Circulating exosomal miR-21 mediates HUVEC proliferation and migration through PTEN/PI3K/AKT in Crohn’s disease
Introduction
Crohn’s disease (CD) is a type of chronic inflammatory bowel disease (IBD) that affects the entire intestine and is characterized by recurrent diarrhea, abdominal pain, and fever that can last from days to weeks. At present, the pathogenesis of CD is not clear (1).
Angiogenesis plays an important role in inflammation and IBD pathogenesis (2). In 2009, Alkim et al. (3). found an increase in microvascular density in the intestinal tissue of patients with ulcerative colitis (UC) and CD, which was closely related to disease activity. Other researchers have confirmed these results that vessels angiogenesis was associated with CD progression using magnetic resonance imaging technology, dynamic ultrasound imaging, and narrow-band imaging techniques (4-6). Patients with CD experience long-term inflammation, crypto-ulcers, and mucosal epithelial self-repair in the intestines. The corresponding angiogenesis may be the pathological basis of CD: vascular hyperplasia supplies nutrients and oxygen to the diseased area, while blood flow perfusion removes necrotic tissue and cell metabolites. During the early stage of the disease, the balance of angiogenesis and anti-angiogenesis is disrupted and physiological vascular angiogenesis transforms into pathological angiogenesis. Whether this transformation is a secondary change in the process of self-healing or a cause of CD progression and persistence remains controversial (7). Some researchers have suggested that the angiogenesis of CD is driven by inflammation and an immune response called “immune-driven angiogenesis”, in which the microcirculation of the intestine plays a major role (8,9).
Exosomes are double-layered membrane vesicles released by the cell vomiting action. They have a diameter of 30–100 nm and contain a variety of molecules, including proteins, lipids, DNA, and RNA. Exosome contents enter cells to achieve intercellular information exchange (10,11). A variety of cell types release exosomes, including white blood cells, stem cells, endothelial cells, immune cells, platelets, and smooth muscle cells (12). Exosomes from different cell sources contain different molecules and therefore have different effects on receptor cells (13). microRNA (miRNA) is a unique class of endogenous, single-stranded, conserved, non-coding RNA fragments with a length of approximately 18–22 nucleotides. More than 2,500 mature human miRNA transcripts have been confirmed (14,15). The combination of miRNAs and messenger RNA (mRNA) targets in humans is not fully complementary, resulting in mRNA instability and translation inhibition. Researchers have found that miRNAs have a highly specific gene expression pattern and can regulate about 60% of protein-coded mRNA, as well as regulating cell functions such as differentiation, proliferation, signal transduction, and apoptosis (15). Studies have also confirmed that miRNAs can be loaded into exosomes, whose lipid envelopes ensure that miRNAs are not hydrolyzed by RNA enzymes in extracellular environments (16,17). Recently, nanoparticle-based drugs have become notable trends in the research and development of CD medications, including multi-responsive nanoparticle-mediated delivery and naturally-derived targeting vesicles (18). Benefitted by their stability and target cell adsorption, exosomes do not need to induce host immune responses when used as drug vectors, further highlighting their potential for clinical treatment (19,20).
MicroRNA 21 (miR-21) plays a crucial role in many biological functions and diseases including development, cancer, cardiovascular diseases and inflammation (21). Uncontrolled miR-21 has been found in almost all types of cancers and therefore was classified as an oncomiR (22). During recent years, additional roles of miR-21 in cardiomyopathies and pulmonary fibrosis have been described (23,24). MiR-21 additionally regulates various immunological and inflammatory processes (25,26). Due to the critical functions of its target proteins in various signaling pathways, miR-21 has become an attractive target for genetic and pharmacological modulation in various disease conditions (21). The endothelial vascular system is one of the largest and most important tissues in the human body, and so it follows that there must be a connection between abnormal hyperplasia of blood vessels and endothelial dysfunction in patients with CD. Our study found that levels of plasma exosomal miR-21 in patients with CD were significantly increased and positively correlated with the severity of the disease. We verified that miR-21 targeted phosphatase and tensin homolog (PTEN) to weaken the obstruction of the phosphoinositide 3-kinase (PI3K)/AKT serine/threonine kinase (AKT) pathway of PTEN. Plasma exosomal miR-21 induced the migration and proliferation of human umbilical vein endothelial cells (HUVECs), which may be an important mechanism leading to CD-related angiogenesis and vascular dysfunctions. Therefore, exosomal miR-21 may be a new biomarker for evaluating the progression and prognosis of CD. Targeting miR-21 in combination with existing conventional treatments may be a new therapeutic strategy for the treatment or prevention of vascular disease in CD. We present the following article in accordance with the MDAR reporting checklist (available at https://atm.amegroups.com/article/view/10.21037/atm-22-475/rc).
