Crosstalk between the gut microbiome and clinical response in locally advanced thoracic esophageal squamous cell carcinoma during neoadjuvant camrelizumab and chemotherapy
Introduction
Esophageal carcinoma (EC) is a major global health problem, and ranks seventh in terms of incidence (604,000 new cases) and sixth in overall mortality (544,000 deaths). Eastern Asia exhibits the highest regional incidence rates for both men and women, and esophageal squamous cell carcinoma (ESCC) causes the large burden on China (1). The most common histological subtypes are squamous cell carcinoma (SCC) and adenocarcinoma (AC), with esophageal squamous cell carcinoma (ESCC) accounting for about 90% of EC cases in China. Immune-checkpoint inhibitor (ICI) treatment, especially programmed cell death protein 1 (PD-1)/programmed cell death-ligand 1(PD-L1) antibody has altered the treatment strategy for a variety of tumors, including hematologic malignancy, advanced-stage melanoma, non-small cell lung cancer (NSCLC), small cell lung cancer (SCLC), and renal cell cancer (RCC).
Pembrolizumab plus chemotherapy has been shown to improve the overall survival (OS) of patients with previously untreated advanced ESCC and a PD-L1 combined positive score (CPS) of ≥10 (2). Furthermore, it has also been demonstrated that nivolumab adjuvant therapy can prolong the disease-free survival (DFS) of patients who had received neoadjuvant chemoradiotherapy in phase III clinical trials (3). There are multiple ongoing clinical trials aiming to evaluate the safety and efficacy of neoadjuvant PD-1/PD-L1 antibody immunotherapy in ESCC (4-6). It has been reported that the pathological complete response (pCR) rate with the application of combined neoadjuvant PD-1/PD-L1 antibody immunotherapy and chemotherapy is 31.4–42.5% (5-7), and the major pathological response (MPR) rate is about 54.9% (5). Also, the rate of ≥ grade 3AEs in patients receiving this treatment was high (11–53.3%) (5,6).
Researchers have attempted to identify biomarkers that could predict the efficacy and adverse reactions to immunotherapy, including PD-L1 CPS, PD-L1 tumor proportion score (TPS), microsatellite instability (MSI), and tumor mutation burden (TMB). Furthermore, additional biomarkers, such as interferon signatures (8), major histocompatibility complex (MHC) status (9), and immune infiltrates (10), are also under investigation. However, at present, consensus remains elusive.
In recent years, a link between human gut microbiota and the clinical responses to PD-1/PD-L1 antibody immunotherapy has emerged. A growing evidence suggests a critical role of the gut microbiota in assisting PD-1/PD-L1 antibody immunotherapy in melanoma, NSCLC, RCC, urothelial cancer, gastrointestinal cancer, and hepatocellular carcinoma (HCC) (11-14), but it has received little attention in patients receiving PD-1 antibody for esophageal cancer, especially neoadjuvant immunotherapy. In healthy individuals, the gut microbiota helps to maintain the gut barrier and immune homeostasis, and may also promote anti-cancer immune surveillance through tumor antigenicity and adjuvanticity (15). The microbiota composition in tumor tissues of ESCC patients were significantly different from that of patients with physiological normal tissues (16). The gut microbiota or its products can mimic tumor antigens and cause T cells to be primed locally before migrating to remote lymph nodes (17). Moreover, it can also trigger systemic innate immune responses via pattern recognition receptors (18), which activate host responses against tumor cells. Although it has been shown that the abundance, diversity, types, and proportion of gut microbiota is related to the clinical response and immune-related AEs (14,19), the clinical benefits of gut microbiota during PD-1/PD-L1 antibody immunotherapy is inconsistent and even contradictory between different patient cohorts, types of cancer, clinical stages, combination of treatment regimens, and so on (11,13,14). Therefore, we analyzed the gut microbiomes (from fecal samples) of patients with ESCC during neoadjuvant PD-1/PD-L1 antibody immunotherapy plus chemotherapy followed by surgery, in order to elucidate the interaction between the gut microbiome and clinical characteristics. We present the following article in accordance with the MDAR reporting checklist (available at https://atm.amegroups.com/article/view/10.21037/atm-22-1165/rc).
