Nrf2 protects against cerebral ischemia-reperfusion injury by suppressing programmed necrosis and inflammatory signaling pathways
Introduction
Across the globe, acute stroke is the main cause of death and permanent disability (1). Ischemic cerebral stroke occurs when the arterial blood flow of brain tissue is blocked. However, the development of cerebral ischemia-reperfusion (I/R) injury involves complex pathophysiological mechanisms, including energy metabolism dysfunction, inflammation, glutamate/neurotoxin release, oxidative stress, and calcium overload (2), along with multiple cell death processes, including apoptosis, autophagy, and necroptosis (3,4).
Necroptosis or programmed necrosis is known as another form of caspase-independent programmed cell death (PCD). Research shows that when apoptotic caspases is inhibited, cells may selectively undergo necroptosis (5). In our previous study, we applied Q-VD-OPH to induce necroptosis in a middle cerebral artery occlusion/reperfusion (MCAO/R) model in rats, and the expression of the NOD-like receptor family pyrin domain-containing 3 (NLRP3) inflammasome was subsequently upregulated (6). However, the molecular mechanism of NLRP3 inflammasome activation in Q-VD-OPH-induced necroptosis in cerebral I/R injury remains unclear.
NLRP3 inflammasome is known as a multiprotein complex, composed of the NLRP3 scaffold, precursor caspase-1, and the apoptosis-associated speck-like protein (ASC) containing a caspase recruitment domain adaptor (7,8). Both exogenous and endogenous factors contribute to NLRP3 inflammasome activation, and the component parts assemble to form the above complex. This complex assembly leads to the maturation of the proinflammatory cytokine interleukin-1β (IL-1β) and IL-18 via the activation of caspase-1 (9,10). NLRP3 inflammasome is related to many inflammatory diseases, such as Alzheimer disease, cancers, type II diabetes mellitus, and atherosclerosis (11,12). Experiments have proven that NLRP3 inflammasome is a key regulator in the inflammatory process during apoptotic cell death induced by I/R injury (13,14). Studies also revealed that NLRP3 is activated by endogenous danger signals present in atherosclerotic lesions, such as oxidized low-density lipoprotein and cholesterol crystals. NLRP3 inflammasome promotes IL-1 family cytokine production in atherosclerosis. Inflammation is an important driver of atherosclerosis, which is a potential pathology of acute cerebral infarction. Therefore, NLRP3 inflammasome activation contributes to vascular inflammatory response, thus promoting the development and progress of atherosclerosis(7,8,13). However, few studies exist concerning the regulatory mechanism of NLRP3 inflammasome in necroptosis signaling pathway.
Using animal models of ischemic stroke, several studies discovered that the mechanism directing NLRP3 inflammasome activation is related to reactive oxygen species (ROS) release, potassium efflux, and lysosomal damage (2,7,13,15). Among these, the increase of ROS can induce oxidative stress and stimulate the release of calcium ions, causing endoplasmic reticulum (ER) stress, damaging organelles, and ultimately leading to apoptosis (16,17). Interestingly, nearly all NLRP3 activators can trigger ROS generation (17), and ROS inhibitors or scavengers can suppress the production of NLRP3 (18). Nrf2 is an endogenous redox sensitive transcription factor that regulates inflammation and oxidative stress through antioxidant action (17). Nrf2 belongs to the basic leucine zipper transcription factor family, which binds to the antioxidant response element (ARE) to activate transcription factors, such as, heme oxygenase-1 (HO-1), glutathione peroxidase (GPx), andNQO1, all of which protect cells from various injuries by contributing to the anti-inflammatory process through the recruitment of inflammatory cells (17,19). Studies have shown that Nrf2 has neuroprotective effect on hypoxic-ischemic brain injury by inhibiting apoptosis signal pathway (17,19). However, it is not known whether the Nrf2/ARE pathway figures prominently in the regulation of NLRP3 inflammasome in necroptosis. Therefore, further in-depth biochemical analysis regarding the relationship among Nrf2, ROS, and NLRP3 inflammasomes is needed. Necroptosis is involved in the early stage of ischemic cerebral infarction, especially in the ischemic penumbra. Effective regulation of this signaling pathway may expand the treatment time window of acute cerebral infarction (16). Therefore, the regulation of Nrf2 on this signal pathway is worth studying. This study was designed to determine the effects Nrf2-mediated inhibition of NLRP3 inflammasome, which ultimately regulates NQO1 expression and ROS generation in Q-VD-OPH-induced necroptosis in cerebral I/R injury. We present the following article in accordance with the ARRIVE reporting checklist (available at https://atm.amegroups.com/article/view/10.21037/atm-22-604/rc).