Methods
Human blood and colon tissues
Blood samples were collected from the median cubital vein of patients with CD and healthy control subjects. Colon tissues were taken from patients during CD surgery, while control samples were taken from individuals without lesions and malignancies. All patients were diagnosed by symptoms and intestinal pathology at The Third Xiangya Hospital of Central South University (Changsha, Hunan, China). All procedures performed in this study involving human participants were in accordance with the Declaration of Helsinki (as revised in 2013). The study was approved by Ethics Committee on Scientific Research of The Third Xiangya Hospital of Central South University (No. 2020-S319) and informed consent was taken from all the patients.
Purification and quality control of exosomes
Exosomes were isolated by gradient centrifugation and filtration (16). In brief, the supernatants were centrifuged at 2,000 ×g for 20 min and 12,000 ×g for 70 min, filtered with a 0.22-µm filter, and ultracentrifuged at 200,000 ×g for 120 min at 4 ℃ using an Optima L-80 XP machine (Beckman Coulter Inc., Brea, CA, USA). The exosome pellets were resuspended in sterile phosphate-buffered saline (PBS), filtered using a 0.22-µm filter, and stored at −80 ℃. The morphology of the exosomes was observed by transmission electron microscopy (TEM; HT7800, Hitachi, Tokyo, Japan). The particle sizes were detected by nanoparticle tracking analysis (NTA; ZetaView PMX 110, Particle Metrix, Meerbusch, Germany). Exosomal markers, including calnexin, heat shock protein 70 (HSP70), tumor susceptibility gene 101 (TSG101), and cell growth-inhibiting gene 2 protein (CD9), were analyzed using western blotting.
Cell culture
HUVEC is a kind of model cell commonly used in vascular endothelial experiments, which can theoretically proliferate indefinitely and is easy to operate. HUVECs (4201HUM-CCTCC00635) were obtained from the Cell Resource Center of Peking Union Medical College, which is the headquarters of the National Infrastructure of Cell Line Resource (NSTI). The cell line was confirmed to be free of mycoplasma contamination by polymerase chain reaction (PCR) and culture. Its species origin was confirmed by PCR. The identity of the cell line was authenticated with short tandem repeat (STR) profiling (CODIS, FBI, Washington, DC, USA). All the results can be viewed on the National Experimental Cell Resource Sharing Platform website (http://cellresource.cn). HUVECs were cultured in Dulbecco’s Modified Eagle Medium (DMEM) F12 (HyClone) medium with 10% fetal bovine serum (Gibco, Life Technologies, Carlsbad, CA, USA) and 1% penicillin/streptomycin (Sangon Biotech, Shanghai, China).
Exosomal incubation
The concentration of exosomes was determined by the bicinchoninic acid (BCA) method, and 100 µg/mL of exosomes was added to the culture medium with 10% exosome-free fetal bovine serum (Gibco) and 1% penicillin/streptomycin (Sangon Biotech).
