A pan-cancer analysis of the oncogenic role of secreted phosphoprotein 1 (SPP1) in human cancers
Introduction
Molecular aberrations, such as somatic TP53 mutations in colorectal cancer (1), copy number alterations, transcriptional expression changes, and epigenetic variations (2), are believed to be the most important mechanism promoting tumorigenesis. Patients with cancer often share identical or similar genomic aberrations. These mechanisms present an opportunity to design tumor treatment strategies involving therapeutic targets that can inhibit or even cure the cancer regardless of the specific tissue in which the tumor originated (3). In this context, multiomic pan-cancer research has become a valuable approach for the exploration of shared molecular mechanisms in cancer treatment strategies (4). The Cancer Genome Atlas (TCGA) has collected and collated tumor sequencing data for most human cancer types to identify molecular aberrations at the level of DNA, RNA, protein, and epigenetics (5). This allows us to conduct a multiomic analysis of any gene and its oncogenic role in human cancers.
Secreted phosphoprotein 1 (SPP1), also known as osteopontin (OPN), is an encoded protein located at 4q22.1. This highly acidic secreted phosphoprotein has a diverse range of functions (6), including bone regeneration (7), angiogenesis (8), cell adhesion and migration (9), and inflammation (10). Emerging evidence has shown that the SPP1 gene plays an important role in tumorigenesis (11) and is associated with prognosis in multiple cancer types (12). The SPP1 gene is overexpressed in tumors such as breast carcinoma (13), hepatocellular carcinoma (14), cervical cancer (15), ovarian carcinoma (16), and lung adenocarcinoma (LUAD) (17). In cancer progression, SPP1 is involved in tumor cell growth, migration, and invasiveness, as well as chemo-resistance (18-21). In LUAD, SPP1 has been shown to mediate macrophage polarization, promote immune escape (22), and act as an immune checkpoint that induces host tumor immune tolerance by suppressing T-cell activation (23). However, the role of SPP1 in different cancers is still not clear. Pan-cancer analysis may help us to explore its role in human cancer.
Our study is the first to use public data from TCGA to conduct an SPP1 pan-cancer analysis. We collected DNA methylation data, RNA expression, immune infiltration, immunohistochemistry, protein phosphorylation, patients’ survival status, and biological pathway data to determine the role of SPP1 in human cancers. We present the following article in accordance with the MDAR reporting checklist (available at https://atm.amegroups.com/article/view/10.21037/atm-22-829/rc).
Methods
Gene expression analysis
TIMER2 (http://timer.cistrome.org/) (24) is a web tool for systematic analysis of immune infiltrates in diverse cancers. We used TIMER2 to assess the gene RNA expression level of SPP1 in tumors and normal tissues for different types of cancer. We also used GEPIA2 (http://gepia2.cancer-pku.cn/#analysis) (25) to explore the clinical and biological information of SPP1 and to conduct survival and box plots to visualize the significant difference between SPP1 expression in tumors and normal tissue. We also used GEPIA2 to create violin plots of SPP1 expression in different pathological stages of TCGA tumors. The study was conducted in accordance with the Declaration of Helsinki (as revised in 2013).
Survival prognosis analysis
The GEPIA2 database was used to analyze patients’ overall survival (OS) and disease-free survival (DFS) and to conduct a survival map of the high and low expression of SPP1 across all TCGA tumors. 95% confidence intervals (CIs) were shown in the survival plot.
DNA methylation analysis
We used the Shiny Methylation Analysis Resource Tool (SMART; http://www.bioinfo-zs.com/smartapp/) (26) to obtain the chromosomal distribution of the methylation probes. MEXPRESS (https://mexpress.be/) (27) and MethSurv (https://biit.cs.ut.ee/methsurv/) (28) were used to analyze the relationship between SPP1 RNA expression data, prognosis data, and DNA methylation data. The MEXPRESS and MethSurv websites collect TCGA data, including DNA methylation data and survival data, thus providing a convenient interface to explore the relationship between methylation of SPP1 and patient prognosis in TCGA data.
Protein phosphoprotein analysis
We used UALCAN (http://ualcan.path.uab.edu/analysis-prot.html) (29) to explore the phosphorylation level of SPP1 (with phosphorylation at the S62S63, S195, S219, S234, S254, and S258 sites; NP_1035147.1). The available data of 6 types of cancers were collected, namely LUAD, uterine corpus endometrial carcinoma (UCEC), kidney renal clear cell carcinoma (KIRC), breast invasive carcinoma (BRCA), ovarian carcinoma, and colon carcinoma.
Protein analysis by immunohistochemistry
Protein expression data provided by the Human Protein Atlas (HPA; https://www.proteinatlas.org/) (30) were detected by immunohistochemistry (IHC) staining. Protein expression was classified as high, medium, low, or not detected by referring to the stained cell proportion (>75%, 25–75%, <25%) and the staining intensity (strong, moderate, weak, and negative). The expression levels of SPP1 in tumor tissues and normal tissues used as a control were compared using antibody CAB002212.
