The delicate balance between pro-(risk of thrombosis) and anti-(risk of bleeding) coagulation during extracorporeal membrane oxygenation
During veno-venous extracorporeal membrane oxygenation (VV-ECMO), a pump drains blood from a large vein, moves it towards a membrane oxygenator, where gas exchange occurs, and then pushes it back into a large vein (1). Blood is thus continuously exposed to non-biological surfaces, including cannulas and membrane oxygenator, and high shear stress, mainly generated by the pump. Platelets and coagulation are activated so that systemic anticoagulation is needed to prevent thrombosis, inside and outside the body.
Despite continuous infusion of unfractionated heparin, visible clots are detected in 20% of adults treated with VV-ECMO. Thrombosis is usually not a fatal complication, not even when it requires urgent replacement of the membrane oxygenator (overall survival is 55%). Conversely, gastrointestinal and intracranial hemorrhages occur in 10% of the cases and are commonly lethal (overall survival declines to 34% and 20%, respectively) (2).
Herein we will briefly discuss hemostatic changes during VV-ECMO. First, we will describe a paper just published in Critical Care Medicine (3). Second, we will present our findings on this same topic. Third, we will highlight some limitations of the way we currently administer unfractionated heparin during VV-ECMO. Finally, we will present our and others ongoing research.
Hemostatic changes during VV-ECMO
Activation of platelets and coagulation during VV-ECMO depends, at least in part, on blood exposure to non-biological surfaces and high shear stress. Accordingly, it can be attenuated by covering non-endothelial surfaces with anticoagulants, by avoiding direct contact between blood and gas inside the membrane oxygenator (nowadays, gas flows inside and blood circulates outside hollow fibers) and by reducing exogenous areas and priming volumes (membrane oxygenators and circuits are becoming smaller and shorter) (4,5).
In a recent issue of Critical Care Medicine, Malfertheiner and colleagues examined the impact of three VV-ECMO systems on hemostasis. Fifty-four adults with severe acute respiratory distress syndrome (ARDS), mainly due to pneumonia, were assigned to the Cardiohelp (Maquet Cardiopulmonary AG, Rastatt, Germany), the Dideco ECC.O5 (Sorin Group) or the Deltastream system with Hilite 7000 LT + DP3 pumphead (Medos Medizintechnik AG, Rastatt, Germany). These three systems differ in non-endothelial surface covering (heparin or phosphorylcholine), type of pump (centrifugal or axial), surface area of membrane oxygenator (1.2–1.9 m2), surface area of heat exchanger (0.14–0.45 m2) and priming volumes (273–390 mL). Nonetheless, they produced analogue variations in hemostasis. During the first 5 days of ECMO, with extracorporeal blood flows typically <3.5 L/min, prothrombin fragment 1.2 and thrombin-antithrombin complex (markers of thrombin generation, i.e., activation of coagulation) and D-dimers (fibrinolysis) equally increased while factor XIII, fibrinogen and platelet count were similarly “consumed”. These changes occurred despite continuous infusion of unfractionated heparin with an activated partial thromboplastin time (aPTT) target of 50–60 sec. Doses of heparin and incidence of thrombotic and hemorrhagic complications were not reported but none of the membrane oxygenators had to be replaced during those first 5 days. Coagulation tests and platelet count tended to return normal after termination of ECMO (3).
These results are only partly in line with what we have observed in 12 other adults similarly treated with VV-ECMO. Unfractionated heparin was infused with an aPTT ratio (patient-to-normal clotting time) target of 1.5–2.0. No significant change occurred in several hemostatic assays, including prothrombin fragment 1.2, D-dimers, factor XIII and fibrinogen, over the first 7 days of ECMO, except for a constant decline in platelet count. Two additional global coagulation tests [thromboelastography (TEG) and thrombin generation] were not consistent with endogenous hypercoagulation (6).
Different findings possibly reflect different study populations. Malfertheiner and colleagues (3) enrolled subjects with mainly infective ARDS that largely alters coagulation and inflammation (7). By contrast, we enrolled many subjects with acute exacerbation of chronic obstructive pulmonary disease that is reasonably associated with less severe inflammation. One may then conclude that hemostatic changes during “modern” VV-ECMO depend primarily on factors other than configuration of the system, possibly including underlying disease.
Our current approach to anticoagulation during VV-ECMO
At our institution, unfractionated heparin is infused according to a standardized protocol (Figure 1). We administer a bolus (50–70 IU/kg of actual body weight, IV) at the time of insertion of vascular cannulas and then a continuous infusion (starting with 18 IU/kg, IV) with an aPTT ratio target of 1.5–2.0. Specific blood products are transfused if antithrombin activity is <70%, hemoglobin is <10 g/dL, fibrinogen is <150 mg/dL, platelet count is <45,000 cell/mm3 (<80,000 cell/mm3 in case of recent surgery) or prothrombin time ratio is >1.5.