RNA transfection
miR-21 mimics and mimic controls were chemically synthesized mature double-stranded miRNAs, and miR-21 inhibitors and inhibitor controls were chemically modified complementary single-chain mature miRNAs. Fluorescent RNAs were flagged by Cy3 dye. All RNAs were designed and synthesized by RiboBio (Guangzhou, Guangdong, China). HUVECs were transfected with a riboFECTTMCP Transfection Kit (RiboBio), and the final concentrations of RNAs were 50 nM (mimics and mimic controls) and 100 nM (inhibitors and inhibitor controls).
miRNA and mRNA isolation and qPCR
The purification of miRNAs from exosomes was performed using a miRNeasy Mini Kit (Qiagen, Germantown, Tennessee, USA), while mRNAs were purified from cells using a Total RNA Kit II (Omega Bio-Tek Inc., Norcross, GA, USA). RNA was dissolved in diethyl pyrocarbonate (DEPC) water (Sangon Biotech). miRNA reverse transcription was performed using a miRNA 1st Strand cDNA Synthesis Kit (by stem-loop; Vazyme, Nanjing, China), after which quantitative PCR (qPCR) was implemented with a Light Cycler 480 II system (Roche, Basel, Switzerland) and a miRNA Universal SYBR qPCR Master Mix (Vazyme) using appropriate primers (Figure S1). mRNA reverse transcription was performed using a HiScript III All-in-one RT SuperMix Perfect for qPCR (Vazyme), after which qPCR was implemented using a Light Cycler 480 II system (Roche) and a ChamQ Universal SYBR qPCR Master Mix (Vazyme) with appropriate primers (Figure S1).
Wound healing assay
Wound healing assay and transwell migration assay were used to evaluate cell migration capacity. For the wound healing assay, cells were seeded in 6-well plates at 1.5×105 cells per well after being treated with the relevant reagents. Upon reaching approximately 90% confluence, the cells monolayers were scratched by the tip of a 200 µL pipette, washed with PBS twice gently, and cultured in serum-free medium. The images were captured using a white light microscope at 0, 24, 48, 72, and 96 h with at least 3 fields per well. The absolute migration area (area of initial area − area of final area) was calculated using ImageJ software (27).
Transwell migration assay
For the transwell migration assay, 8 µm Transwell-24 chambers (Corning Inc., Corning, NY, USA) were used. A serum-free medium was briefly added to the lower chamber. Approximately 1×105 cells/well were seeded in the upper chamber and were cultured with a serum-free medium at 37 ℃, 5% CO2 for 8 h. Then, cells adhering to the lower chamber were fixed with 4% paraformaldehyde Fixative Solution (Biosharp, Beijing, China) for 60 min and stained with 1% crystal violet solution (G-CLONE, Beijing, China) for 30 min. Five randomly selected fields were counted for each filter.
Tube formation assay
The Matrigel basement membrane matrix (Corning, 365231) was added to a 96-well plate on ice at 100 µL/well, then the 96-well plates were incubated at 37 ℃, 5% CO2 for 1 hour to cure the Matrigel. HUVECs were transferred to the 96-well plates at a density of 3×104 cells in the medium, and tube formation was observed after 2, 4, and 8 h. Data analysis of tube formation was performed using the Angiogenesis Analyzer plugin of ImageJ.
Western blotting
Western blotting was conducted using fresh cell lysates, which were separated via 10% sodium dodecyl sulphate–polyacrylamide gel electrophoresis (SDS-PAGE) and transferred to polyvinylidene difluoride (PVDF) membranes (Millipore Sigma, St. Louis, MOUSA) that were then blocked for 1 to 2 h with 1% bovine serum albumin (BSA). The blots were probed overnight with appropriate primary antibodies at 4 ℃, followed by probing for 1 h with secondary horseradish peroxidase (HRP)-conjugated antibodies. Protein bands were then detected with an enhanced chemiluminescence (ECL) kit (Biosharp). The primary antibodies used were as follows: anti-calnexin (2679, Cell Signaling Technology, Danvers, MA, USA), anti-HSP70 (4872, Cell Signaling Technology), anti-TSG101 (sc-7964, Santa Cruz Biotechnology, Santa Cruz, CA, USA), anti-CD9 (ab92726, Abcam, Cambridge, UK), anti-PTEN (9552, Cell Signaling Technology), anti-PI3K (4249, Cell Signaling Technology), anti-AKT (9272, Cell Signaling Technology), anti-P-AKT (4060, Cell Signaling Technology), and anti-GAPDH (10494-1-AP, Proteintech, Wuhan, China). Goat anti-rabbit IgG secondary antibody (HRP; Proteintech) and goat anti-mouse IgG (HRP; Proteintech) were used.