Methods
Animals
Male Sprague-Dawley rats (240–270 g, 50 days old) were obtained from the experimental animal center of Xuzhou Medical University in an environment consisting of the following controlled parameters: temperature 21±2 ℃, humidity 55–70%, and a consistent light cycle (light from 6 am to 6 pm). A protocol was prepared before the study without registration. The experiments were approved by the Ethics Committee for the Use of Experimental Animals of Xuzhou Medical University [No: SYXK(SU)2020-0048], in accordance with National Institutes of Health (NIH) guidelines for the care and use of animals.
MCAO model
Male rats were intraperitoneally anesthetized with 5% chloral hydrate. After disinfection, surgery was performed via a midline incision, and then the right external carotid artery (ECA), internal carotid artery (ICA), and common carotid artery (CCA) were separated. Approximately 18–20 mm of nylon suture was advanced through the opening of the external carotid artery and extended into the ICA until faint resistance was felt. The blood supply of the middle cerebral artery was blocked for 2 h, after which the thread was removed and reperfusion sustained for 24 h. The rats were subsequently killed, and brain tissue was collected for follow-up tests.
Animals were scored after recovery from anesthesia with the Bederson scale (5-point scale). After the MCAO model was established, rats with a score of 1–3 were included in the study. Rats that died due to anesthesia, operation, or subarachnoid hemorrhage; and those with a score of less than 1 or with a score of more than 4 were excluded from the study. Rats scoring of 1–3 grades were randomly selected for supplementation so as to ensure the required number of experimental animals. Each experiment was repeated at least 4 times with at least 5 rats in each group.
Drug administration
Rats were given a 1.0 mg/kg Q-VD-OPH (Selleck Chemicals, Houston, Texas, USA) intraperitoneal injection, 30 min before I/R. Adenosine triphosphate (ATP; Sigma-Aldrich, St. Louis, MO, USA) was unilaterally administered into the right cortex: anteroposterior (AP), −0.7 mm; mediolateral (ML), −2.0 mm; dorsoventral (DV), −2.0 mm from the bregma at a dose of 10, 100, and 500 nmol/L. N-acetylcysteine (NAC) was administered intraperitoneally at the beginning of reperfusion and again 6 h later, at a concentration of 200 mg/mL and doses of 50, 150, 250, 500 mg/kg body weight, diluted in normal saline, pH 7.4.
Lentiviral ventricular injection
Rats were placed on a stereotactic apparatus following the anesthetization described above. Lentivirus (5×108 TU/mL) preparations were infused stereotactically into the right lateral ventricle at the following coordinates: AP, −0.8 mm; ML, −1.5 mm, and DV, −4.2 mm from the bregma. Rats were fed for 14 days to achieve sufficient gene expression. Nrf2 was knocked down/overexpressed, and the interference sequence of Nrf2 was 5'-CCGAGTTACAGTGTCTTAA-3' (GeneChem Company, Shanghai, China). Nrf2 expression in the cells was then confirmed by real-time polymerase chain reaction (PCR).
Western blot assay
A bicinchoninic acid (BCA) protein assay kit (Beyotime Biotechnology, Shanghai, China) was used to quantify the proteins. After being separated by 10–12% SDS-PAGE electrophoresis, the proteins were electrotransferred onto nitrocellulose (NC) membranes (Millipore, Bedford, MA, USA). Following blocking with 5% nonfat dry milk for 2 h at room temperature, NC membranes were incubated with primary antibodies NLRP3 (1:1,000, Novus Biologicals, Littleton, CO, USA), caspase-1 (1:1,000, Santa Cruz Biotechnology, Santa Cruz, CA, USA), Nrf2 (1:1,000, Santa Cruz), NQO1 (1:1,000, Abcam, UK), IL-1β (1:1,000, Abcam), cleaved caspase-3 (1:1,000, Cell Signaling Technology, Danvers, MA, USA), and β-actin (1:10,000, Sigma-Aldrich) overnight at 4 ℃. After incubation with secondary antibodies for 2 h in a dark environment, the bands were visualized with the Odyssey Infrared Imaging System (LI-COR Biosciences, Lincoln, NE, USA).