SPP1-related gene enrichment analysis
We used GEPIA2 to obtain the top 100 SPP1-correlated targeting genes based on the datasets of 6 tumor types in which the expression of SPP1 was correlated with poor prognosis. The log2 transcript count per million (TPM), P value, and correlation coefficient (R) were visualized by dot plots. The Search Tool for the Retrieval of Interacting Genes (STRING; https://string-db.org/) is a search tool for identifying interacting genes and proteins. We employed the STRING database to generate a protein-protein interaction (PPI) network for SPP1, and the top 100 genes generated by GEPIA2 were analyzed using the STRING website algorithm. We then created a PPI network map showing the connections between the inputted proteins to identify pathways involving SPP1. Next, we combined the 2 sets of genes to conduct Kyoto Encyclopedia of Genes and Genomes (KEGG) and Gene Ontology (GO) analyses using the “clusterProfiler” package in R. The results for biological processes, cellular components (CC), and molecular function were visualized as a cnetplot and dot plot. The R software used in our study was R-4.0.2, 64-bit.
Immune infiltration analysis
We used TIMER2 to investigate whether SPP1 was involved in immune infiltration in human tumor types. The purity-adjusted Spearman’s rank correlation test was used in the TIMER2 algorithms, and results including partial correlation values and P values and were obtained. Heatmaps and scatter plots were used to visualize the results. We also used TISIDB (http://cis.hku.hk/TISIDB/) (31), an integrated repository portal for tumor-immune system interactions, to explore the relationship between SPP1 expression and immunomodulators, including immune inhibitors, immune stimulators, MHC molecules, and chemokines. Spearman’s test was performed to calculate the correlation intensity between SPP1 and immunomodulators. P values of <0.05 were considered significant in all tests. The results were visualized as heatmaps.
Statistical analysis
Median and quartile values were used to divide the high and low expression cohorts. Pearson correlation analysis was used to determine the relationship of SPP1 and other similar expressed genes. For survival analysis, A logrank test was performed, and the hazards ratio (HR) was calculated based on the Cox Proportional Hazards (PH) Model. Two-tailed P<0.05 was considered statistically significant.
Results
SPP1 expression in various cancers and normal tissues
We first analyzed the expression pattern of SPP1 in multiple tumor and normal tissue types using TIMER2 and GEPIA2. The TCGA RNA expression data showed that when compared with the corresponding control tissues, SPP1 was overexpressed in most types of cancers, such as BRCA, colon adenocarcinoma (COAD), glioblastoma multiforme (GBM), head and neck squamous cell cancer (HNSC), liver hepatocellular cancer (LIHC), cholangiocarcinoma (CHOL), LUAD, lung squamous cell cancer (LUSC), and stomach adenocarcinoma (STAD; Figure 1A). However, we also observed SPP1 downregulation in KIRC compared with normal tissues. For cancers without normal corresponding normal tissues in TIMER2, including uterine carcinosarcoma (UCS), testicular germ cell tumor (TGCT), ovarian serous cystadenocarcinoma (OV), lymphoid neoplasm diffuse large B-cell lymphoma (DLBC), and brain lower grade glioma (LGG), we assessed the expression of SPP1 using GEPIA2, which includes the normal tissue sequencing data of the Genotype-Tissue Expression (GTEx) project as controls (Figure 1B).
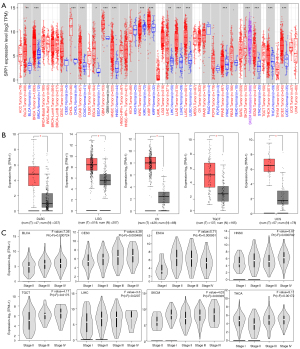
We also used GEPIA2 to explore the significance relationship between SPP1 expression and tumor pathological stages in bladder urothelial carcinoma (BLCA), cervical squamous cell carcinoma and endocervical adenocarcinoma (CESC), esophageal carcinoma (ESCA), HNSC, TGCT, LIHC, skin cutaneous melanoma (SKCM), and thyroid carcinoma (THCA; Figure 1C).
SPP1 expression correlated with survival prognosis
We divided the tumor sequencing data into high and low SPP1 RNA expression groups and investigated the correlation of SPP1 with prognosis in different tumors. As shown in Figure 2, high expression of SPP1 was linked to poor OS in adrenocortical carcinoma (ACC; HR =2.3, P=0.042), CESC (HR =1.8, P=0.014), HNSC (HR =1.3, P=0.045), LGG (HR =2.2, P=2.3e-05), LIHC (HR =2.0, P=0.00011), and LUAD (HR =1.4, P=0.015). The results showed that high expression of SPP1 was related to poor DFS in ESCA (HR =2.2, P=0.001) and LGG (HR =1.8, P=1e-04), while low expression of the SPP1 was related to poor OS in uveal melanoma (UVM; HR =0.37, P=0.023).