Statistical analysis
All statistical analyses were performed using IBM SPSS Statistics 22.0 (IBM Corp., Armonk, NY, USA). GraphPad Prism 8.0 software (GraphPad Prism Software, Inc., San Diego, CA, USA) was used to create graphs. Data are expressed as mean ± standard deviation (SD). One-way analysis of variance was used for different indicators between groups. For 2 sets of mean comparisons, a t-test was used for 2 independent groups that showed a normal distribution. The ratio comparison between 2 groups was analyzed by a chi-squared test, and the ratio comparison among 3 groups was analyzed by a 2×2 contingency table chi-squared test.
Results
Quality control of exosomes and higher enrichment of exosomal miR-21 in CD
Transmission electron microscopy showed that the purified vesicles had a saucer-like structure with a clear membrane and a diameter of approximately 100 nm (Figure 1A). The particle size analysis further confirmed that the diameter of the extracted vesicles was 90–120 nm (Figure 1B). Western blot analysis indicated that the exosomes were positive for the HSP70, TSG101, and CD9 markers and negative for calnexin (Figure 1C). The plasma exosomal miR-21 levels in patients with active CD were higher than those in patients with stable CD and in healthy subjects (Figure 1D).
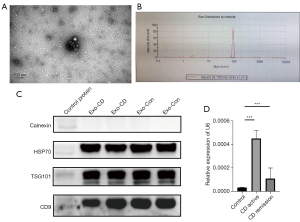
Exosomes derived from CD and exosomal miR-21 promote umbilical vein endothelial migration and tubulogenesis through activation of the PI3K/ATK pathway
We incubated PKH67-labeled exosomes with HUVECs under dark conditions, and the exosomes were primarily observed to enter the cells within 30 min (Figure S1A). We transfected HUVECs with miR-21 mimics and inhibitors and adjusted the transfection conditions to ensure high-efficiency transfection (determined by CY3 fluorescence under an inverted fluorescence microscope; Figure S1B). Wound healing assay and transwell invasion test showed that HUVECs transfected with miR-21 mimic had more powerful migration abilities than those of the control groups (P<0.01; Figure 2A-2D) (Figure 2A, the color difference in images of 48 h could be machine errors that caused by the aperture adjustment of the microscope). We also performed a tube formation assay and found that miR-21 could markedly enhance the tubule-forming capacity of HUVECs when compared with mimic controls (P<0.01; Figure 2E,2F). Western blotting results showed that the PI3K/AKT pathway was activated in HUVEC transfected with miR-21, and the expression of hypoxia-inducible factor 1-alpha (HIF-1α) and vascular endothelial growth factor (VEGF) was significantly increased (P<0.01; Figure 2G,2H). Through bioinformatic analysis (Figure S1C), we found that miR-21 could target and bind to PTEN 3’ untranslated region (3’UTR), which affected its protein expression level but did not interfere with its mRNA level (P<0.01; Figure 2I,2J).
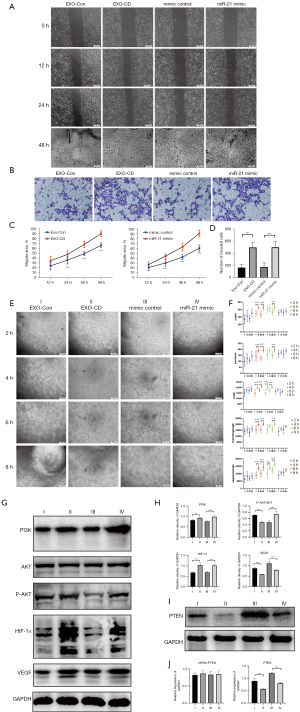
miR-21 inhibitors can effectively antagonize PI3K/AKT pathway activation and endothelial migration tubulogenesis induced by plasma exosomes of patients with CD in vitro
To further explore the mechanism by which the plasma exosomal miR-21 of patients with CD promotes migration and tubulogenesis of endothelial cells, we transfected miR-21 inhibitors into HUVECs that had been incubated in advance by CD-derived plasma exosomes. Wound healing assay, transwell invasion test and tube formation assay were also performed, and the results showed that miR-21 inhibitors significantly blocked the quick migration and tubule formation of HUVECs induced by CD exosomes (P<0.01; Figure 3A-3F). Western blotting results showed that miR-21 inhibitors can effectively antagonize the PI3K/AKT pathway activated by CD exosomes, and the expression of HIF-1α and VEGF in miR-21 inhibitor group was significantly decreased compared with that in the exosomes-CD-only group and the normal group (P<0.01; Figure 3G,3H).
