the 3 genes in the smooth cells were also analyzed using both qPCR and Western blot techniques. The mRNA and protein expression levels in the NaHS group were lower than those in the model group (Figure 5). The results suggest that H2S regulates colonic motility by promoting ion channel remodeling.
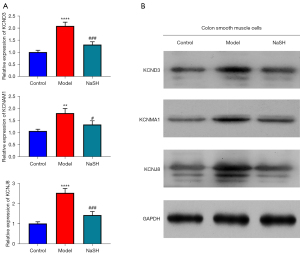
Discussion
DNBS-induced colitis can be applied to study the mechanisms involved in mucosal injury and intestinal inflammation, and the effects of potential therapies on IBD (34,35). IBD is characterized by chronic relapsing inflammation in the gastrointestinal tract, which induces symptoms of diarrhea, weight loss, and injured mucosa. In this study, we also detected the typical symptoms of IBD in a DNBS-induced model, and further found the DNBS damaged rat intestinal motility and affected the abnormal distribution of Ca2+ in smooth muscle cells, while H2S protected the rat intestine from DNBS by reconstructing the ion channels.
In healthy individuals, semi-solid contents from the terminal ileum enter the colon, and are compacted and propelled in the colon. When the colonic contents enter the rectum, rectal ampulla distension, and the anal sphincter reflex send signals to the central nervous system about whether to evacuate. However, in IBD individuals, the function may be disordered by inflammatory conditions in the gut (20). We observed that DNBS induced small intestine shortening and reduced intestinal transit in rats, while the administration of H2S donor NaHS reversed the alterations. The colonic mucosa possesses an effective H2S-detoxifying mechanism in the rat colon to oxidize H2S (>300 µmol per day) (36). If severe colitis damages the barrier, abundant H2S may enter the muscle layers to suppress motility (37). However, these findings conflict with our findings, but this may be due to the animal (rat) and the dosage (1 mg/kg) of NaHS we used in our experiments. For example, Grasa et al. (38) administered 5 mg/kg of NaHS for Tlr2−/− and Tlr4−/− mice. The limits between physiological, pharmacological, and even toxic concentrations are still unknown (21). Our results showed that a lower dosage of NaHS improved intestinal motility in rats with DNBS-induced colitis. In addition, low dosage of NaHS may be the key reason for reducing inflammation. A previous study at the cellular level revealed that low concentration of H2S enhances cell viability, mitochondrial sulfide metabolism and oxidative stress, whereas high concentration of H2S promotes inflammatory response and oxidative stress, further influencing the mitochondrial sulfide metabolism ability, which may be detrimental to gut health (39). These provide direct evidence for using lower doses of hydrogen sulfide for the treatment of colitis. Moreover, upon the treatment with H2S, the reducing level of inflammatory factors in blood could serve as a prognostic indicator, but the threshold requires further validation.
Intestinal transit is generated by intestinal smooth muscles (33,40). Smooth muscles constitute 2 layers of circular or longitudinal muscle bundles throughout most of the gastrointestinal tract. Smooth muscle cells coordinate contractions by forming mechanical and electrical junctions between cells. Ca2+ entry through ion channels causes excitation-contraction coupling in the plasma membrane, which results in an elevation of intracellular Ca2+ (33). The concentration of intracellular free Ca2+ widely varies based on its location. In the resting state, the extracellular Ca2+ is −10−3 M, 104 times higher than the cytoplasmic Ca2+ of −10−7 M. In the cells, the levels of Ca2+ in the mitochondrial matrix and in the nuclear matrix are comparable to those in the cytoplasm. Conversely, other intracellular organelles that are considered Ca2+ stores can accumulate Ca2+ and keep a higher Ca2+ of 1–5×10−4 M than that kept in the cytoplasm. The endoplasmic reticulum and the sarcoplasmic reticulum in the muscle cells are the main organelles for the internal Ca2+ store. The healthy physiological distribution of Ca2+ is vital if smooth muscle cells are to exchange signals (41). However, our results showed that the distribution of Ca2+ in the smooth muscle cells of rats with DNBS-induced colitis was abnormal, but H2S donor NaHS reversed the destruction of DNBS on rat colon and restored the normal physiological distribution of calcium. H2S regulates various calcium-ion channels and transporters and is thus involved in a strong physiological and pathological process (42). Further, H2S mediates various cellular biological processes associated with the regulation of