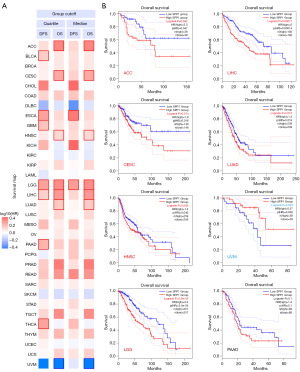
DNA methylation analysis
The MEXPRESS DNA methylation analysis results showed a significant negative correlation of SPP1 gene expression and DNA-methylated CpG sites, such as CpG site cg00088885 in ACC (R=−0.233, P<0.05), HNSC (R=−0.14, P<0.01), LGG (R=−0.238, P<0.001), LIHC (R=−0.421, P<0.001), LUAD (R=−0.249, P<0.001), and UVM (R=−0.514, P<0.001); and CpG site cg15460348 in ACC (R=−0.243, P<0.05), LIHC (R=−0.728, P<0.001), LUAD (R=−0.196, P<0.001), pancreatic adenocarcinoma (PAAD; R=−0.310, P<0.001), and UVM (R=−0.725, P<0.001). In addition, cg20261167 was also negatively associated with SPP1 expression in LGG (R=−0.101, P<0.05), LIHC (R=−0.703, P<0.001), LUAD (R=−0.156, P<0.001), PAAD (R=−0.235, P<0.01), and UVM (R=−0.708, P<0.001; Figure 3). We also analyzed the correlation between prognosis and DNA methylation of SPP1. The DNA methylation levels and survival analysis at each CpG site of SPP1 were analyzed using MethSurv. As shown in Figure 3B, hypermethylation of cg11226901 was correlated with poor prognosis in ACC (HR =0.43, P=0.038), while hypomethylation of cg15460348 was correlated with good prognosis in HNSC (HR =1.387, P=0.029). Hypermethylation of cg00088885 indicated a good prognosis in LUAD (HR =0.504, P=9.4e-09) and PAAD (HR =0.628, P=0.055), while hypermethylation of cg20261167 indicated a good prognosis in LGG (HR =0.363, P=2e-07) and LIHC (HR =0.685, P=0.03). As shown in Figure 3H, hypermethylation of 4 CpG sites in UVM were all correlated with poor prognosis (cg11226901: HR =3.929, P=0.027; cg15460348: HR =6.115, P=5e-04; cg20261167: HR =9.744, P=0.0014; cg00088885: HR =2.713, P=0.072).
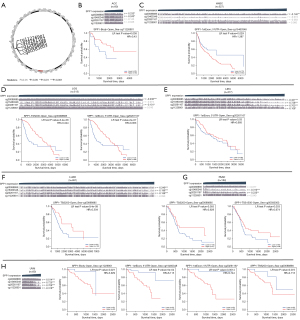
Protein phosphorylation and IHC analysis
We used the Clinical Proteomic Tumor Analysis Consortium (CPTAC) dataset to perform protein phosphorylation and IHC analysis of 4 types of tumors (BRCA, COAD, LUAD, and UCEC). The S234 of SPP1 showed higher levels of phosphorylation in tumor tissue compared with those in normal tissue (all P<0.001; Figure 4A-4D). Phosphorylation levels were also increased in S195 (P=1.84e-04), S219 (P=1.67e-04), S258 (P=6.38e-03), and S280 (P=5.67e-03) in BRCA (Figure 4A); in S62S63 (P=4.8e-08), S219 (P=1.79e-06), S254 (P=1.33e-05), and S258S263 (P=7.87e-08) in COAD (Figure 4B); in S62S63 (P=9e-19), S219 (P=1.94e-10), S258 (P=2.46e-22), and T190 (P=3.4e-15) in LUAD (Figure 4C); and in S195 (P=1.55e-05), S219 (P=6.5e-02), S254 (P=6.88e-08), and S263 (P=1.52e-05) in UCEC (Figure 4D). We also explored the protein expression of SPP1 using IHC data from the HPA in 6 tumor types in which mRNA expression was related with poor prognosis. The protein expression levels of SPP1 in tumor tissues and normal tissues were compared using antibody CAB002212. As shown in Figure 5, IHC results showed that high SPP1 protein expression was detected in both LIHC and normal liver tissue. HNSC tumor tissue showed medium staining compared with normal oral tissue, in which staining was low. SPP1 protein expression was not detected in normal lung tissue, but LUAD tumor tissue staining was medium. IHC results showed high expression of SPP1 in PAAD and CESC tumor samples, while SPP1 expression in normal pancreas and cervix tissue was medium and low, respectively.
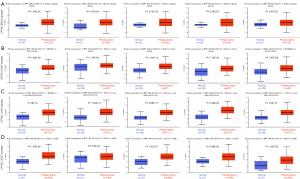
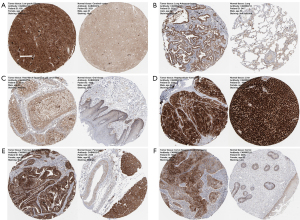
Enrichment analysis of SPP1-related genes
We used the GEPIA2 website correlation analysis algorithm to generate 100 similarly expressed genes in sample sequencing results from TCGA RNA expression data to explore potential proteins that might bind and/or influence SPP1 function. Using the STRING website, we analyzed 50 related genes and their reported or experimentally confirmed connections, which were represented by lines between the protein nodes. Figure 6A shows the relation network of the proteins, with the lines between nodes indicating evidence. These 2 datasets were combined for enrichment analysis using the KEGG and GO methods. The KEGG enrichment analysis data (Figure 6B) involved osteoclast differentiation, regulation of actin cytoskeleton, focal adhesion, and extracellular matrix (ECM)-receptor interaction. The GO enrichment analysis (Figure 6C) indicated that most of these genes were involved in immune response pathways, such as myeloid cell differentiation, leukocyte migration (Figure 6D), and neutrophil mediated immunity, etc. As shown in Figure 6E, the SPP1 expression level was positively correlated with that of hepatitis A virus cellular receptor 2 (HAVCR2; R=0.48, P=0), sialic acid-binding Ig-like lectin 9 (SIGLEC9; R=0.46, P=0), amyloid beta A4 precursor protein-binding family B member 1-interacting protein (APBB1IP; R=0.44, P=0), and linker for activation of T cells family member 2 (AT2; R=0.35, P=0).