RNA extraction and quantitative real-time reverse transcription PCR
Total RNA was extracted and purified from tissue samples by Trizol Reagent (Invitrogen, Waltham, MA, USA). After the concentration and purity of RNA were detected, real-time reverse transcription (RT-PCR) was performed using SYBR Green PCR master mix and was analyzed with the LightCycler 480 Real-Time PCR System (Roche, Basel, Switzerland). The primers for Nrf2 were as follows: forward primer 5'-ATCCAGACAGACACCAGTGGATG-3' and reverse primer 5'-GGCAGTGAAGACCGAACTTTCA-3'. GAPDH was used as an internal reference in each reaction (forward primer: 5'-GATGCTGGTGCTGAGTATGTCG-3' reverse primer: 5'-GTGGTGCAGGATGCATTGCTGACA-3').
Measurement of ROS generation
ROS was quantified with a dihydroethidium (DHE) fluorogenic probe, and brain tissue slides were incubated with 10 µM of DHE for 30 min at 37 ℃. After a wash with phosphate-buffered saline (PBS), DHE fluorescence was imaged by fluorescence microscopy. The DHE fluorescence intensity was measured by Ipp 6.0 software (Roper Technologies, Sarasota, FL, USA).
Statistics analysis
Statistical analysis was performed using Prism 6.0 software (GraphPad, San Diego, CA, USA), and all the values are presented as the mean ± SD. Statistical significance was determined with the Student’s t-test for 2 groups. One-way analysis of variance (ANOVA) was used for comparing differences among groups. Values of P<0.05 were considered significant.
Results
Negative regulation of Nrf2 on NLRP3 inflammasome activation in Q-VD-OPH-induced necroptosis following cerebral I/R injury
To detect the potential roles of Nrf2 in the activation of NLRP3 inflammasome in necroptosis signaling pathway, lentivirus-mediated Nrf2 knocked down (referred to as sh Nrf2), Nrf2 overexpress (referred to as OE-Nrf2), lentivirus vector–carrying scrambled short hairpin RNA (referred to as cont-shRNA) was injected into the right lateral ventricle of rats with a stereotactic method. At 14 days after injection, we simulated cerebral I/R injury by establishing a model of MCAO by intraluminal suture. One day after MCAO, green fluorescent protein (GFP)-tagged sh Nrf2/OE-Nrf2 was observed in the right cerebral cortex, contrasting with cont-shRNA group. Additionally, there was a significant reduction in Nrf2 expression in the cerebral cortex of the sh Nrf2 group when compared with the cont-shRNA group, while the overexpression showed the opposite trend. Results from quantitative RT-PCR were consistent with those from western blots (Figure 1A-1D). The NLRP3 inflammasome protein level was greater in the sh Nrf2 group than in the control group (Figure 1E). Meanwhile, Nrf2 overexpression following cerebral I/R injury was associated with an apparent decrease in NLRP3 inflammasome components (Figure 1F).
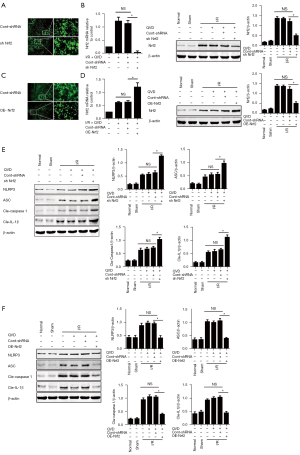
Nrf2 negatively regulated NLRP3 inflammasome by mediating NQO1 activation in necroptosis
Nrf2 was previously shown to be a negative regulator of NLRP3 inflammasome activation in necroptosis. To further understand the underlying mechanism of the Nrf2-mediated inhibition of NLRP3 inflammasome activation, we detected the expression levels of the Nrf2-targeted ARE gene, NQO1, which is an antioxidant enzyme gene that plays a critical role in scavenging ROS. We found that the expression of NQO1 was significantly reduced in the sh Nrf2 group (Figure 2A); in contrast, in the OE-Nrf2 group, the expression levels of NQO1 were increased (Figure 2B).
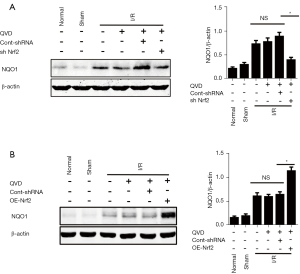
Nrf2-induced NLRP3 inflammasome inhibition was ROS dependent
To determine if Nrf2-induced NLRP3 inflammasome inhibition was ROS dependent under Q-VD-OPH-induced ischemic conditions, we used NAC and ATP as a tool for regulating ROS. NAC, a potent antioxidant, decreased ROS in a dose-dependent manner. Specifically, NAC at concentrations of 150 and 250 mg/kg showed beneficial efficacy compared with concentrations of 50 and 500 mg/kg (Figure 3A). In contrast, ATP (10, 100, and 500 nmol/L) administered into the cortex increased ROS levels. Although 10 nmol/L of ATP had little effect, 500 nmol/L of ATP induced a significant increase in ROS levels (Figure 3B). Therefore, to analyze the ROS regulation of NLRP3 inflammasome in brain I/R injury, we chose 250 mg/kg of NAC and 500 nmol of ATP as the optimum dose and concentration, respectively, for further study.