intracellular calcium concentration (42). Our results support those previous reports above. Of course, given that H2S function as a novel signaling molecule has been found to be involved in all kinds of cellular functions, including apoptosis, inflammation, and perfusion. However, we still have some questions for the deeper regulatory mechanism of H2S on colonic motility. A study has proved that H2S augments of colonic motility by regulating cGMP/PKG signaling pathway (43). Besides, there is also evidence revealed that H2S enhances gastric fundus smooth muscle tone by regulating voltage-dependent potassium and calcium channels (44). Therefore, we can speculate that H2S influences calcium channels, further regulating colonic motility by affecting cGMP/PKG signaling pathway in the context of intestinal inflammation. However, this hypothesis needs to be confirmed in the subsequent study. The interaction between H2S and transporters and calcium-ion channels have been exhibited in different cells (42). The DNBS intervention inhibited the KCND3, KCNJ8, and KCNMA1 protein and mRNA levels in rat smooth muscles. The proteins expressed by KCND3, KCNJ8, and KCNMA1 are components of the A-type K+ channel, the Adenosine Triphosphate (ATP)-sensitive K+ channel and Ca2+-dependent K+ channel, respectively (33). This suggests that DNBS reduces these channels by activating the expression of KCND3, KCNMA1, and KCNJ8, which is consistent with previous study about alterations in gene and protein expressions of smooth muscle isoform of ATP-sensitive K+ channels and L-type Ca2+ channels due to gastrointestinal inflammation (32). Conversely, H2S donor NaHS improved their expression in smooth muscle cells, which suggests that H2S can reconstruct the A-type K+ channel, ATP-sensitive K+ channel and Ca2+-based K+ channel in our experiments. This provides novel insights into the role of H2S in anti-inflammatory actions.
Conclusions
Taken together, H2S donor NaHS improved the intestinal mucosa of colitis rats by alleviating tissue edema and congestion and repairing the gland structure of the intestinal mucosa. NaHS also reduced the levels of inflammatory factors in rat blood. Additionally, the NaHS intervention partially restored rat intestinal motility by downregulating the protein and mRNA expressions of KCND3, KCNMA1, and KCNJ8 to reconstruct ion channels.
Acknowledgments
Funding: This work was supported by the National Natural Science Foundation of China (No. 81960106).
Footnote
Reporting Checklist: The authors have completed the ARRIVE reporting checklist. Available at https://atm.amegroups.com/article/view/10.21037/atm-22-2126/rc
Data Sharing Statement: Available at https://atm.amegroups.com/article/view/10.21037/atm-22-2126/dss
Conflicts of Interest: All authors have completed the ICMJE uniform disclosure form (available at https://atm.amegroups.com/article/view/10.21037/atm-22-2126/coif). The authors have no conflicts of interest to declare.
Ethical Statement: The authors are accountable for all aspects of the work, including ensuring that any questions related to the accuracy or integrity of any part of the work have been appropriately investigated and resolved. This animal experiment was approved by the Institutional Animal Ethics Committee of Guilin Medical University (No. GLMC202203270), in compliance with Guide for the Care and Use of Laboratory Animals, 8th edition.
Open Access Statement: This is an Open Access article distributed in accordance with the Creative Commons Attribution-NonCommercial-NoDerivs 4.0 International License (CC BY-NC-ND 4.0), which permits the non-commercial replication and distribution of the article with the strict proviso that no changes or edits are made and the original work is properly cited (including links to both the formal publication through the relevant DOI and the license). See: https://creativecommons.org/licenses/by-nc-nd/4.0/.
References
- Sudha SB, Lin HC. Hydrogen Sulfide in Physiology and Diseases of the Digestive Tract. Microorganisms 2015;3:866-89. [Crossref] [PubMed]
- Blachier F, Andriamihaja M, Larraufie P, et al. Production of hydrogen sulfide by the intestinal microbiota and epithelial cells and consequences for the colonic and rectal mucosa. Am J Physiol Gastrointest Liver Physiol 2021;320:G125-35. [Crossref] [PubMed]
- Xiao A, Liu C, Li J. The Role of H2S in the Gastrointestinal Tract and Microbiota. Adv Exp Med Biol 2021;1315:67-98. [Crossref] [PubMed]
- Wallace JL, Vong L, McKnight W, et al. Endogenous and exogenous hydrogen sulfide promotes resolution of colitis in rats. Gastroenterology 2009;137:569-78, 578.e1.