Methods
Patient cohort
Patients were considered eligible based on the following criteria: (I) aged 18–75 years; (II) had histopathologically confirmed thoracic ESCC; (III) the upper border of the lesion was above 20 cm from incisors; (IV) treatment-naive; (V) had T2–4aNanyM0 or T1N1–3M0 stage disease according to American Joint Committee on Cancer (AJCC) TNM system (20); (VI) had an Eastern Cooperative Oncology Group (ECOG) performance status of 0 or 1; and (VII) had at least one measurable lesion according to the Response Evaluation Criteria in Solid Tumors version 1.1 (RECIST 1.1). The key exclusion criteria were as follows: (I) patients with lymph node metastases in the supraclavicular and cervical regions; (II) those with autoimmune and interstitial lung diseases; and (III) patients receiving immunosuppressive therapy. Patients with a high risk of esophageal perforation or active hemorrhage were also excluded. The study was conducted in accordance with the Declaration of Helsinki (as revised in 2013). This study was approved by the Ethics Committee of Zhejiang Cancer Hospital (IRB-2020-320), and written informed consent was provided by all patients and their families. The study is registered with ClinicalTrials.gov, number NCT04506138.
Fecal sample collection and deoxyribonucleic acid (DNA) extraction
Fecal samples were collected using aseptic procedures at the following time points: within 3 days before neoadjuvant therapy, three days before surgery, and at the first postoperative defecation. The samples were dissolved in into fecal DNA preservation fluid (Simgen, 4103100, Hangzhou, China), transported to the laboratory, and stored at −8 ℃ for subsequent processing. Total genomic DNA samples were extracted using the OMEGA Soil DNA Kit (M5635-02) (Omega BioTek, Norcross, GA, USA). The quantity and quality of extracted DNA were measured using a NanoDrop NC2000 spectrophotometer (Thermo Fisher Scientific, Waltham, MA, USA) and agarose gel electrophoresis, respectively.
Gut microbiota analysis
Bacterial 16S ribosomal ribonucleic acid (rRNA) sequencing and taxonomic profilin
Polymerase chain reaction amplification of the bacterial 16S rRNA genes V3–V4 region was performed using the forward primer 338F (5'-ACTCCTACGGGAGGCAGCA-3') and the reverse primer 806R (5'-GGACTACHVGGGTWTCTAAT-3'). Sample-specific 7-bp barcodes were incorporated into the primers for multiplex sequencing. Polymerase chain reaction amplicons were purified with Vazyme VAHTSTM DNA Clean Beads (Vazyme, Nanjing, China), and quantified using the Quant-iT PicoGreen ds DNA Assay Kit (Invitrogen, Carlsbad, CA, USA). After the individual quantification step, amplicons were pooled in equal amounts, and pair-end 2×250 bp sequencing was performed using the sequencer (Illlumina NovaSeq 6000, San Diego, CA, USA) and kit (Illlumina NovaSeq Reagent Kit v3, San Diego, CA, USA).
Microbiota bioinformatics analysis
Microbiota bioinformatics analyses were performed with QIIME2 (21) with slight modification according to the official tutorials (https://docs.qiime2.org/2019.4/tutorials/). Sequence data analyses were mainly performed using QIIME2 and R packages (v3.2.0, The R Foundation for Statistical Computing). Amplicon sequence variant (ASV)-level alpha diversity indices, such as the Chao1 enrichment estimator, observed species, Shannon diversity index, Simpson index, Faith’s PD, Pielou’s evenness, and Good’s coverage, were calculated using the ASV table in QIIME2 and visualized as box plots. The taxonomy compositions and abundances were visualized using MEGAN (22) and GraPhlAn (23). A Venn diagram was generated to visualize the shared and unique ASVs among samples or groups using the R package “VennDiagram”, based on the occurrence of ASVs between samples/groups regardless of their relative abundance (24). Taxa abundances at the ASV levels were statistically compared among samples or groups by MetagenomeSeq (25), and visualized as Manhattan plots (26). Linear discriminant analysis effect size (LEfSe) was performed to detect differentially-abundant taxa between groups using the default parameters (27).
Statistical analysis
We choose 13% as null proportion and 30% as alternative proportion. The power value and two-side alpha value is defined as 0.8 and 0.05. Regarding the exploratory nature of this study, a sample size of 46 patients was calculated by PASS version 15.0.5 (NCSS, LLC. Kaysville, UT, USA). Continuous variables in accordance with normal distribution were presented as mean and standard deviation (SD), otherwise were presented as median (range or interquartile range). The ratios of cells with different phenotypes to total cell number were recalculated by logarithmic conversion because they were no in accordance with normal distribution. Continuous variables were compared by t-test or Kruskal-Wallis rank test, as appropriate. Categorical variables were presented as frequency and percentage, and compared by Fisher’s exact test. In cluster analysis the parameters of were single linkage and Euclidean, and the variable was the percentage of residual tumor in primary lesion. Two-sided P<0.05 was considered statistically significant. All statistical analyses were performed using STATA version 15.1 (StataCorp, College Station, TX, USA).