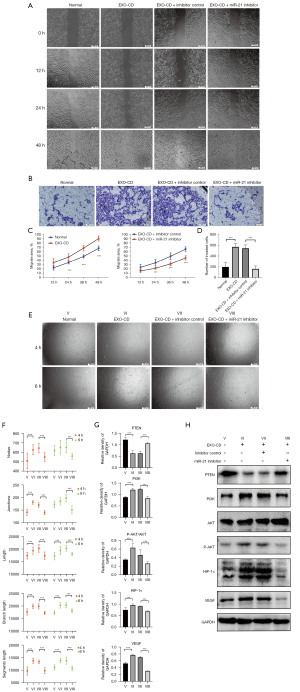
Discussion
Exosome research on CD has been advancing for some time. Exosomal miRNAs play an important role in regulating the function of the intestinal barrier by inhibiting the growth and function of intestinal intrinsic layer T cells or DC cells, activating differentiation factors, and driving nuclear factor kappa B (NF-κB) signaling pathways in macrophages (28-32). However, few studies have investigated the mechanism of vascular changes in CD. A recent study (33) on atherosclerosis showed that exosomes derived from the vascular smooth muscle cells can enter into an endothelial cell to destroy its integrity. Moreover, the highly expressed miRNA in the exosomes can improve the function of the epithelial barrier, suggesting that exosomes can enter endothelial cells by endocytosis and can release miRNAs to participate in the biological function of vascular endothelial cells.
Our research found miR-21 enrichment in plasma exosomes derived from patients with CD, and this exosomal miR-21 could stimulate the migration and tubule formation of HUVECs with a high expression of AKT phosphorylation. Therefore, we speculated that the plasma exosomal miR-21 of patients with CD may promote vascular pathological proliferation by regulating AKT phosphorylation. Studies have found that exosomal miR-21 can target the PTEN gene of chromosome 10 to activate the PI3K/AKT signaling pathway in malignant neoplasms, while others have used dual luciferase reporter gene assay to verify the specific binding of miR-21 to the 3’UTR of PTEN (34-36). However, there have been no previous reports on the role of exosomal miR-21 in CD. Overactivated PI3K/AKT can raise hypoxia-inducible factor 1-alpha (HIF-1α) to promote the expression and secretion of VEGF and induce vascular regeneration, while PTEN can inhibit the above effect by suppressing the PI3K/AKT signaling pathway. Therefore, we propose the following hypothesis: plasma exosomal miR-21 enters the vascular endothelial cells to inhibit PTEN gene transcription, activates the PI3K/AKT signaling pathway to raise HIF-1α, promotes the expression and secretion of VEGF, and induces vascular neogenesis.