- Magierowski M, Magierowska K, Kwiecien S, et al. Gaseous mediators nitric oxide and hydrogen sulfide in the mechanism of gastrointestinal integrity, protection and ulcer healing. Molecules 2015;20:9099-123. [Crossref] [PubMed]
- Flannigan KL, Agbor TA, Motta JP, et al. Proresolution effects of hydrogen sulfide during colitis are mediated through hypoxia-inducible factor-1α. FASEB J 2015;29:1591-602. [Crossref] [PubMed]
- Gemici B, Wallace JL. Anti-inflammatory and cytoprotective properties of hydrogen sulfide. Methods Enzymol 2015;555:169-93. [Crossref] [PubMed]
- Roediger WE, Duncan A, Kapaniris O, et al. Sulphide impairment of substrate oxidation in rat colonocytes: a biochemical basis for ulcerative colitis? Clin Sci (Lond) 1993;85:623-7. [Crossref] [PubMed]
- Attene-Ramos MS, Wagner ED, Plewa MJ, et al. Evidence that hydrogen sulfide is a genotoxic agent. Mol Cancer Res 2006;4:9-14. [Crossref] [PubMed]
- Kalantar-Zadeh K, Berean KJ, Burgell RE, et al. Intestinal gases: influence on gut disorders and the role of dietary manipulations. Nat Rev Gastroenterol Hepatol 2019;16:733-47. [Crossref] [PubMed]
- Tomasova L, Konopelski P, Ufnal M. Gut Bacteria and Hydrogen Sulfide: The New Old Players in Circulatory System Homeostasis. Molecules 2016;21:1558. [Crossref] [PubMed]
- Motta JP, Flannigan KL, Agbor TA, et al. Hydrogen sulfide protects from colitis and restores intestinal microbiota biofilm and mucus production. Inflamm Bowel Dis 2015;21:1006-17. [Crossref] [PubMed]
- Jensen AR, Drucker NA, Khaneki S, et al. Hydrogen Sulfide: A Potential Novel Therapy for the Treatment of Ischemia. Shock 2017;48:511-24. [Crossref] [PubMed]
- Wu L, Yang W, Jia X, et al. Pancreatic islet overproduction of H2S and suppressed insulin release in Zucker diabetic rats. Lab Invest 2009;89:59-67. [Crossref] [PubMed]
- Wallace JL, Wang R. Hydrogen sulfide-based therapeutics: exploiting a unique but ubiquitous gasotransmitter. Nat Rev Drug Discov 2015;14:329-45. [Crossref] [PubMed]
- Wallace JL, Caliendo G, Santagada V, et al. Markedly reduced toxicity of a hydrogen sulphide-releasing derivative of naproxen (ATB-346). Br J Pharmacol 2010;159:1236-46. [Crossref] [PubMed]
- Ekundi-Valentim E, Santos KT, Camargo EA, et al. Differing effects of exogenous and endogenous hydrogen sulphide in carrageenan-induced knee joint synovitis in the rat. Br J Pharmacol 2010;159:1463-74. [Crossref] [PubMed]
- Pan J, Fan Z, Wang Z, et al. CD36 mediates palmitate acid-induced metastasis of gastric cancer via AKT/GSK-3β/β-catenin pathway. J Exp Clin Cancer Res 2019;38:52. [Crossref] [PubMed]
- Dinakaran V, Mandape SN, Shuba K, et al. Identification of Specific Oral and Gut Pathogens in Full Thickness Colon of Colitis Patients: Implications for Colon Motility. Front Microbiol 2018;9:3220. [Crossref] [PubMed]
- Bassotti G, Antonelli E, Villanacci V, et al. Colonic motility in ulcerative colitis. United European Gastroenterol J 2014;2:457-62. [Crossref] [PubMed]
- Jimenez M, Gil V, Martinez-Cutillas M, et al. Hydrogen sulphide as a signalling molecule regulating physiopathological processes in gastrointestinal motility. Br J Pharmacol 2017;174:2805-17. [Crossref] [PubMed]
- Martinez-Cutillas M, Gil V, Mañé N, et al. Potential role of the gaseous mediator hydrogen sulphide (H2S) in inhibition of human colonic contractility. Pharmacol Res 2015;93:52-63. [Crossref] [PubMed]
- Quan X, Luo H, Liu Y, et al. Hydrogen sulfide regulates the colonic motility by inhibiting both L-type calcium channels and BKCa channels in smooth muscle cells of rat colon. PLoS One 2015;10:e0121331. [Crossref] [PubMed]
- Medani M, Collins D, Docherty NG, et al. Emerging role of hydrogen sulfide in colonic physiology and pathophysiology. Inflamm Bowel Dis 2011;17:1620-5. [Crossref] [PubMed]
- Abu-Gharbieh E, Bayoumi FA, Ahmed NG. Alleviation of antioxidant defense system by ozonized olive oil in DNBS-induced colitis in rats. Mediators Inflamm 2014;2014:967205. [Crossref] [PubMed]