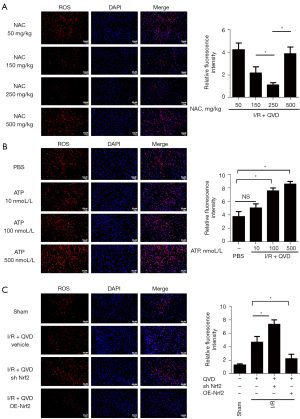
We analyzed ROS levels in OE-Nrf2 and sh Nrf2 of rat MCAO models and determined the role of ROS in Nrf2-induced NLRP3 inflammasome inhibition. Fluorescence staining test showed that the level of ROS was highly elevated in the sh Nrf2 group compared with the I/R group, while the ROS level was significantly reduced with Nrf2 overexpression (Figure 3C). We then detected the expression of NLRP3 inflammasome and IL-1β, with results indicating that the expression of NLRP3 inflammasomes, cleaved caspase-1, and cleaved IL-1β were increased in sh Nrf2 rats compared with the cont-shRNA group, which was a similar trend to that of ROS level (Figure 4A). After administration of NAC, expression levels of NLRP3 inflammasomes and cleaved IL-1β were found to be further suppressed in the sh Nrf2 + NAC group compared with the sh Nrf2 group (Figure 4A). However, ATP treatment after Nrf2 silencing significantly enhanced I/R-induced NLRP3 inflammasome, cleaved caspase-1, and cleaved IL-1β expression, while decreased in the sh Nrf2 + NAC group compared with sh Nrf2 group (Figure 4A). We also tested the expression levels of NLRP3 inflammasome in OE-Nrf2 rats. Results showed that I/R-induced elevation of NLRP3 inflammasome and cleaved IL-1β were reduced in OE-Nrf2 rats; however, the protein levels in the OE-Nrf2 group and NAC + OE-Nrf2 group showed no significant difference. Meanwhile, protein levels of NLRP3 inflammasome, cleaved caspase-1 and cleaved IL-1β showed much greater upregulation in ATP + OE-Nrf2 group than that of the OE-Nrf2 group (Figure 4B).
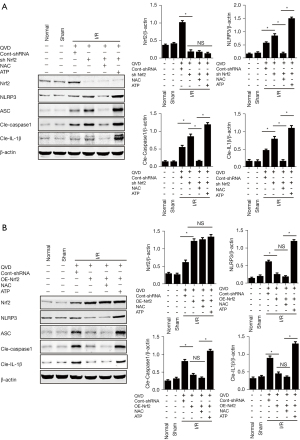
Discussion
Necroptosis, as a recently discovered form of programmed cell death, can be triggered by death receptors, such as tumor necrosis factor (TNF) and receptor 1 (TNFR1), while apoptosis is blocked (20). Increasing evidence suggests that in the absence of active caspase-8, receptor-interacting protein kinase 1 (RIPK1), RIPK3, and MLKL interact with one another to eventually induce necroptosis (5). We therefore use caspase inhibitor Q-VD-OPH as a tool in medicine.
The recovery of blood flow in the ischemic tissue after I/R injury leads to the aggravation of potentially irreversible tissue damage which often occurs in the brain, heart, liver, kidney, and lung (21). Recently, NLRP3 inflammasome was found to play a pivotal role in cerebral I/R injury. Studies have revealed that endogenous damage-associated molecular patterns (DAMPs) increase NLRP3 expression, leading to the activation of caspase-1, which in turn causes release of IL-1β, and therefore ultimately serves as a central component in the inflammatory response in ischemia stroke (6,7,12,22). Deroide et al. showed that through inhibiting NLRP3 inflammasome and the subsequent production of IL-1β, milk fat globule-EGF 8 could regulate innate immunity and subsequently reduce infarct volume and brain inflammation (23); Yang et al. reported that NLRP3 played a key role in the development of I/R-induced brain injury in NLRP3-deficient mice (24); their data clearly showed NLRP3 deficiency could reduce neurovascular damage, improve infarct volume, and attenuate neurological deficits after I/R injury (24). Although studies have revealed that NLRP3 inflammasome serves as a central component in inflammatory responses and that suppressing NLRP3 activation reduces inflammation associated with cerebral I/R injury, the mechanism and pathway involved in these processes remains elusive, especially in necroptosis signaling pathway.