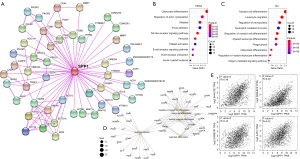
Immune infiltration and SPP1-correlated immunomodulators analysis
We analyzed immune cells infiltration level and SPP1 expression in multiple cancer types (Figure 7). A significant positive relationship was observed between SPP1 and dendritic cell infiltration in 27 cancer types. SPP1 and macrophage infiltration were positively related in 24 cancer types, and SPP1 and neutrophil infiltration were positively related in 24 cancer types. Notably, in LGG, SPP1 was significantly positively correlated with B cells (R=0.647, P<0.001), CD4+T cells (R=0.606, P<0.001), CD8+T cells (R=0.278, P<0.001), dendritic cells (R=0.685, P<0.001), macrophages (R=0.649, P<0.001), and neutrophil infiltration (R=0.639, P<0.001). Similarly, in THCA, SPP1 was positively corelated with B cells (R=0.368, P<0.001), CD4+T cells (R=0.188, P<0.001), CD8+T cells (R=0.268, P<0.001), dendritic cells (R=0.599, P<0.001), macrophages (R=0.472, P<0.001), and neutrophil infiltration (R=0.535, P<0.001). We used TISIDB to explore the relationship between SPP1, immunomodulators, and chemokines. As shown in the heatmap in Figure 7C, SPP1 was positively corelated with 14 immune inhibitors, including HAVCR2, CSF1R, galectin-9 (LGALS9), programmed cell death protein 1 (PDCD1), and transforming growth factor beta 1 (TGFB1). SPP1 was positively corelated with HAVCR2 and colony stimulating factor 1 receptor (CSF1R) in 24 and 27 cancer types, respectively. In terms of immune simulators, there was significant positive correlation between SPP1 and inducible t cell costimulator ligand (ICOSLG), cluster of differentiation 86 (CD86) in LGG, and tumor necrosis factor ligand superfamily member 4 (TNFSF4) in rectum adenocarcinoma (READ). Additionally, SPP1 was positively correlated with almost all major histocompatibility complex (MHC) molecules in LGG, OV, and THCA, including HLA-DMA, HLA-DMB, HLA-DOA, and HLADPA1. Chemokines showed various relationships with SPP1 expression. Chemokine ligand 16 (CXCL16) was positively associated with SPP1 in LGG, TGCT, and THCA. Chemokine ligand 7 (CCL7) was positively associated with SPP1 in COAD, ESCA, GBM, OV, and READ. All these results indicated that SPP1 plays an important role in immune infiltration and regulation in human cancers.
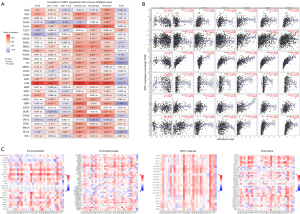
Discussion
According to the HPA database, SPP1 is widely expressed in normal human tissues, such as gallbladder, kidney, nasopharynx, colon, rectum, and caudate tissues. In this study, we observed significant overexpression of SPP1 in almost all tumor types, which indicates a potential role in tumorigenesis. It has been reported that the expression of SPP1 is higher in ovarian cancer than in normal tissues. In one study, silencing of SPP1 resulted in decreased cell proliferation activity, migration ability, and invasion ability via the integrin beta-1 (β1)/focal adhesion kinase (FAK)/protein kinase B (AKT) signaling pathway (19). Similarly, It was reported that SPP1 overexpression in colorectal cancer leads to enhanced anchorage-independent growth, cell migration ability, and invasion ability in Kirsten rat sarcoma virus (KRAS) gene mutant tumor cells and to a lesser extent in KRAS wild-type (32), promoting stem cell-like properties through the phosphoinositide 3-kinase (PI3K)/AKT/glycogen synthase kinase 3 beta (GSK-3β)/catenin beta-1 (β-catenin) pathway (33). The SPP1/PI3K/AKT signaling pathway has been noted in a variety of cancer types. In gastric cancer cells, targeting of SPP1 by miR-340 may contribute to the inhibition of proliferation, migration, invasion, and epithelial-mesenchymal transition via suppression of the PI3K/AKT signaling pathway (34). Pang et al. found that both protein and messenger RNA (mRNA) expression levels of SPP1 were remarkably upregulated in metastatic castration-resistant prostate cancer and could regulate the androgen receptor signaling pathway (35). SPP1 plays multifaceted roles in the bone microenvironment and drug resistance via binding to the alpha-v beta-3 (αvβ3) integrin and the CD44 receptor and inducing signaling cascades (36). In lung cancer, SPP1 has been observed to be significantly increased in afatinib-resistant lung cancer cells and to enhance second-generation epidermal growth factor receptor (EGFR)-tyrosine kinase inhibitor (TKI) resistance in lung cancer (37). These studies indicate that SPP1 promotes tumor progression through a variety of mechanisms.