PTEN, a newly identified tumor suppressor gene, regulates intracellular phosphor phospholipase levels by negatively regulating the AKT/PKB signaling pathway, which inhibits cell growth. PTEN was once considered to be a part of the PI3K/AKT/mammalian target of rapamycin (mTOR) pathway, but mTOR inhibitors are not effective in the treatment of patients with PTEN deficiency (30). Therefore, the ability of miRNA to target PTEN has attracted the attention of many researchers. Multiple studies have shown that exosomal miR-21 can regulate the proliferation of multiple cells (33,37,38). In our study, we simulated the process of miRNA delivery through the lipid shell of exosomes and transferred miR-21 mimics and inhibitors into HUVECs for observation. Wound scratch, transwell migration, and tubule formation experiments were performed. The results confirmed that exosomal miR-21 could significantly promote the migration and tubule formation ability of HUVECs, and miR-21 inhibitors could effectively counteract this migration, preventing the tubulogenic effect of CD-exosomes. In HUVECs transfected with CD-exosomes or miR-21, HIF-1α levels similarly increased when the PI3K/AKT pathway was activated, promoting the expression of VEGF. In HUVECs transfected with both CD-exosomes and miR-21 inhibitors, miR-21 inhibitors could also effectively counteract the PTEN/PI3K/HIF-1α axis. Thus, we clarified exosomal miR-21 mimic’s relationship with PTEN and further demonstrated its regulation of HIF-1α and VEGF downstream of the pathway. The activation of the PI3K/AKT signaling pathway may be a key player in the proliferation and migration of endothelial cells in CD, and this activation is induced by the targeted inhibition of PTEN by exosomal miR-21.
Conclusions
As demonstrated by the circulating exosomal miR-21 in CD, the regulation of the PTEN/PI3K/AKT signaling pathway by exosomal miR-21 enhances vascular endothelial proliferation and migration, thereby facilitating angiogenesis.
Acknowledgments
We thank the Medical Experiment Center of The Third Xiangya Hospital of Central South University for providing a scientific research platform.
Funding: This work was supported by the Changsha Municipal Natural Science Foundation (No. kq2014257), the National Natural Science Foundation of China (No. 81570509), the Science and Technology Program of Changsha (No. kq1701091), the Science and Technology Program of Hunan Province (No. 2018JJ6136), and the Scientific Research Project of Hunan Provincial Health Commission (No. 202103031034).
Footnote
Reporting Checklist: The authors have completed the MDAR reporting checklist. Available at https://atm.amegroups.com/article/view/10.21037/atm-22-475/rc
Data Sharing Statement: Available at https://atm.amegroups.com/article/view/10.21037/atm-22-475/dss
Conflicts of Interest: All authors have completed the ICMJE uniform disclosure form (available at https://atm.amegroups.com/article/view/10.21037/atm-22-475/coif). All authors report fundings from the Changsha Municipal Natural Science Foundation (No. kq2014257), the National Natural Science Foundation of China (No. 81570509), the Science and Technology Program of Changsha (No. kq1701091), the Science and Technology Program of Hunan Province (No. 2018JJ6136), and the Scientific Research Project of Hunan Provincial Health Commission (No. 202103031034). The authors have no other conflicts of interest to declare.
Ethical Statement: The authors are accountable for all aspects of the work in ensuring that questions related to the accuracy or integrity of any part of the work are appropriately investigated and resolved. All procedures performed in this study involving human participants were in accordance with the Declaration of Helsinki (as revised in 2013). The study was approved by Ethics Committee on Scientific Research of The Third Xiangya Hospital of Central South University (No. 2020-S319) and informed consent was taken from all the patients.
Open Access Statement: This is an Open Access article distributed in accordance with the Creative Commons Attribution-NonCommercial-NoDerivs 4.0 International License (CC BY-NC-ND 4.0), which permits the non-commercial replication and distribution of the article with the strict proviso that no changes or edits are made and the original work is properly cited (including links to both the formal publication through the relevant DOI and the license). See: https://creativecommons.org/licenses/by-nc-nd/4.0/.