Mitochondria are the major source of cellular ROS, and overgeneration of free radicals under pathophysiological conditions such as ischemia can markedly promote ROS production, resulting in oxidative stress (17,25,26). The Kelch-like ECH-associated protein 1(KEAP1)/Nrf2/ARE signaling pathway is known to regulate anti-inflammatory gene expression, thus inhibiting inflammation (19). KEAP1 is a substrate adaptor for cullin-based E3 ubiquitin ligase. Under oxidative or chemical stress, the activated Nrf2 translocates into the nucleus through its dissociation from KEAP1 and interacts with ARE to activate transcription of antioxidant enzyme genes (27) such as HO-1 and NQO-1 (28), thus inhibiting the generation of ROS. We can also conclude that Nrf2 positively regulates NQO1, as their gene and protein expression levels are consistent.
In a previous study, we demonstrated that the NLRP3 inflammasome participated in Q-VD-OPH-induced necroptosis after cerebral I/R injury (6), yet a precise mechanism was not confirmed. Therefore, in this study, we endeavored to elucidate the mechanism involved in NLRP3 inflammasomes activation in necroptosis. We consequently demonstrated that Nrf2 could inhibit NLRP3 inflammasome activation in a ROS-dependent manner in necroptosis following cerebral ischemia.
Our study furthered found the increased expression of NLRP3 inflammasomes in sh Nrf2 rats as compared to control group rats. In contrast, in OE-Nrf2 rats, the expression levels of NLRP3 inflammasomes and IL-1β were decreased, indicating that Nrf2 is a negative regulator of NLRP3 inflammasome. Other research has demonstrated that the high expression of certain Nrf2 activators inhibit inflammasome activation (29). In addition, it was also reported that Nrf2 activators exert anti-inflammatory effects by altering the expression levels of proinflammatory cytokines (30,31), which is in agreement with our results. NQO1, an antioxidant gene, protect cells from various injuries due to its anti-inflammatory effects, thus affecting the progress of disease (24). Notably, in our study, NQO1 expression was closely related to the Nrf2-mediated inhibition of NLRP3 inflammasome and thus also inhibited ROS production.
To investigate whether the anti-inflammatory effects of NQO1 were exerted via the inhibition of ROS, the expression of NLRP3 inflammasome was detected under conditions that either promoted ROS generation with ATP or inhibited ROS generation with NAC. The results showed that NAC administration decreased ROS levels and NLRP3 inflammasome, while ATP produced dissimilar outcomes.
Oxidative stress is considered to be a critical pathogenic factor of inflammatory response and is related to the imbalance of the production and clearance rate of ROS. The homeostasis of ROS plays an important role in reducing oxidative damage. The level of cellular reactive oxygen species maintains a dynamic balance in cells, which is regulated by the cellular process of producing and eliminating reactive oxygen species (32,33). Here, we found that expression of NLRP3 inflammasome was increased upon upregulation of ROS. Correspondingly, when ROS was downregulated, NLRP3 inflammasome expression was inhibited. These results were consistent across both sh Nrf2and OE-Nrf2 rats. Therefore, we can conclude that ROS activates NLRP3 inflammasomes in necroptosis. Furthermore, Nrf2-mediated inhibition of NLRP3 inflammasomes induced NQO1 activation, which in turn downregulated ROS in necroptosis signaling pathway.
Several studies have demonstrated a devastating pathophysiological process, or conversely, a potential therapeutic strategy for modulating the oxidative stress and inflammatory pathways involved in NLRP3 inflammasome activation–induced ischemic brain injury (34,35). Inhibiting the activation of NLRP3 inflammasome could inhibit the inflammatory response, thereby reducing tissue damage (36). Several NLRP3 inflammasome inhibitors that have potential clinical efficacy, including nitric oxide, small molecules, microRNAs, and type I interferon, have been promoted (8,37,38). Experimental evidence suggests that increased ROS generation, either during the ischemic phase or during the reperfusion period, can induce cell injury by disrupting cellular signal transduction and activating NLRP3 inflammasomes in cardiac diseases (39). Some studies have reported that activation of NLRP3 inflammasomes is accompanied by an increase in NLRP3 itself, as well as ASC, caspase-1, IL-1β, and IL-18 in mouse primary cortical neurons both in vitro and in vivo ischemic conditions (40,41). Another study revealed that Nlrp3−/− mice had reduced infarct volume, edema formation, and blood–brain barrier permeability preservation in a MCAO mouse model (25). Therefore, further experiments examining the NLRP3 inflammasome inhibition mechanisms in necroptosis are required to improve ischemic penumbra salvage. Our study showed that Nrf2 was an inhibitor of ROS-induced NLRP3 inflammasome activation and also caused increased expression of NQO1, an antioxidant gene, in Q-VD-OPH-induced necroptosis after cerebral I/R injury (Figure 5).