In our results, SPP1 expression was also positively corelated with tumor stages in multiple types of cancer. The later stages in BLCA, CESC, HNSC, TGCT, LIHC, and SKCM expressed higher SPP1. Tumor patients at advanced stages have fewer treatment options and poorer prognosis than those at early stages. Chemotherapy is a common treatment for multiple types of advanced cancer patients (38-41). Targeted therapy and drug resistance are the main focus in cancer research (42). Previous studies have reported that SPP1 expression is involved in chemoresistance in various tumor types. Chen et al. found that downregulation of SPP1 improved the cisplatin sensitivity of the cervical cancer HeLa cell line by inhibiting the PI3K/AKT signaling pathway through the same mechanisms as those in the SPP1 tumor-inducing progression mentioned above (20). Li et al. found that SPP1 is highly expressed in a chemo-sensitive group of patients with laryngeal squamous cell carcinoma (43); however, there was no corresponding experiment to further confirm the results. Liu et al. reported that SPP1 is capable of promoting chemo-resistance of hepatocellular carcinoma via autophagy (21). Carbone et al. assayed the serum SPP1 from blood samples of patients with non-small cell lung cancer and found that patients with high SPP1 levels had a worse response to nivolumab and a much higher mortality rate compared to those of patients with low serum OPN levels (44). The mechanisms between SPP1 and chemotherapy need to be further explored in various types of cancers to identify new targets for the treatment of advanced cancer patients.
Gene expression can be regulated by epigenetic mechanisms without nucleotide sequence changes (45). Methylation of cytosine carbon-5 within 5'-C-phosphate-G-3' (CpG) is one of the most common epigenetic alterations and plays an important role in the regulation of gene expression. Abnormal DNA methylation orchestrates many cancer-related gene expression irregularities, such as the inactivation of tumor suppressor genes through hypermethylation and the activation of pro-metastatic genes through hypomethylation (46). We observed that the hypermethylation of SPP1 was negatively corelated with SPP1 mRNA expression and was associated with good prognosis in some cancer types, such as ACC, LGG, LIHC, LUAD, and PAAD. However, hypermethylation of SPP1 in UVM was associated with poor prognosis, corresponding to the low SPP1 mRNA expression associated with poor survival in the survival analysis results. Long et al. analyzed the relationship between SPP1 methylation and clinical characteristics in hepatocellular carcinoma and found that hypomethylation of SPP1 was significantly associated with poor prognosis and high recurrence rate (47). The same trend was also found in gastrointestinal stromal tumors (48). Therefore, SPP1 methylation might be valuable for determining the prognosis of cancer patients.
Phosphorylation is an important post-translational modification involved in many biological functions, including activation or inactivation of enzyme activity, modulation of molecular interactions, and signaling pathways. In our study, high SPP1 phosphorylation in multiple phosphorylation sites was observed in 4 tumor types when compared with that of corresponding normal tissues. S234 was consistently a high phosphorylated site in the 4 tumor types. SPP1 is a phosphorylated ECM protein that promotes cell adhesion by interacting with several integrin receptors (49) and plays a role in a variety of cellular processes from bone resorption and ECM remodeling to immune cell activation and the inhibition of apoptosis (50). Phosphorylated SPP1 has been shown to mediate the intrafibrillar mineralization of collagen and the activation of osteoclasts, as well as nucleation, growth, and precipitation of calcium phosphate (51). However, we failed to find tumorigenesis-associated mechanisms concerning the phosphorylation site S234, which may play a general and important role in cancers. Additional experiments are required to evaluate the potential role of the phosphorylation site S234 in SPP1.
Another key finding in this study is the relationship between SPP1 and the immune system. The SPP1-associated genes enrichment analysis, immune infiltration analysis, and immunomodulators correlation analysis all indicated that SPP1 plays a critical role in the regulation of immune cell function, resulting in tumorigenesis and progression. In our results, the mRNA expression of SPP1 was positively related to dendritic cells, macrophages, and neutrophils. It has been reported that high SPP1 levels and low rapamycin complex 2 (mTORC2) activity in tumor-associated macrophages correlate with a worsened clinical prognosis in colorectal cancer patients, and that mTORC2-deficient macrophages promote colitis via the cytokine SPP1 to stimulate tumor growth (52). Zhu et al. demonstrated that SPP1 facilitates chemotactic migration and alternative activation of macrophages and promotes programmed death-ligand 1 (PD-L1) expression in hepatocellular carcinoma via activation of the CSF1/CSF1R pathway in macrophages (53). The OPN/CSF1/CSF1R axis plays a critical role in the immunosuppressive nature of the hepatocellular carcinoma microenvironment. This corroborates with our results, which showed that SPP1 was positively correlated with CSF1R in 27 cancer types. Chen et al. genotyped 3 promoter SPP1 polymorphisms using DNA from blood lymphocytes and found that SPP1-443C/T polymorphism is a potential predictive marker of survival in patients with lung cancer and is significantly correlated with bone metastasis (54). Wei et al. reported that SPP1 is an important chemokine for recruiting macrophages to glioblastoma that mediates crosstalk between tumor cells and the innate immune system (55). In our correlation analysis of SPP1 and immune inhibitors, we found a significant positive relationship between SPP1 and HAVCR2 in 25 tumor types, particularly in LGG, GBM, OV, and READ. The protein encoded by HAVCR2, also known as TIM3, is a Th1-specific cell surface protein that regulates macrophage activation, inhibits Th1-mediated auto- and alloimmune responses, and promotes immunological tolerance (56). Thus, we speculate that HAVCR2 might be involved in SPP1 regulation of immune cell infiltration.