Results
Patient characteristics
From August 11, 2020 to February 8, 2021, 51 patients with ESCC were assessed for eligibility. One patient refused immunotherapy, one had a history of renal cancer, one had cervical lymph node metastasis, one was inoperable as their tumor was located in the cervical esophagus, and one could not be diagnosed pathologically despite repeated endoscopic biopsies. Finally, 46 were enrolled in this study. Most patients were male [44 (95.7%)] (Table 1), and the mean age of patients was 63.3±5.96 years. Forty-five patients completed two cycles of neoadjuvant therapy and 37 patients underwent esophagectomy after neoadjuvant treatment. Eight patients had pCR and 18 patients had MPR (Figure 1).
Table 1
Characteristics (n=37) | Total | pCR | Non-pCR | P value |
---|---|---|---|---|
Age (years) | 0.401 | |||
Mean | 63.3 | 61.6 | 63.7 | |
SD | 5.96 | 6.55 | 5.96 | |
Sex | 0.390 | |||
Male | 35 (94.6%) | 7 | 28 | |
Female | 2 (5.4%) | 1 | 1 | |
BMI (kg/m2) | 0.581 | |||
Mean | 21.52 | 21.16 | 21.61 | |
SD | 2.01 | 2.19 | 1.99 | |
Drinking | 0.631 | |||
Yes | 30 | 6 | 24 | |
No | 7 | 2 | 5 | |
Smoking | 0.649 | |||
Yes | 28 | 7 | 21 | |
No | 9 | 1 | 8 | |
Clinical stage | 0.404 | |||
cII | 10 (27.0%) | 1 | 9 | |
cIII–cIVA | 27 (73.0%) | 7 | 20 | |
PD-L1 | 1.000 | |||
TPS <1% | 7 (19.6%) | 1 | 6 | |
TPS ≥1% | 30 (80.4%) | 7 | 23 | |
PD-L1 | 1.000 | |||
CPS <10 | 25 (65.2%) | 5 | 20 | |
CPS ≥10 | 12 (34.8%) | 3 | 9 | |
TMB (muts/Mb) | 0.311 | |||
Mean | 3.04 | 3.43 | 3.01 | |
SD | 0.93 | 1.04 | 1.01 |
pCR, pathological complete response; SD, standard deviation; BMI, body mass index; TPS, tumor proportion score; CPS, combined positive score; TMB, tumor mutation burden; PD-L1, programmed death ligand-1.
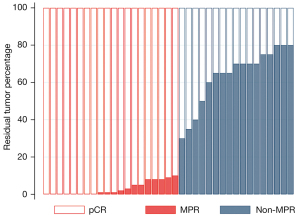
Diversity and composition of gut microbiota during neoadjuvant therapy
A total 44 fecal samples were collected within 3 days before neoadjuvant treatment, 42 were collected within 3 days before surgery, and 35 were collected at the first postoperative defecation (Table S1). A total of 676,674 valid sequences were obtained by 16S rRNA sequencing and 7,121 ASVs were obtained by clustering. A total of 32 phyla, 84 classes, 155 orders, 287 families, 633 genera, and 899 species were obtained from taxonomic annotations.
The top ten phyla were as follows: Bacteroidetes, Firmicutes, Proteobacteria, Fusobacteria, Synergistetes, Actinobacteria, Verrucomicrobia, Cyanobacteria, Lentisphaerae, and Tenericutes before neoadjuvant treatment; and Bacteroidetes, Firmicutes, Proteobacteria, Verrucomicrobia, Actinobacteria, Fusobacteria, Cyanobacteria, Synergistetes, Tenericutes, and Lentisphaerae before surgery; and Bacteroidetes, Firmicutes, Fusobacteria, Proteobacteria, Actinobacteria, Cyanobacteria, Verrucomicrobia, Synergistetes, Thermi, and Planctomycetes after surgery (Figure 2A-2C).
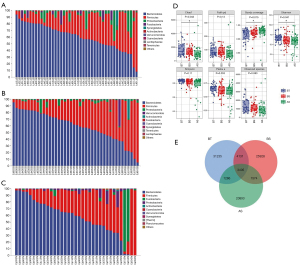
Statistically significant differences were observed in the ASV-level alpha diversity indices, including Chao1, Shannon, and Good’s coverage, between the three time points (Figure 2D). The indices decreased gradually after neoadjuvant treatment and surgery. Gut microbiome composition was different at three time points (Figure 2E). But there were no statistical differences at the three time points in the ASV-level alpha diversity indices, including the Chao1 enrichment estimator, observed species, Shannon diversity index, Simpson index, Faith’s PD, Pielou’s evenness, and Good’s coverage between pathological response (MPR or pCR), adverse event (AE) grade, TPS, CPS, and TMB.