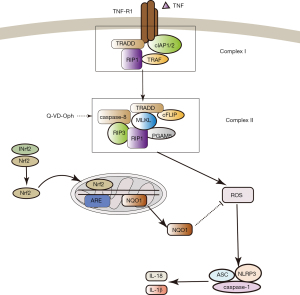
In conclusion, as necroptosis is one of the mechanisms of delayed ischemic cerebral injury, our results indicate that NLRP3 inflammasome could be a potential therapeutic target for this disease. In parallel, we also showed that Nrf2 may be of therapeutic value in NLRP3 inflammasome activation–associated diseases in necroptosis.
Acknowledgments
We would like to express our sincere thanks for the helpful advice provided by Hongyan Dong, Ting Li, and Fuxing Dong, who are from the Laboratory of Neurobiology Research Center of Xuzhou Medical University.
Footnote
Funding: This study was supported by the National Natural Science Foundation of China (No. 81501138), The Sixth Peak Talent Training Project of Jiangsu Province (No. YY-095), and the Xuzhou science and technology project (Nos. KC19027 and KC20108).
Reporting Checklist: The authors have completed the ARRIVE reporting checklist. Available at https://atm.amegroups.com/article/view/10.21037/atm-22-604/rc
Data Sharing Statement: Available at https://atm.amegroups.com/article/view/10.21037/atm-22-604/dss
Conflicts of Interest: All authors have completed the ICMJE uniform disclosure form (available at https://atm.amegroups.com/article/view/10.21037/atm-22-604/coif). WC reports that this study was supported by the National Natural Science Foundation of China (No. 81501138), The Sixth Peak Talent Training Project of Jiangsu Province (No. YY-095), and the Xuzhou science and technology project (Nos. KC19027 and KC20108). The other authors have no conflicts of interest to declare.
Ethical Statement:
Open Access Statement: This is an Open Access article distributed in accordance with the Creative Commons Attribution-NonCommercial-NoDerivs 4.0 International License (CC BY-NC-ND 4.0), which permits the non-commercial replication and distribution of the article with the strict proviso that no changes or edits are made and the original work is properly cited (including links to both the formal publication through the relevant DOI and the license). See: https://creativecommons.org/licenses/by-nc-nd/4.0/.
References
- Appleton JP, Krishnan K, Bath PM. Transdermal delivery of glyceryl trinitrate: clinical applications in acute stroke. Expert Opin Drug Deliv 2020;17:297-303. [Crossref] [PubMed]
- Duan J, Gao S, Tu S, et al. Pathophysiology and Therapeutic Potential of NADPH Oxidases in Ischemic Stroke-Induced Oxidative Stress. Oxid Med Cell Longev 2021;2021:6631805. [Crossref] [PubMed]
- D'Arcy MS. Cell death: a review of the major forms of apoptosis, necrosis and autophagy. Cell Biol Int 2019;43:582-92. [Crossref] [PubMed]
- Zille M, Ikhsan M, Jiang Y, et al. The impact of endothelial cell death in the brain and its role after stroke: A systematic review. Cell Stress 2019;3:330-47. [Crossref] [PubMed]
- Chen J, Jin H, Xu H, et al. The Neuroprotective Effects of Necrostatin-1 on Subarachnoid Hemorrhage in Rats Are Possibly Mediated by Preventing Blood-Brain Barrier Disruption and RIP3-Mediated Necroptosis. Cell Transplant 2019;28:1358-72. [Crossref] [PubMed]
- Teng X, Chen W, Liu Z, et al. NLRP3 Inflammasome Is Involved in Q-VD-OPH Induced Necroptosis Following Cerebral Ischemia-Reperfusion Injury. Neurochem Res 2018;43:1200-9. [Crossref] [PubMed]
- Sun J, Chi L, He Z, et al. NLRP3 inflammasome contributes to neurovascular unit damage in stroke. J Drug Target 2019;27:866-75. [Crossref] [PubMed]