Taken together, our first pan-cancer analysis of SPP1 indicated statistical correlations of SPP1 expression with DNA methylation, patient survival, protein phosphorylation, immunomodulators, and immune cell infiltration in various cancer types, which might help us to understand the role that SPP1 plays in tumorigenesis.
Acknowledgments
Funding: This study was supported by the National Natural Science Foundation of China (No. 81773045), Medical Science and Technology Key Project of Henan Province, China (No. SBGJ202002084), and the Joint Construction Project of Henan Medical Science and Technology Project of China (No. LHGJ20190301).
Footnote
Reporting Checklist: The authors have completed the MDAR reporting checklist. Available at https://atm.amegroups.com/article/view/10.21037/atm-22-829/rc
Conflicts of Interest: All authors have completed the ICMJE uniform disclosure form (available at https://atm.amegroups.com/article/view/10.21037/atm-22-829/coif). The authors have no conflicts of interest to declare.
Ethical Statement: The authors are accountable for all aspects of the work in ensuring that questions related to the accuracy or integrity of any part of the work are appropriately investigated and resolved. The study was conducted in accordance with the Declaration of Helsinki (as revised in 2013).
Open Access Statement: This is an Open Access article distributed in accordance with the Creative Commons Attribution-NonCommercial-NoDerivs 4.0 International License (CC BY-NC-ND 4.0), which permits the non-commercial replication and distribution of the article with the strict proviso that no changes or edits are made and the original work is properly cited (including links to both the formal publication through the relevant DOI and the license). See: https://creativecommons.org/licenses/by-nc-nd/4.0/.
References
- Iranzo J, Martincorena I, Koonin EV. Cancer-mutation network and the number and specificity of driver mutations. Proc Natl Acad Sci U S A 2018;115:E6010-9. [Crossref] [PubMed]
- Liu Z, Zhang S. Toward a systematic understanding of cancers: a survey of the pan-cancer study. Front Genet 2014;5:194. [Crossref] [PubMed]
- Liu Z, Zhang S. Tumor characterization and stratification by integrated molecular profiles reveals essential pan-cancer features. BMC Genomics 2015;16:503. [Crossref] [PubMed]
- Cao Z, Zhang S. An integrative and comparative study of pan-cancer transcriptomes reveals distinct cancer common and specific signatures. Sci Rep 2016;6:33398. [Crossref] [PubMed]
- Cancer Genome Atlas Research Network. The Cancer Genome Atlas Pan-Cancer analysis project. Nat Genet 2013;45:1113-20. [Crossref] [PubMed]
- Denhardt DT, Guo X. Osteopontin: a protein with diverse functions. FASEB J 1993;7:1475-82. [Crossref] [PubMed]
- Srirussamee K, Mobini S, Cassidy NJ, et al. Direct electrical stimulation enhances osteogenesis by inducing Bmp2 and Spp1 expressions from macrophages and preosteoblasts. Biotechnol Bioeng 2019;116:3421-32. [Crossref] [PubMed]
- Zhu M, He H, Meng Q, et al. Osteopontin sequence modified mesoporous calcium silicate scaffolds to promote angiogenesis in bone tissue regeneration. J Mater Chem B 2020;8:5849-61. [Crossref] [PubMed]
- Liaw L, Skinner MP, Raines EW, et al. The adhesive and migratory effects of osteopontin are mediated via distinct cell surface integrins. Role of alpha v beta 3 in smooth muscle cell migration to osteopontin in vitro. J Clin Invest 1995;95:713-24. [Crossref] [PubMed]
- Castello LM, Raineri D, Salmi L, et al. Osteopontin at the Crossroads of Inflammation and Tumor Progression. Mediators Inflamm 2017;2017:4049098. [Crossref] [PubMed]
- Lamort AS, Giopanou I, Psallidas I, et al. Osteopontin as a Link between Inflammation and Cancer: The Thorax in the Spotlight. Cells 2019;8:815. [Crossref] [PubMed]
- Tu Y, Chen C, Fan G. Association between the expression of secreted phosphoprotein - related genes and prognosis of human cancer. BMC Cancer 2019;19:1230. [Crossref] [PubMed]
- Göthlin Eremo A, Lagergren K, Othman L, et al. Evaluation of SPP1/osteopontin expression as predictor of recurrence in tamoxifen treated breast cancer. Sci Rep 2020;10:1451. [Crossref] [PubMed]