Gut microbiome composition and association with pathological response
The composition of gut microbiota was different between the 8 patients with pCR and the 29 patients with non-pCR before neoadjuvant treatment. A difference was also observed between the 18 patients with MPR and the 19 patients without MPR. The non-pCR-enriched gut microbiota included Proteobacteria phylum [linear discriminant analysis (LDA) score 4.16, P=0.009], Dialister genus (LDA score 3.28, P=0.032), Aeromonadales order (LDA score 3.19, P=0.023), Pseudomonadales order (LDA score 2.79, P=0.041), Thermi phylum (LDA score 2.16, P=0.041), Deinococci class (LDA score 2.16, P=0.041), Moraxellaceae family (LDA score 2.13, P=0.005), Rhodocyclales family (LDA score 2.11, P=0.039), Rhodocyclaceae genus (LDA score 2.07, P=0.039), and Acinetobacter genus (LDA score 2.03, P=0.006). The non-MPR-enriched gut microbiota included Pseudomonadales order (LDA score 2.58, P=0.035) and mitochondria family (LDA score 2.42, P=0.027) (Figure 3A,3B).
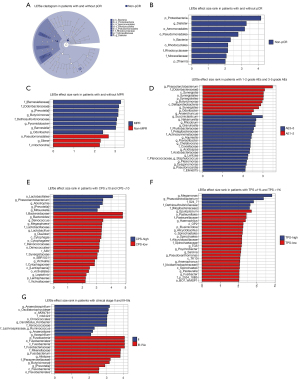
The MPR-enriched gut microbiota included Barnesiellaceae family (LDA score 3.33, P=0.003), Pyramidobacter genus (LDA score, 3.14 P=0.002), Dethiosulfovibrionaceae family (LDA score 3.13, P=0.004), Odoribacteraceae family (LDA score 3.09, P=0.012), Butyricimonas genus (LDA score 3.08, P=0.039), Prevotella genus (LDA score 3.08, P=0.011), Barnesiella genus (LDA score 3.02, P=0.002), and Odoribacter genus (LDA score 2.40, P=0.032) (Figure3C).
Gut microbiome composition and association with AEs grade
The composition of gut microbiota was different between the seven patients with ≥ grade 3 AEs and the 39 patients with grade 1–2 AEs. Patients with grade 1–2 AEs exhibited enrichment in the Phascolarctobacterium genus (LDA score 3.48, P=0.001), Odoribacteraceae family (LDA score 3.08, P=0.014), Synergistia order (LDA score 3.02, P=0.047), Synergistia class (LDA score 3.02, P=0.047), Synergistales phylum (LDA score 3.01, P=0.047), Butyricimonas genus (LDA score 2.96, P=0.013), Deltaproteobacteria class (LDA score 2.90, P=0.046), Synergistes genus (LDA score 2.79, P=0.037), Odoribacter genus (LDA score 2.53, P=0.024), and Anaerotruncus genus (LDA score 2.44, P=0.049).
Patients with ≥ grade 3 AEs exhibited enrichment in the Succiniclasticum genus (LDA score 2.98, P=0.001), Nakamurella genus (LDA score 2.65, P=0.017), Rhizobium genus (LDA score 2.62, P=0.017), Granulicella genus (LDA score 2.62, P=0.017), Phyllobacteriaceae family (LDA score 2.59, P=0.017), Pelagibacteraceae family (LDA score 2.51, P=0.017), Actinosynnemataceae family (LDA score 2.43, P=0.017), Aquirestis genus (LDA score 2.42, P=0.017), Flavisolibacter genus (LDA score 2.38, P=0.017), Chelativorans genus (LDA score 2.38, P=0.017), Coxiellaceae family (LDA score 2.37, P=0.017), Acidicapsa genus (LDA score 2.35, P=0.017), Acidobacteriaceae family (LDA score 2.33, P=0.001), Lentzea genus (LDA score 2.26, P=0.017), Staphylococcus genus (LDA score 2.24, P=0.010), Plesiomonas genus (LDA score 2.19, P=0.017), Dysgonomonas genus (LDA score 2.17, P=0.000), Pseudonocardia genus (LDA score 2.13, P=0.017), and Ellin6075 genus (LDA score 2.12, P=0.046) (Figure 3D).
Gut microbiome composition and association with PD-L1 CPS
Sixteen (34.8%) patients had CPS ≥10 and 30 (65.2%) patients had CPS <10. Patients with PD-L1 CPS ≥10 exhibited enrichment in the Lactobacillales order (LDA score 4.08, P=0.050), Abiotrophia genus (LDA score 3.48, P=0.038), Phascolarctobacterium genus (LDA score 3.39, P=0.001), and so on. Also, patients with PD-L1 CPS <10 exhibited enrichment in the Bacteroidaceae family (LDA score 4.79, P=0.023), Bacteroides genus (LDA score 4.79, P=0.023), Megasphaera genus (LDA score 3.46, P=0.003), and so on (Figure 3E).