- Xie J, Liu Y, Chen B, et al. Ganoderma lucidum polysaccharide improves rat DSS-induced colitis by altering cecal microbiota and gene expression of colonic epithelial cells. Food Nutr Res 2019;63: [Crossref] [PubMed]
- Xie W, Xing D, Zhao Y, et al. A new tactic to treat postprandial hyperlipidemia in diabetic rats with gastroparesis by improving gastrointestinal transit. Eur J Pharmacol 2005;510:113-20. [Crossref] [PubMed]
- Yu ZC, Cen YX, Wu BH, et al. Berberine prevents stress-induced gut inflammation and visceral hypersensitivity and reduces intestinal motility in rats. World J Gastroenterol 2019;25:3956-71. [Crossref] [PubMed]
- Teng B, Murthy KS, Kuemmerle JF, et al. Expression of endothelial nitric oxide synthase in human and rabbit gastrointestinal smooth muscle cells. Am J Physiol 1998;275:G342-51. [PubMed]
- Murthy KS, Zhou H, Grider JR, et al. Inhibition of sustained smooth muscle contraction by PKA and PKG preferentially mediated by phosphorylation of RhoA. Am J Physiol Gastrointest Liver Physiol 2003;284:G1006-16. [Crossref] [PubMed]
- May AT, Crowe MS, Blakeney BA, et al. Identification of expression and function of the glucagon-like peptide-1 receptor in colonic smooth muscle. Peptides 2019;112:48-55. [Crossref] [PubMed]
- Akbarali HI, G, Hawkins E, Ross GR, et al. Ion channel remodeling in gastrointestinal inflammation. Neurogastroenterol Motil 2010;22:1045-55. [Crossref] [PubMed]
- Koh SD, Ward SM, Sanders KM. Ionic conductances regulating the excitability of colonic smooth muscles. Neurogastroenterol Motil 2012;24:705-18. [Crossref] [PubMed]
- Morampudi V, Bhinder G, Wu X, et al. DNBS/TNBS colitis models: providing insights into inflammatory bowel disease and effects of dietary fat. J Vis Exp 2014;e51297. [Crossref] [PubMed]
- Chidrawar V, Alsuwayt B. Defining the role of CFTR channel blocker and ClC-2 activator in DNBS induced gastrointestinal inflammation. Saudi Pharm J 2021;29:291-304. [Crossref] [PubMed]
- Suarez F, Furne J, Springfield J, et al. Production and elimination of sulfur-containing gases in the rat colon. Am J Physiol 1998;274:G727-33. [PubMed]
- Guo FF, Yu TC, Hong J, et al. Emerging Roles of Hydrogen Sulfide in Inflammatory and Neoplastic Colonic Diseases. Front Physiol 2016;7:156. [Crossref] [PubMed]
- Grasa L, Abecia L, Peña-Cearra A, et al. TLR2 and TLR4 interact with sulfide system in the modulation of mouse colonic motility. Neurogastroenterol Motil 2019;31:e13648. [Crossref] [PubMed]
- Zhang X, Zhu W. Effects of different concentrations of hydrogen sulfide on inflammation, mitochondrial function and oxidative stress of intestinal epithelial cells. Journal of Nanjing Agricultural University 2020;43:157-63.
- Singh K, Randhwa G, Salloum FN, et al. Decreased smooth muscle function, peristaltic activity, and gastrointestinal transit in dystrophic (mdx) mice. Neurogastroenterol Motil 2021;33:e13968. [Crossref] [PubMed]
- Bagur R, Hajnóczky G. Intracellular Ca2+ Sensing: Its Role in Calcium Homeostasis and Signaling. Mol Cell 2017;66:780-8. [Crossref] [PubMed]
- Zhang W, Xu C, Yang G, et al. Interaction of H2S with Calcium Permeable Channels and Transporters. Oxid Med Cell Longev 2015;2015:323269. [Crossref] [PubMed]
- Nalli AD, Bhattacharya S, Wang H, et al. Augmentation of cGMP/PKG pathway and colonic motility by hydrogen sulfide. Am J Physiol Gastrointest Liver Physiol 2017;313:G330-41. [Crossref] [PubMed]
- Meng XM, Huang X, Zhang CM, et al. Hydrogen sulfide-induced enhancement of gastric fundus smooth muscle tone is mediated by voltage-dependent potassium and calcium channels in mice. World J Gastroenterol 2015;21:4840-51. [Crossref] [PubMed]
(English Language Editor: L. Huleatt)
Cite this article as: Liu Y, Liao R, Qiang Z, Yang W, Cao J. Exogenous H2S promotes ion channel reconstruction to regulate colonic motility in rats with dinitrobenzene sulfonic acid-induced colitis. Ann Transl Med 2022;10:681.