- Alishahi M, Farzaneh M, Ghaedrahmati F, et al. NLRP3 inflammasome in ischemic stroke: As possible therapeutic target. Int J Stroke 2019;14:574-91. [Crossref] [PubMed]
- Cao Z, Wang Y, Long Z, et al. Interaction between autophagy and the NLRP3 inflammasome. Acta Biochim Biophys Sin (Shanghai) 2019;51:1087-95. [Crossref] [PubMed]
- Jiang Q, Geng X, Warren J, et al. Hypoxia Inducible Factor-1α (HIF-1α) Mediates NLRP3 Inflammasome-Dependent-Pyroptotic and Apoptotic Cell Death Following Ischemic Stroke. Neuroscience 2020;448:126-39. [Crossref] [PubMed]
- Tong Y, Wang Z, Cai L, et al. NLRP3 Inflammasome and Its Central Role in the Cardiovascular Diseases. Oxid Med Cell Longev 2020;2020:4293206. [Crossref] [PubMed]
- Wang Z, Zhang S, Xiao Y, et al. NLRP3 Inflammasome and Inflammatory Diseases. Oxid Med Cell Longev 2020;2020:4063562. [PubMed]
- Ismael S, Zhao L, Nasoohi S, et al. Inhibition of the NLRP3-inflammasome as a potential approach for neuroprotection after stroke. Sci Rep 2018;8:5971. [Crossref] [PubMed]
- Zhang J, Huang L, Shi X, et al. Metformin protects against myocardial ischemia-reperfusion injury and cell pyroptosis via AMPK/NLRP3 inflammasome pathway. Aging (Albany NY) 2020;12:24270-87. [Crossref] [PubMed]
- Eren E, Özören N. The NLRP3 inflammasome: a new player in neurological diseases. Turk J Biol 2019;43:349-59. [Crossref] [PubMed]
- Gao L, Dong Q, Song Z, et al. NLRP3 inflammasome: a promising target in ischemic stroke. Inflamm Res 2017;66:17-24. [Crossref] [PubMed]
- Liu X, Zhang X, Ding Y, et al. Nuclear Factor E2-Related Factor-2 Negatively Regulates NLRP3 Inflammasome Activity by Inhibiting Reactive Oxygen Species-Induced NLRP3 Priming. Antioxid Redox Signal 2017;26:28-43. [Crossref] [PubMed]
- Qiu Z, He Y, Ming H, et al. Lipopolysaccharide (LPS) Aggravates High Glucose- and Hypoxia/Reoxygenation-Induced Injury through Activating ROS-Dependent NLRP3 Inflammasome-Mediated Pyroptosis in H9C2 Cardiomyocytes. J Diabetes Res 2019;2019:8151836. [Crossref] [PubMed]
- Ahmed SM, Luo L, Namani A, et al. Nrf2 signaling pathway: Pivotal roles in inflammation. Biochim Biophys Acta Mol Basis Dis 2017;1863:585-97. [Crossref] [PubMed]
- Laurien L, Nagata M, Schünke H, et al. Autophosphorylation at serine 166 regulates RIP kinase 1-mediated cell death and inflammation. Nat Commun 2020;11:1747. [Crossref] [PubMed]
- Guo Z, Yu S, Chen X, et al. NLRP3 Is Involved in Ischemia/Reperfusion Injury. CNS Neurol Disord Drug Targets 2016;15:699-712. [Crossref] [PubMed]
- Dai M, Wu L, Yu K, et al. D-Carvone inhibit cerebral ischemia/reperfusion induced inflammatory response TLR4/NLRP3 signaling pathway. Biomed Pharmacother 2020;132:110870. [Crossref] [PubMed]
- Deroide N, Li X, Lerouet D, et al. MFGE8 inhibits inflammasome-induced IL-1β production and limits postischemic cerebral injury. J Clin Invest 2013;123:1176-81. [Crossref] [PubMed]
- Yang F, Wang Z, Wei X, et al. NLRP3 deficiency ameliorates neurovascular damage in experimental ischemic stroke. J Cereb Blood Flow Metab 2014;34:660-7. [Crossref] [PubMed]
- Ye M, Wang Q, Zhang W, et al. Oroxylin A exerts anti-inflammatory activity on lipopolysaccharide-induced mouse macrophage via Nrf2/ARE activation. Biochem Cell Biol 2014;92:337-48. [Crossref] [PubMed]