Gut microbiome composition and association with PD-L1 TPS
Thirty-seven (80.4%) patients had PD-L1 TPS ≥1% and nine (19.6%) patients had PD-L1 TPS <1%. Patients with PD-L1 TPS ≥1% exhibited enrichment in the Megamonas genus (LDA score 3.68, P=0.020), Phascolarctobacterium genus (LDA score 3.43, P=0.006), S24_7 family (LDA score 2.83, P=0.015), and so on. Also, patients with PD-L1 TPS <1% exhibited enrichment in the Epulopiscium genus (LDA score 2.71, P=0.019), Pasteurellales order (LDA score 2.41, P=0.010), Pasteurellaceae family (LDA score 2.41, P=0.010), and so on (Figure 3F).
Gut microbiome composition and association with clinical stage
Forty-six patients were enrolled in this study, among whom 11 (23.9%) patients were clinical stage II, and 35 (76.1%) patients were stages III and IVa at baseline. Patients with clinical stage II exhibited enrichment in the AKIW781 order (LDA score 3.30, P=0.016), Xenococcaceae family (LDA score 3.12, P=0.016), Chroococcales order (LDA score 3.09, P=0.016), and so on. The patients with clinical stage III–IVa exhibited enrichment in the Fusobacterium genus (LDA score 3.83, P=0.038), Rikenellaceae order (LDA score 3.84, P=0.025), Alistipes genus (LDA score 3.82, P=0.030), and so on (Figure 3G).
Discussion
Esophageal microbiota plays important roles in esophageal cancer. Streptococcus, Prevotella and Veillonella were the dominant taxon in the healthy oesophageal mucosa in Chinese populations (28). Escherichia coli, Prevotella spp., Clostridium spp. and Bacteroides fragilis (29) significantly increased ,but Bacteroidetes, Fusobacteria, and Spirochaetes and microbial diversity (16) reduced in patients with ESCC compared with that in healthy subjects. The role of gut microbiota in patients receiving PD-1 antibody for esophageal cancer was unclear, most of the data came from clinical trials for other types of tumors. There was a trial finding that high levels of intratumoral F. nucleatum had a prognostic significance for predicting poor relapse-free survival (RFS) in patients with ESCC during neoadjuvant chemotherapy. F. nucleatum expresses FadA on the bacterial cell surface, which could bind to E-cadherin, activates b-catenin signaling, and promotes cancer cell proliferation (30). Recently, there were some clinical trials to explore the PD-1 antibody neoadjuvant immunotherapy on ESCC.
In this study, we found that the gut microbiota was related to the pathological response and AEs during treatment with a combination of neoadjuvant PD-1 antibody immunotherapy and chemotherapy for locally advanced ESCC. We observed a variation in the diversity and composition of gut microbiota during neoadjuvant therapy. Significantly higher alpha diversity in the gut microbiota have been reported in responders with PD-1/PD-L1 antibody compared to non-responders in a wide variety of tumors, including melanoma (13), gastrointestinal cancer (14), HCC (31,32), and lung cancer (11). However, the results have not always been consistent in different cohorts (19).
We found that there was no statistically significant difference in alpha diversity between patients with pCR and non-pCR. Next, we assessed the compositional differences in the gut microbiota by using LEfSe and found that Pseudomonadales was enriched in patients without pCR and those without MPR. The present study focused on HCC, and non-responders exhibited a marked increase in Proteobacteria at the third week after anti-PD-1 immunotherapy, which became predominant after 12 weeks (31). However, the ratio of Proteobacteria did not increase after neoadjuvant therapy in our study. Furthermore, we found there was no significant enrichment in patients with pCR, but five genera were enriched in patients with MPR, including Pyramidobacter, Butyricimonas, Prevotella, Barnesiella, and Odoribacter. Most genera belonged to Bacteroidales, which were related with a significantly better response, progression-free survival (PFS), and OS (19,32). Prevotella was related with high PD-L1 CPS, which maybe leaded to the phenomena of its enrichment in patients with MPR have enrichment. Proteobacteria was enriched in patients without pCR (Figure 3A), which represent a highly diverse class of bacteria with numerous metabolic pathways involved.