References
- Bayoumy AB, de Boer NKH, Mulder CJJ. Management of Crohn Disease. JAMA 2021;325:1793-4. [Crossref] [PubMed]
- Wakefield AJ, Sawyerr AM, Dhillon AP, et al. Pathogenesis of Crohn's disease: multifocal gastrointestinal infarction. Lancet 1989;2:1057-62. [Crossref] [PubMed]
- Alkim C, Savas B, Ensari A, et al. Expression of p53, VEGF, microvessel density, and cyclin-D1 in noncancerous tissue of inflammatory bowel disease. Dig Dis Sci 2009;54:1979-84. [Crossref] [PubMed]
- Taylor SA, Punwani S, Rodriguez-Justo M, et al. Mural Crohn disease: correlation of dynamic contrast-enhanced MR imaging findings with angiogenesis and inflammation at histologic examination--pilot study. Radiology 2009;251:369-79. [Crossref] [PubMed]
- Saevik F, Nylund K, Hausken T, et al. Bowel perfusion measured with dynamic contrast-enhanced ultrasound predicts treatment outcome in patients with Crohn's disease. Inflamm Bowel Dis 2014;20:2029-37. [Crossref] [PubMed]
- Danese S, Fiorino G, Angelucci E, et al. Narrow-band imaging endoscopy to assess mucosal angiogenesis in inflammatory bowel disease: a pilot study. World J Gastroenterol 2010;16:2396-400. [Crossref] [PubMed]
- Principi M, Mastrolonardo M, Scicchitano P, et al. Endothelial function and cardiovascular risk in active inflammatory bowel diseases. J Crohns Colitis 2013;7:e427-33. [Crossref] [PubMed]
- Stordiau GE, Alecha JS, Goñi S, et al. Arterial thromboembolism from aortic floating thrombus in a patient with Crohn's Disease. J Crohns Colitis 2011;5:169-70. [Crossref] [PubMed]
- Maconi G, Bolzacchini E, Dell'Era A, et al. Portal vein thrombosis in inflammatory bowel diseases: a single-center case series. J Crohns Colitis 2012;6:362-7. [Crossref] [PubMed]
- Tian T, Zhu YL, Hu FH, et al. Dynamics of exosome internalization and trafficking. J Cell Physiol 2013;228:1487-95. [Crossref] [PubMed]
- Keerthikumar S, Chisanga D, Ariyaratne D, et al. ExoCarta: A Web-Based Compendium of Exosomal Cargo. J Mol Biol 2016;428:688-92. [Crossref] [PubMed]
- Saunderson SC, Dunn AC, Crocker PR, et al. CD169 mediates the capture of exosomes in spleen and lymph node. Blood 2014;123:208-16. [Crossref] [PubMed]
- Zhang J, Li S, Li L, et al. Exosome and exosomal microRNA: trafficking, sorting, and function. Genomics Proteomics Bioinformatics 2015;13:17-24. [Crossref] [PubMed]
- Chapman CG, Pekow J. The emerging role of miRNAs in inflammatory bowel disease: a review. Therap Adv Gastroenterol 2015;8:4-22. [Crossref] [PubMed]
- Kalla R, Ventham NT, Kennedy NA, et al. MicroRNAs: new players in IBD. Gut 2015;64:504-17. [Crossref] [PubMed]
- Lai RC, Yeo RW, Tan KH, et al. Exosomes for drug delivery - a novel application for the mesenchymal stem cell. Biotechnol Adv 2013;31:543-51. [Crossref] [PubMed]
- Azmi AS, Bao B, Sarkar FH. Exosomes in cancer development, metastasis, and drug resistance: a comprehensive review. Cancer Metastasis Rev 2013;32:623-42. [Crossref] [PubMed]
- Yang C, Merlin D. Nanoparticle-Mediated Drug Delivery Systems For The Treatment Of IBD: Current Perspectives. Int J Nanomedicine 2019;14:8875-89. [Crossref] [PubMed]
- Zhang Y, Xiao Y, Sun G, et al. Harnessing the therapeutic potential of extracellular vesicles for cancer treatment. Semin Cancer Biol 2021;74:92-104. [Crossref] [PubMed]