- Pan HW, Ou YH, Peng SY, et al. Overexpression of osteopontin is associated with intrahepatic metastasis, early recurrence, and poorer prognosis of surgically resected hepatocellular carcinoma. Cancer 2003;98:119-27. [Crossref] [PubMed]
- Zhao K, Ma Z, Zhang W. Comprehensive Analysis to Identify SPP1 as a Prognostic Biomarker in Cervical Cancer. Front Genet 2022;12:732822. [Crossref] [PubMed]
- Periyasamy A, Gopisetty G, Subramanium MJ, et al. Identification and validation of differential plasma proteins levels in epithelial ovarian cancer. J Proteomics 2020;226:103893. [Crossref] [PubMed]
- Guo Z, Huang J, Wang Y, et al. Analysis of Expression and Its Clinical Significance of the Secreted Phosphoprotein 1 in Lung Adenocarcinoma. Front Genet 2020;11:547. [Crossref] [PubMed]
- Wang J, Hao F, Fei X, et al. SPP1 functions as an enhancer of cell growth in hepatocellular carcinoma targeted by miR-181c. Am J Transl Res 2019;11:6924-37. [PubMed]
- Zeng B, Zhou M, Wu H, et al. SPP1 promotes ovarian cancer progression via Integrin β1/FAK/AKT signaling pathway. Onco Targets Ther 2018;11:1333-43. [Crossref] [PubMed]
- Chen X, Xiong D, Ye L, et al. SPP1 inhibition improves the cisplatin chemo-sensitivity of cervical cancer cell lines. Cancer Chemother Pharmacol 2019;83:603-13. [Crossref] [PubMed]
- Liu G, Fan X, Tang M, et al. Osteopontin induces autophagy to promote chemo-resistance in human hepatocellular carcinoma cells. Cancer Lett 2016;383:171-82. [Crossref] [PubMed]
- Zhang Y, Du W, Chen Z, et al. Upregulation of PD-L1 by SPP1 mediates macrophage polarization and facilitates immune escape in lung adenocarcinoma. Exp Cell Res 2017;359:449-57. [Crossref] [PubMed]
- Klement JD, Paschall AV, Redd PS, et al. An osteopontin/CD44 immune checkpoint controls CD8+ T cell activation and tumor immune evasion. J Clin Invest 2018;128:5549-60. [Crossref] [PubMed]
- Li T, Fu J, Zeng Z, et al. TIMER2.0 for analysis of tumor-infiltrating immune cells. Nucleic Acids Res 2020;48:W509-14. [Crossref] [PubMed]
- Tang Z, Kang B, Li C, et al. GEPIA2: an enhanced web server for large-scale expression profiling and interactive analysis. Nucleic Acids Res 2019;47:W556-60. [Crossref] [PubMed]
- Li Y, Ge D, Lu C. The SMART App: an interactive web application for comprehensive DNA methylation analysis and visualization. Epigenetics Chromatin 2019;12:71. [Crossref] [PubMed]
- Koch A, Jeschke J, Van Criekinge W, et al. MEXPRESS update 2019. Nucleic Acids Res 2019;47:W561-5. [Crossref] [PubMed]
- Modhukur V, Iljasenko T, Metsalu T, et al. MethSurv: a web tool to perform multivariable survival analysis using DNA methylation data. Epigenomics 2018;10:277-88. [Crossref] [PubMed]
- Chandrashekar DS, Bashel B, Balasubramanya SAH, et al. UALCAN: A Portal for Facilitating Tumor Subgroup Gene Expression and Survival Analyses. Neoplasia 2017;19:649-58. [Crossref] [PubMed]
- Uhlén M, Fagerberg L, Hallström BM, et al. Proteomics. Tissue-based map of the human proteome. Science 2015;347:1260419. [Crossref] [PubMed]
- Ru B, Wong CN, Tong Y, et al. TISIDB: an integrated repository portal for tumor-immune system interactions. Bioinformatics 2019;35:4200-2. [Crossref] [PubMed]
- Wei R, Wong JPC, Lyu P, et al. In vitro and clinical data analysis of Osteopontin as a prognostic indicator in colorectal cancer. J Cell Mol Med 2018;22:4097-105. [Crossref] [PubMed]
- Cheng Y, Wen G, Sun Y, et al. Osteopontin Promotes Colorectal Cancer Cell Invasion and the Stem Cell-Like Properties through the PI3K-AKT-GSK/3β-β/Catenin Pathway. Med Sci Monit 2019;25:3014-25. [Crossref] [PubMed]
- Song SZ, Lin S, Liu JN, et al. Targeting of SPP1 by microRNA-340 inhibits gastric cancer cell epithelial-mesenchymal transition through inhibition of the PI3K/AKT signaling pathway. J Cell Physiol 2019;234:18587-601. [Crossref] [PubMed]
- Pang X, Xie R, Zhang Z, et al. Identification of SPP1 as an Extracellular Matrix Signature for Metastatic Castration-Resistant Prostate Cancer. Front Oncol 2019;9:924. [Crossref] [PubMed]