Previous studies have reported that patients who exhibited a clinical benefit had a high relative abundance of Ruminococcaceae (13,14), Prevotella (11,14), Lachnospiraceae (14), Lactobacillus (3,6), Bifidobacterium (11,31), Akkermansia muciniphila (33), and so on. The exact mechanisms of gut microbes on clinical response are not well understood. Gut microflora could regulate cancer development through Inflammatory reaction, Immune reaction, specific protein activation, carcinogenic metabolite production (34). It’s important that gut microbes have broader effects on immune cells, such as, dendritic cells (DCs), natural killer (NK) cell, and the subset of T cell (CD4+T cell, CD8+T cell or Th17 cells) in tumor microenvironment. Those immune cells have the expression of PD-L1 and were the important effector cells in PD-1 antibody therapy.
However, the compositional differences were inconsistent. This variation can be attributed to the trial design, including aspects such as types of cancer, clinical stage, combination of treatment regimen, research endpoint, or bioinformatics analysis. Previous studies (14,31) have focused on advanced or metastatic tumors, and the responses were evaluated by imaging examinations. Our trial focused on patients with locally advanced ESCC who received only two cycles of neoadjuvant chemotherapy plus immunotherapy before surgery, and the responses were evaluated by pathological examination. This indicates that the characteristics of gut microbiomes before neoadjuvant treatment could hopefully become a biomarker for predicting the efficacy of treatment.
AE
In this study, we also explored whether ≥ grade 3 AEs were affected by the gut microbiome composition. Five genera were associated with less severe AEs, including Phascolarctobacterium, Synergistes, Odoribacter, Anaerotruncus, and Butyricimonas, of which two belonged to Firmicute. However, the relative abundance of taxa from the Firmicutes phylum might not be a protective factor against AEs, as Succiniclasticum and Staphylococcus were enriched in patients with ≥ grade 3 AEs. Thirteen genera were associated with more severe AEs, of which five belonged to Actinobacteria, including Nakamurella, Granulicella, Acidicapsa, Lentzea, and Pseudonocardia. It was reported that the taxa enriched in patients with ≥ grade 3 AEs included Bacteroides intestinalis and Intestinibacter bartlettii. The taxa enriched in patients without ≥ grade 3 AEs were also identified, including Anaerotignum lactatifermentans and Dorea formicigenerans (19).
Gut bacterial enrichment is affected by multiple clinical factors
After identifying the differentially-enriched taxa in patients with different responses and AEs, we addressed the relationship between the gut bacterial features and clinical factors in patients with ESCC. PD-L1 CPS and TPS are biomarkers during PD-1 antibody immunotherapy. We found that Phascolarctobacterium was enriched in patients with CPS ≥10 and those with TPS ≥1%. Ruminococcus was obviously negatively associated with clinical stage, which was could lead a clinical benefit for certain types of cancer, such as melanoma, NSCLC, RCC, and HCC (11,31,33).
Our study has several limitations that should be noted. Firstly, we did not identify a correlation between gut microbiome composition and dietary history. Also, 16S rRNA gene sequence, was selected rather than metagenomic sequencing, thereby making it difficult to identify different species. Furthermore, the neoadjuvant immunotherapy plus chemotherapy only lasted for 6 weeks, and samples were collected on only three timepoints. Lastly, only one sampling was arranged after surgery, which resulted in an abnormal gut microbiome. After follow-up, the relationship between survival data and gut microbes could be analyzed. Considering the result of Checkmate 577 trial (3), PD-1 antibody was recommend as the adjuvant immunotherapy regimen, further study should be designed to include postoperative immunotherapy and set more sampling timepoints metagenomic sequencing should be selected as the research method.
In summary, we demonstrated that the diversity and composition of gut microbiota had changed during neoadjuvant immunotherapy plus chemotherapy, and identified a differential bacterial repertoire in patients experiencing favorable clinical outcomes or low-grade AEs. Our data reinforces the importance of developing diagnostic fecal tests to identify gut dysbiosis, so as to predict the efficacy or AEs in locally advanced ESCC patients with treated with neoadjuvant PD-1 antibody immunotherapy plus chemotherapy.
Conclusions
In conclusion, we found that the diversity of the gut microbiome declined after neoadjuvant PD-1 antibody immunotherapy plus chemotherapy and surgery. Patients with pCR had different types and proportions of gut microbiota before treatment compared to those without pCR. There were also differences between patients with or without ≥ grade 3 AEs. The taxonomic features of the gut microbiome are potential biomarkers that predict the pathological response and AEs.
Acknowledgments
Funding: This work was funded by the Medical and Health Technology Plan of Zhejiang Province (Nos. 2019KY330 and 2020KY057), and was supported by the Key Laboratory of Prevention, Diagnosis and Therapy of Upper Gastrointestinal Cancer of Zhejiang Province (No. 2022E10021).