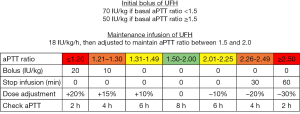
We rely on aPTT rather than activated clotting time (ACT) because it possibly better reflects heparin dosage (8) and anti-factor Xa activity (the gold standard for monitoring the anticoagulant effect of heparin) (6) during VV-ECMO. One single-center retrospective study reported fewer, potentially lethal, bleeding complications in pediatric patients receiving ECMO when heparin infusion was guided by aPTT compared to ACT (8).
Even so, aPTT is not the ideal test for monitoring systemic anticoagulation during ECMO. It is time consuming, it is not well standardized and it is not routinely available at the bedside. The target we use is derived from guidelines for preventing thrombosis in other settings and, even there it is supported by weak evidence (9). Using a similar target in subjects with hardly predictable concomitant changes in hemostasis could result in unacceptably frequent bleeding. The correlation between aPTT and heparin dosage or anti-factor Xa activity, although significant, is weak (6,8). aPTT does not provide any insight on other critical variables such as factor XIII activity and platelet count and function.
To overcome some of these limitations, one could rely on additional tests, including anti-factor Xa activity (10) or, maybe, on point-of-care assays that better assess whole blood hemostasis, such as TEG (see below).
We consider replacing membrane oxygenators whenever at least two of the following criteria are met: (I) sharp increase in D-dimers; (II) otherwise unexplained large decline in platelets; (III) membrane oxygenator dysfunction; (IV) detection of large clots (10). Again, this practice is not evidence-based. Clinical suspicion alone may lead to (hazardous) replacement of membrane oxygenators that do not contain any large clot, as judged by multi-detector computed tomography (11).
Overall, it is our experience that using a protocol, as the one described above, ensures uniform decision and facilitates achievement and maintenance of anticoagulation target over time. However, one has to bear in mind that commonly available tests are not perfect. We strongly advise discussion among staff members, possibly including an expert in coagulation, whenever management of anticoagulation calls for critical decisions.
Ongoing and future research
TEG is a point-of-care test that graphically displays the viscoelastic properties of whole blood as it clots ex-vivo. It can be used to guide transfusion of blood products in bleeding patients, especially after trauma or during cardiac surgery (12). It can also be used to examine changes in coagulation, including fibrinolysis, during sepsis (13).
The first component of the graphical output generated by TEG is known as “clotting reaction (R) time”. It represents the delay between activation of coagulation and initiation of clotting. R-time mainly depends on the balance between pro- and anti-coagulant factors and it is typically prolonged by heparin. By comparing values obtained with or without heparinase one can assess the anticoagulant effect of heparin (12).
We have recently studied the relationship between aPTT and R-time in 316 blood samples taken from 32 patients treated with VV-ECMO (14). When aPTT ratio was in target (1.5–2.0), R-time was unexpectedly high (>24 minutes, without heparinase) 80% of the times. This finding might indicate that current aPTT ratio target frequently results in excessive anticoagulation and risk of bleeding. Based on this premise, we are evaluating safety and feasibility of infusing unfractionated heparin with a R-time target (without heparinase) of 16–24 minutes during VV-ECMO (NCT02271126).
Finally, two other exciting areas of ongoing research deserve a comment. One relates to changes in platelet function during VV-ECMO. In fact, bleeding is exceptionally more common in patients treated with ECMO than in those receiving systemic anticoagulation for other reasons (15). Factors other than unfractionated heparin infusion could explain this finding. Saini and coworkers have recently noted that more than 75% of blood samples obtained from 24 children treated with ECMO had signs of severe platelet dysfunction predictive of bleeding and death (16). The other one concerns alternative ways to provide systemic anticoagulation during ECMO. Fibrin formation is normally initiated by factor XII, in response to blood contact with non-endothelial surfaces (intrinsic pathway of coagulation), or by tissue factor, in response to tissue disruption (extrinsic pathway). In rabbits undergoing 6 hours of ECMO, antibodies directed against activated factor XII prevented thrombosis of membrane oxygenators as efficiently as heparin, but without increasing bleeding from wounds (17,18).
In conclusion, systemic anticoagulation is needed during VV-ECMO and it strongly impacts on outcome. Further research is needed to optimize its use, with the aim of minimizing risk of thrombosis without increasing that of (potentially fatal) bleeding.
Acknowledgements
We are grateful to Andrea Artoni (Fondazione IRCCS Ca’ Granda—Ospedale Maggiore Policlinico, Milan, Italy) for help with manuscript preparation.