- Fang H, Feng Q, Shi Y, et al. Hepatic insulin resistance induced by mitochondrial oxidative stress can be ameliorated by sphingosine 1-phosphate. Mol Cell Endocrinol 2020;501:110660. [Crossref] [PubMed]
- Lin G, Sun Y, Long J, et al. Involvement of the Nrf2-Keap1 signaling pathway in protection against thallium-induced oxidative stress and mitochondrial dysfunction in primary hippocampal neurons. Toxicol Lett 2020;319:66-73. [Crossref] [PubMed]
- Wang K, Lv Q, Miao YM, et al. Cardamonin, a natural flavone, alleviates inflammatory bowel disease by the inhibition of NLRP3 inflammasome activation via an AhR/Nrf2/NQO1 pathway. Biochem Pharmacol 2018;155:494-509. [Crossref] [PubMed]
- Hennig P, Garstkiewicz M, Grossi S, et al. The Crosstalk between Nrf2 and Inflammasomes. Int J Mol Sci 2018;19:562. [Crossref] [PubMed]
- Li S, Yang H, Chen X. Protective effects of sulforaphane on diabetic retinopathy: activation of the Nrf2 pathway and inhibition of NLRP3 inflammasome formation. Exp Anim 2019;68:221-31. [Crossref] [PubMed]
- Kim GY, Jeong H, Yoon HY, et al. Anti-inflammatory mechanisms of suppressors of cytokine signaling target ROS via NRF-2/thioredoxin induction and inflammasome activation in macrophages. BMB Rep 2020;53:640-5. [Crossref] [PubMed]
- Tang YS, Zhao YH, Zhong Y, et al. Neferine inhibits LPS-ATP-induced endothelial cell pyroptosis via regulation of ROS/NLRP3/Caspase-1 signaling pathway. Inflamm Res 2019;68:727-38. [Crossref] [PubMed]
- Ye JS, Chen L, Lu YY, et al. Honokiol-Mediated Mitophagy Ameliorates Postoperative Cognitive Impairment Induced by Surgery/Sevoflurane via Inhibiting the Activation of NLRP3 Inflammasome in the Hippocampus. Oxid Med Cell Longev 2019;2019:8639618. [Crossref] [PubMed]
- Yang H, Lv H, Li H, et al. Oridonin protects LPS-induced acute lung injury by modulating Nrf2-mediated oxidative stress and Nrf2-independent NLRP3 and NF-κB pathways. Cell Commun Signal 2019;17:62. [Crossref] [PubMed]
- Espinosa-Garcia C, Atif F, Yousuf S, et al. Progesterone Attenuates Stress-Induced NLRP3 Inflammasome Activation and Enhances Autophagy following Ischemic Brain Injury. Int J Mol Sci 2020;21:3740. [Crossref] [PubMed]
- Ye Y, Jin T, Zhang X, et al. Meisoindigo Protects Against Focal Cerebral Ischemia-Reperfusion Injury by Inhibiting NLRP3 Inflammasome Activation and Regulating Microglia/Macrophage Polarization via TLR4/NF-κB Signaling Pathway. Front Cell Neurosci 2019;13:553. [Crossref] [PubMed]
- Liu A, Ding S. Anti-inflammatory Effects of Dopamine in Lipopolysaccharide (LPS)-stimulated RAW264.7 Cells via Inhibiting NLRP3 Inflammasome Activation. Ann Clin Lab Sci 2019;49:353-60. [PubMed]
- Si L, Wang H, Wang L. Suppression of miR-193a alleviates neuroinflammation and improves neurological function recovery after traumatic brain injury (TBI) in mice. Biochem Biophys Res Commun 2020;523:527-34. [Crossref] [PubMed]
- Yu J, Zhu H, Taheri S, et al. Serum Amyloid A-Mediated Inflammasome Activation of Microglial Cells in Cerebral Ischemia. J Neurosci 2019;39:9465-76. [Crossref] [PubMed]
- Wu X, Zhang H, Qi W, et al. Nicotine promotes atherosclerosis via ROS-NLRP3-mediated endothelial cell pyroptosis. Cell Death Dis 2018;9:171. [Crossref] [PubMed]
- Liu H, Wu X, Luo J, et al. Adiponectin peptide alleviates oxidative stress and NLRP3 inflammasome activation after cerebral ischemia-reperfusion injury by regulating AMPK/GSK-3β. Exp Neurol 2020;329:113302. [Crossref] [PubMed]
(English Language Editor: J. Gray)