Footnote
Reporting Checklist: The authors have completed the MDAR reporting checklist. Available at https://atm.amegroups.com/article/view/10.21037/atm-22-1165/rc
Data Sharing Statement: Available at https://atm.amegroups.com/article/view/10.21037/atm-22-1165/dss
Conflicts of Interest: All authors have completed the ICMJE uniform disclosure form (available at https://atm.amegroups.com/article/view/10.21037/atm-22-1165/coif). The authors have no conflicts of interest to declare.
Ethical Statement: The authors are accountable for all aspects of the work in ensuring that questions related to the accuracy or integrity of any part of the work are appropriately investigated and resolved. The study was conducted in accordance with the Declaration of Helsinki (as revised in 2013). This study was approved by the Ethics Committee of Zhejiang Cancer Hospital (IRB-2020-320), and written informed consent was provided by all patients and their families.
Open Access Statement: This is an Open Access article distributed in accordance with the Creative Commons Attribution-NonCommercial-NoDerivs 4.0 International License (CC BY-NC-ND 4.0), which permits the non-commercial replication and distribution of the article with the strict proviso that no changes or edits are made and the original work is properly cited (including links to both the formal publication through the relevant DOI and the license). See: https://creativecommons.org/licenses/by-nc-nd/4.0/.
References
- Sung H, Ferlay J, Siegel RL, et al. Global Cancer Statistics 2020: GLOBOCAN Estimates of Incidence and Mortality Worldwide for 36 Cancers in 185 Countries. CA Cancer J Clin 2021;71:209-49. [Crossref] [PubMed]
- Sun JM, Shen L, Shah MA, et al. Pembrolizumab plus chemotherapy versus chemotherapy alone for first-line treatment of advanced oesophageal cancer (KEYNOTE-590): a randomised, placebo-controlled, phase 3 study. Lancet 2021;398:759-71. [Crossref] [PubMed]
- Kelly RJ, Ajani JA, Kuzdzal J, et al. Adjuvant Nivolumab in Resected Esophageal or Gastroesophageal Junction Cancer. N Engl J Med 2021;384:1191-203. [Crossref] [PubMed]
- Xing W, Zhao L, Fu X, et al. A phase II, single-centre trial of neoadjuvant toripalimab plus chemotherapy in locally advanced esophageal squamous cell carcinoma. J Thorac Dis 2020;12:6861-7. [Crossref] [PubMed]
- Li J, Liu J, Li Z, et al. Camrelizumab plus chemotherapy as neoadjuvant therapy for resectable, locally advanced esophageal squamous cell carcinoma (NIC-ESCC2019): A multicenter, open-label, single-arm, phase 2 study. J Clin Oncol 2021;39:abstr 4028.
- Li Z, Liu J, Zhang M, et al. A phase II study of neoadjuvant immunotherapy combined with chemotherapy (camrelizumab plus albumin paclitaxel and carboplatin) in resectable thoracic esophageal squamous cell cancer (NICE study): Interim results. J Clin Oncol 2021;39:abstr 4060.
- Liu D, Zhang Q, Zhu J, et al. Phase-II study of toripalimab combined with neoadjuvant chemotherapy for the treatment of resectable esophageal squamous cell carcinoma. J Clin Oncol 2021;39:abstr e16029.