Footnote
Provenance: This is a Guest Editorial commissioned by Guest Editor Zhongheng Zhang, MD (Department of Critical Care Medicine, Jinhua Municipal Central Hospital, Jinhua Hospital of Zhejiang University, Jinhua, China).
Conflicts of Interest: The authors have no conflicts of interest to declare.
References
- Brodie D, Bacchetta M. Extracorporeal membrane oxygenation for ARDS in adults. N Engl J Med 2011;365:1905-14. [Crossref] [PubMed]
- Murphy DA, Hockings LE, Andrews RK, et al. Extracorporeal membrane oxygenation-hemostatic complications. Transfus Med Rev 2015;29:90-101. [Crossref] [PubMed]
- Malfertheiner MV, Philipp A, Lubnow M, et al. Hemostatic Changes During Extracorporeal Membrane Oxygenation: A Prospective Randomized Clinical Trial Comparing Three Different Extracorporeal Membrane Oxygenation Systems. Crit Care Med 2015. [Epub ahead of print]. [Crossref] [PubMed]
- Knoch M, Köllen B, Dietrich G, et al. Progress in veno-venous long-term bypass techniques for the treatment of ARDS. Controlled clinical trial with the heparin-coated bypass circuit. Int J Artif Organs 1992;15:103-8. [PubMed]
- Khoshbin E, Roberts N, Harvey C, et al. Poly-methyl pentene oxygenators have improved gas exchange capability and reduced transfusion requirements in adult extracorporeal membrane oxygenation. ASAIO J 2005;51:281-7. [Crossref] [PubMed]
- Panigada M, Artoni A, Passamonti SM, et al. Hemostasis changes during veno-venous extracorporeal membrane oxygenation for respiratory support in adults. Minerva Anestesiol 2015. [Epub ahead of print]. [PubMed]
- Kinasewitz GT, Yan SB, Basson B, et al. Universal changes in biomarkers of coagulation and inflammation occur in patients with severe sepsis, regardless of causative micro-organism Crit Care 2004;8:R82-90. [ISRCTN74215569]. [Crossref] [PubMed]
- Maul TM, Wolff EL, Kuch BA, et al. Activated partial thromboplastin time is a better trending tool in pediatric extracorporeal membrane oxygenation. Pediatr Crit Care Med 2012;13:e363-71. [Crossref] [PubMed]
- Eikelboom JW, Hirsh J. Monitoring unfractionated heparin with the aPTT: time for a fresh look. Thromb Haemost 2006;96:547-52. [PubMed]
- Northrop MS, Sidonio RF, Phillips SE, et al. The use of an extracorporeal membrane oxygenation anticoagulation laboratory protocol is associated with decreased blood product use, decreased hemorrhagic complications, and increased circuit life. Pediatr Crit Care Med 2015;16:66-74. [Crossref] [PubMed]
- Panigada M, L'Acqua C, Passamonti SM, et al. Comparison between clinical indicators of transmembrane oxygenator thrombosis and multidetector computed tomographic analysis. J Crit Care 2015;30:441.e7-13.
- Whiting P, Al M, Westwood M, et al. Viscoelastic point-of-care testing to assist with the diagnosis, management and monitoring of haemostasis: a systematic review and cost-effectiveness analysis. Health Technol Assess 2015;19:1-228. v-vi. [Crossref] [PubMed]
- Panigada M, Zacchetti L, L'Acqua C, et al. Assessment of Fibrinolysis in Sepsis Patients with Urokinase Modified Thromboelastography. PLoS One 2015;10:e0136463. [Crossref] [PubMed]
- Panigada M, Iapichino G, L'Acqua C, et al. Prevalence Of "Flat-Line" thromboelastography during extracorporeal membrane oxygenation for respiratory failure in adults. ASAIO J 2015. [Epub ahead of print]. [Crossref] [PubMed]
- Palareti G, Leali N, Coccheri S, et al. Bleeding complications of oral anticoagulant treatment: an inception-cohort, prospective collaborative study (ISCOAT). italian study on complications of oral anticoagulant therapy. Lancet 1996;348:423-8. [Crossref] [PubMed]
- Saini A, Hartman ME, Gage BF, et al. Incidence of Platelet dysfunction by thromboelastography-platelet mapping in children supported with ECMO: a pilot retrospective study. Front Pediatr 2016;3:116. [Crossref] [PubMed]
- Larsson M, Rayzman V, Nolte MW, et al. A factor XIIa inhibitory antibody provides thromboprotection in extracorporeal circulation without increasing bleeding risk. Sci Transl Med 2014;6:222ra17.
- Dobrovolskaia MA, McNeil SE. Safe anticoagulation when heart and lungs are "on vacation". Ann Transl Med 2015;3:S11. [PubMed]