- Pang X, Gong K, Zhang X, et al. Osteopontin as a multifaceted driver of bone metastasis and drug resistance. Pharmacol Res 2019;144:235-44. [Crossref] [PubMed]
- Wang X, Zhang F, Yang X, et al. Secreted Phosphoprotein 1 (SPP1) Contributes to Second-Generation EGFR Tyrosine Kinase Inhibitor Resistance in Non-Small Cell Lung Cancer. Oncol Res 2019;27:871-7. [Crossref] [PubMed]
- Borella F, Bertero L, Morrone A, et al. Brain Metastases from Ovarian Cancer: Current Evidence in Diagnosis, Treatment, and Prognosis. Cancers (Basel) 2020;12:2156. [Crossref] [PubMed]
- Alobuia W, Gillis A, Kebebew E. Contemporary Management of Anaplastic Thyroid Cancer. Curr Treat Options Oncol 2020;21:78. [Crossref] [PubMed]
- Chung LY, Tang SJ, Wu YC, et al. Platinum-based combination chemotherapy triggers cancer cell death through induction of BNIP3 and ROS, but not autophagy. J Cell Mol Med 2020;24:1993-2003. [Crossref] [PubMed]
- Chen R, Li D, Zheng M, et al. FGFRL1 affects chemoresistance of small-cell lung cancer by modulating the PI3K/Akt pathway via ENO1. J Cell Mol Med 2020;24:2123-34. [Crossref] [PubMed]
- Zhang J, Zhang F, Niu R. Functions of Shp2 in cancer. J Cell Mol Med 2015;19:2075-83. [Crossref] [PubMed]
- Li L, Wang R, He S, et al. The identification of induction chemo-sensitivity genes of laryngeal squamous cell carcinoma and their clinical utilization. Eur Arch Otorhinolaryngol 2018;275:2773-81. [Crossref] [PubMed]
- Carbone F, Grossi F, Bonaventura A, et al. Baseline serum levels of osteopontin predict clinical response to treatment with nivolumab in patients with non-small cell lung cancer. Clin Exp Metastasis 2019;36:449-56. [Crossref] [PubMed]
- Zhu A, Hopkins KM, Friedman RA, et al. DNMT1 and DNMT3B regulate tumorigenicity of human prostate cancer cells by controlling RAD9 expression through targeted methylation. Carcinogenesis 2021;42:220-31. [Crossref] [PubMed]
- Mahmood N, Arakelian A, Cheishvili D, et al. S-adenosylmethionine in combination with decitabine shows enhanced anti-cancer effects in repressing breast cancer growth and metastasis. J Cell Mol Med 2020;24:10322-37. [Crossref] [PubMed]
- Long J, Chen P, Lin J, et al. DNA methylation-driven genes for constructing diagnostic, prognostic, and recurrence models for hepatocellular carcinoma. Theranostics 2019;9:7251-67. [Crossref] [PubMed]
- Haller F, Zhang JD, Moskalev EA, et al. Combined DNA methylation and gene expression profiling in gastrointestinal stromal tumors reveals hypomethylation of SPP1 as an independent prognostic factor. Int J Cancer 2015;136:1013-23. [Crossref] [PubMed]
- Oyama M, Kariya Y, Kariya Y, et al. Biological role of site-specific O-glycosylation in cell adhesion activity and phosphorylation of osteopontin. Biochem J 2018;475:1583-95. [Crossref] [PubMed]
- Kazanecki CC, Uzwiak DJ, Denhardt DT. Control of osteopontin signaling and function by post-translational phosphorylation and protein folding. J Cell Biochem 2007;102:912-24. [Crossref] [PubMed]
- Lenton S, Grimaldo M, Roosen-Runge F, et al. Effect of Phosphorylation on a Human-like Osteopontin Peptide. Biophys J 2017;112:1586-96. [Crossref] [PubMed]
- Katholnig K, Schütz B, Fritsch SD, et al. Inactivation of mTORC2 in macrophages is a signature of colorectal cancer that promotes tumorigenesis. JCI Insight 2019;4:124164. [Crossref] [PubMed]
- Zhu Y, Yang J, Xu D, et al. Disruption of tumour-associated macrophage trafficking by the osteopontin-induced colony-stimulating factor-1 signalling sensitises hepatocellular carcinoma to anti-PD-L1 blockade. Gut 2019;68:1653-66. [Crossref] [PubMed]
- Chen Y, Liu H, Wu W, et al. Osteopontin genetic variants are associated with overall survival in advanced non-small-cell lung cancer patients and bone metastasis. J Exp Clin Cancer Res 2013;32:45. [Crossref] [PubMed]
- Wei J, Marisetty A, Schrand B, et al. Osteopontin mediates glioblastoma-associated macrophage infiltration and is a potential therapeutic target. J Clin Invest 2019;129:137-49. [Crossref] [PubMed]
- Solinas C, De Silva P, Bron D, et al. Significance of TIM3 expression in cancer: From biology to the clinic. Semin Oncol 2019;46:372-9. [Crossref] [PubMed]
(English Language Editor: C. Gourlay)