- Ayers M, Lunceford J, Nebozhyn M, et al. IFN-γ-related mRNA profile predicts clinical response to PD-1 blockade. J Clin Invest 2017;127:2930-40. [Crossref] [PubMed]
- Chowell D, Morris LGT, Grigg CM, et al. Patient HLA class I genotype influences cancer response to checkpoint blockade immunotherapy. Science 2018;359:582-7. [Crossref] [PubMed]
- Zheng Y, Chen Z, Han Y, et al. Immune suppressive landscape in the human esophageal squamous cell carcinoma microenvironment. Nat Commun 2020;11:6268. [Crossref] [PubMed]
- Jin Y, Dong H, Xia L, et al. The Diversity of Gut Microbiome is Associated With Favorable Responses to Anti-Programmed Death 1 Immunotherapy in Chinese Patients With NSCLC. J Thorac Oncol 2019;14:1378-89. [Crossref] [PubMed]
- Routy B, Gopalakrishnan V, Daillère R, et al. The gut microbiota influences anticancer immunosurveillance and general health. Nat Rev Clin Oncol 2018;15:382-96. [Crossref] [PubMed]
- Gopalakrishnan V, Spencer CN, Nezi L, et al. Gut microbiome modulates response to anti-PD-1 immunotherapy in melanoma patients. Science 2018;359:97-103. [Crossref] [PubMed]
- Peng Z, Cheng S, Kou Y, et al. The Gut Microbiome Is Associated with Clinical Response to Anti-PD-1/PD-L1 Immunotherapy in Gastrointestinal Cancer. Cancer Immunol Res 2020;8:1251-61. [Crossref] [PubMed]
- Zitvogel L, Ayyoub M, Routy B, et al. Microbiome and Anticancer Immunosurveillance. Cell 2016;165:276-87. [Crossref] [PubMed]
- Yang W, Chen CH, Jia M, et al. Tumor-Associated Microbiota in Esophageal Squamous Cell Carcinoma. Front Cell Dev Biol 2021;9:641270. [Crossref] [PubMed]
- Tai N, Peng J, Liu F, et al. Microbial antigen mimics activate diabetogenic CD8 T cells in NOD mice. J Exp Med 2016;213:2129-46. [Crossref] [PubMed]
- Thaiss CA, Zmora N, Levy M, et al. The microbiome and innate immunity. Nature 2016;535:65-74. [Crossref] [PubMed]
- Andrews MC, Duong CPM, Gopalakrishnan V, et al. Gut microbiota signatures are associated with toxicity to combined CTLA-4 and PD-1 blockade. Nat Med 2021;27:1432-41. [Crossref] [PubMed]
- Amin MB, Edge S, Greene F, et al. AJCC Cancer Staging Manual. 8th edition. 8 ed. New York: Springer International Publishing, 2017.
- Bolyen E, Rideout JR, Dillon MR, et al. Reproducible, interactive, scalable and extensible microbiome data science using QIIME 2. Nat Biotechnol 2019;37:852-7. [Crossref] [PubMed]
- Huson DH, Mitra S, Ruscheweyh HJ, et al. Integrative analysis of environmental sequences using MEGAN4. Genome Res 2011;21:1552-60. [Crossref] [PubMed]
- Asnicar F, Weingart G, Tickle TL, et al. Compact graphical representation of phylogenetic data and metadata with GraPhlAn. PeerJ 2015;3:e1029. [Crossref] [PubMed]
- Zaura E, Keijser BJ, Huse SM, et al. Defining the healthy "core microbiome" of oral microbial communities. BMC Microbiol 2009;9:259. [Crossref] [PubMed]
- Paulson JN, Stine OC, Bravo HC, et al. Differential abundance analysis for microbial marker-gene surveys. Nat Methods 2013;10:1200-2. [Crossref] [PubMed]
- Zgadzaj R, Garrido-Oter R, Jensen DB, et al. Root nodule symbiosis in Lotus japonicus drives the establishment of distinctive rhizosphere, root, and nodule bacterial communities. Proc Natl Acad Sci U S A 2016;113:E7996-8005. [Crossref] [PubMed]
- Segata N, Izard J, Waldron L, et al. Metagenomic biomarker discovery and explanation. Genome Biol 2011;12:R60. [Crossref] [PubMed]
- Dong L, Yin J, Zhao J, et al. Microbial Similarity and Preference for Specific Sites in Healthy Oral Cavity and Esophagus. Front Microbiol 2018;9:1603. [Crossref] [PubMed]
- Li D, He R, Hou G, et al. Characterization of the Esophageal Microbiota and Prediction of the Metabolic Pathways Involved in Esophageal Cancer. Front Cell Infect Microbiol 2020;10:268. [Crossref] [PubMed]
- Yamamura K, Izumi D, Kandimalla R, et al. Intratumoral Fusobacterium Nucleatum Levels Predict Therapeutic Response to Neoadjuvant Chemotherapy in Esophageal Squamous Cell Carcinoma. Clin Cancer Res 2019;25:6170-9. [Crossref] [PubMed]
- Zheng Y, Wang T, Tu X, et al. Gut microbiome affects the response to anti-PD-1 immunotherapy in patients with hepatocellular carcinoma. J Immunother Cancer 2019;7:193. [Crossref] [PubMed]
- Mao J, Wang D, Long J, et al. Gut microbiome is associated with the clinical response to anti-PD-1 based immunotherapy in hepatobiliary cancers. J Immunother Cancer 2021;9:e003334. [Crossref] [PubMed]
- Routy B, Le Chatelier E, Derosa L, et al. Gut microbiome influences efficacy of PD-1-based immunotherapy against epithelial tumors. Science 2018;359:91-7. [Crossref] [PubMed]
- Chen C, Chen L, Lin L, et al. Research progress on gut microbiota in patients with gastric cancer, esophageal cancer, and small intestine cancer. Appl Microbiol Biotechnol 2021;105:4415-25. [Crossref] [PubMed]
(English Language Editor: A. Kassem)