- Buschmann D, Mussack V, Byrd JB. Separation, characterization, and standardization of extracellular vesicles for drug delivery applications. Adv Drug Deliv Rev 2021;174:348-68. [Crossref] [PubMed]
- Kumarswamy R, Volkmann I, Thum T. Regulation and function of miRNA-21 in health and disease. RNA Biol 2011;8:706-13. [Crossref] [PubMed]
- Tse J, Pierce T, Carli ALE, et al. Onco-miR-21 Promotes Stat3-Dependent Gastric Cancer Progression. Cancers (Basel) 2022;14:264. [Crossref] [PubMed]
- Surina S, Fontanella RA, Scisciola L, et al. miR-21 in Human Cardiomyopathies. Front Cardiovasc Med 2021;8:767064. [Crossref] [PubMed]
- Wang J, He F, Chen L, et al. Resveratrol inhibits pulmonary fibrosis by regulating miR-21 through MAPK/AP-1 pathways. Biomed Pharmacother 2018;105:37-44. [Crossref] [PubMed]
- Zhang L, Yu L, Liu Y, et al. miR-21-5p promotes cell proliferation by targeting BCL11B in Thp-1 cells. Oncol Lett 2021;21:119. [Crossref] [PubMed]
- Xue J, Liu J, Xu B, et al. miR-21-5p inhibits inflammation injuries in LPS-treated H9c2 cells by regulating PDCD4. Am J Transl Res 2021;13:11450-60. [PubMed]
- Schneider CA, Rasband WS, Eliceiri KW. NIH Image to ImageJ: 25 years of image analysis. Nat Methods 2012;9:671-5. [Crossref] [PubMed]
- Alexander M, Hu R, Runtsch MC, et al. Exosome-delivered microRNAs modulate the inflammatory response to endotoxin. Nat Commun 2015;6:7321. [Crossref] [PubMed]
- Lippai D, Bala S, Catalano D, et al. Micro-RNA-155 deficiency prevents alcohol-induced serum endotoxin increase and small bowel inflammation in mice. Alcohol Clin Exp Res 2014;38:2217-24. [Crossref] [PubMed]
- Krissansen GW, Yang Y, McQueen FM, et al. Overexpression of miR-595 and miR-1246 in the sera of patients with active forms of inflammatory bowel disease. Inflamm Bowel Dis 2015;21:520-30. [Crossref] [PubMed]
- Cooks T, Pateras IS, Jenkins LM, et al. Mutant p53 cancers reprogram macrophages to tumor supporting macrophages via exosomal miR-1246. Nat Commun 2018;9:771. [Crossref] [PubMed]
- Tian T, Zhou Y, Feng X, et al. MicroRNA-16 is putatively involved in the NF-kappaB pathway regulation in ulcerative colitis through adenosine A2a receptor (A2aAR) mRNA targeting. Sci Rep. 2016;6:30824. [Crossref] [PubMed]
- Zhu J, Liu B, Wang Z, et al. Exosomes from nicotine-stimulated macrophages accelerate atherosclerosis through miR-21-3p/PTEN-mediated VSMC migration and proliferation. Theranostics 2019;9:6901-19. [Crossref] [PubMed]
- Haddadi N, Lin Y, Travis G, et al. PTEN/PTENP1: 'Regulating the regulator of RTK-dependent PI3K/Akt signalling', new targets for cancer therapy. Mol Cancer 2018;17:37. [Crossref] [PubMed]
- Chen T, Yu Q, Xin L, et al. Circular RNA circC3P1 restrains kidney cancer cell activity by regulating miR-21/PTEN axis and inactivating PI3K/AKT and NF- kB pathways. J Cell Physiol 2020;235:4001-10. [Crossref] [PubMed]
- Li X, Dai Y, Xu J. MiR-21 promotes pterygium cell proliferation through the PTEN/AKT pathway. Mol Vis 2018;24:485-94. [PubMed]
- Cao LQ, Yang XW, Chen YB, et al. Exosomal miR-21 regulates the TETs/PTENp1/PTEN pathway to promote hepatocellular carcinoma growth. Mol Cancer 2019;18:148. [Crossref] [PubMed]
- Terren C, Nisolle M, Munaut C. Pharmacological inhibition of the PI3K/PTEN/Akt and mTOR signalling pathways limits follicle activation induced by ovarian cryopreservation and in vitro culture. J Ovarian Res 2021;14:95. [Crossref] [PubMed]
(English Language Editor: C. Gourlay)