IKKα contributes to ischemia-induced autophagy after acute cerebral ischemic injury
Introduction
Stroke is a leading cause of mortality and disability worldwide, placing significant burden on the social healthcare system (1). Tissue-type plasminogen activator (tPA) is currently the only medical treatment for acute ischemic strokes approved by the American Food and Drug Administration. However, less than 5% of ischemic patients benefit from tPA therapy due to its narrow therapeutic time window and potential severe adverse events including brain edema and the risk of hemorrhagic transformation (2,3). Therefore, novel therapeutic targets for the treatment of ischemic injury and its related mechanisms are urgently being investigated.
The IκB kinase (IKK) complex contains two catalytic subunits, IKKα and IKKβ, that play important roles in triggering nuclear factor (NF)-κB activation under physiological and pathological conditions. While IKKα and IKKβ share partial structural similarities, they activate NF-κB through different mechanisms (4). Notably, both IKKα and IKKβ exert crucial NF-κB-independent functions through other substrates, such as c-Fos, p85α, 14-3-3δ, silencing mediator for retinoid or thyroid-hormone receptors (SMRT), mammalian target of rapamycin (mTOR), steroid receptor coactivator 3 (SRC3), and maspin (4-7). Together, these studies suggest that both IKKα and IKKβ may act as multifunctional proteins that trigger a series of NF-κB-independent signaling events beyond their well-known functions in the NF-κB pathway. A previous study has shown that activation of IKKβ enhances the ischemia infarct volume at 48 hours after the onset of middle cerebral artery occlusion (MCAO) (8). In transient focal cerebral ischemia, IKKα is involved in oxidative stress injury and microglial polarization after stroke (9,10). However, the role of IKKα in permanent cerebral ischemia remains unclear.
Autophagy is a highly conserved and complex catabolic process that maintains intracellular homeostasis through degradation of cytoplasmic components (macromolecules or damaged organelles) (11,12). Autophagy is generally induced by stress, such as nutrient starvation, oxygen and energy deprivation (13,14). Various indicators can be used to detect autophagic activity, such as the conversion of microtubule associated protein 1 light chain 3 (LC3) I to LC3 II, Beclin 1 upregulation, and SQSTM1/p62 degradation. Among these proteins, LC3 II turnover is the most widely used maker for autophagosomes (14,15). The role of autophagy after cerebral ischemia is still controversial (16). It is reported that autophagy activation alleviated global ischemia induced neuronal death (17). In cultured cortical neurons, autophagy inducer rapamycin protected against neuronal damage after oxygen-glucose deprivation (OGD) treatment (18). However, inhibition of overactivated autophagy by Sodium hydrosulfide (NaHS) was reported to attenuate cerebral ischemia/reperfusion injury (19). In our study, we proposed that autophagy activation contributed to permanent cerebral ischemia injury.
In transient cerebral ischemic-reperfusion, autophagy dependent IKKα degradation is involved in microglial polarization (10). However, the relationship between IKKα and autophagy is unclear after permanent cerebral ischemia. This current study investigated the potential role of IKKα in mediating cerebral ischemia injury via regulating autophagy in a cellular oxygen-glucose deprivation/reperfusion (OGD/R) model and a permanent cerebral ischemic mice model. We present the following article in accordance with the ARRIVE reporting checklist (available at https://atm.amegroups.com/article/view/10.21037/atm-22-517/rc).
Methods
Animal models and experimental groups
All experimental procedures were performed under a project license (No. 201902039) by the Animal Welfare Ethics Committee of Beijing Neurosurgical Institute and were performed in accordance with the guidelines of the National Institutes of Health on the care and use of laboratory animals. Male C57/BL6 mice were housed individually in polycarbonate cages in a regular 12-hour light-dark cycle at 22±2 °C.
Distal MCAO (dMCAO) was performed by electrocoagulation of the distal middle cerebral artery (MCA) as previously reported (20,21). To confirm the ischemia in mice, regional cerebral blood flow (rCBF) was detected with a laser Doppler flowmetry (RWD Life Science, Shenzhen, China) before and after dMCAO. The body temperature of the mice was maintained at normothermia during surgery with a heating pad (RWD Life Science).
A total of 60 mice (age of 8 to 10 weeks) weighed 24±0.5 g were used in this study. The mice were randomly assigned into four groups, namely, sham (n=10), dMCAO (n=10), dMCAO + control small interfering RNA (control siRNA; n=21), dMCAO + IKKα siRNA (n=19). Surgery, siRNA preparation and administration, outcome assessment, and data analyses were performed by researchers who were blinded to the experimental conditions of the mice. No mice died due to surgery or stroke complications. A protocol was prepared before the study without registration.
Cell culture and transfection
The mouse neuroblastoma cells neuro-2A (N2A) were grown in Dulbecco’s modified Eagle’s medium (DMEM) supplemented with 10% fetal bovine serum. Cells were transfected with IKKα siRNA (CCGGGAACUUGAUCUCAAA) or control siRNA (UUCUCCGAACGUGUCACGU, GenePharma, Suzhou, China) using Lipofectamine™ RNAi MAX (Invitrogen, Waltham, MA, USA) according to the manufacturer’s instructions.
OGD/R
To mimic ischemia in vitro, N2A cells were exposed to OGD for 1 hour, followed by reoxygenation for 24 hours. Briefly, the culture medium was replaced with glucose-free DMEM (Gibco, Grand Island, NY, USA) and cells were placed in a hypoxic chamber (Billups Rothenberg, San Diego, CA, USA) containing 5% CO2, 0.02% O2 and 94.98% N2 according to our previous study (22). After 1 hour of OGD, cells were maintained in original culture medium for 24 hours. Cells in the control group were maintained in normal growth medium under normoxic culture conditions.
Western blot assay
Total proteins were extracted from N2A cells with lysis buffer or from mice cortex as previously described (23). All protein blots were probed with primary antibodies against IKKα, IKKβ, microtubule associated protein 1 light chain 3β (LC3B), or actin (1:1,000, Cell Signaling Technology, Beverly, MA, USA). Protein expression was quantified and normalized to actin, the loading control.
Cyto-ID autophagy detection
To detect specific autophagic fluorescence signals in N2A cells, the Cyto-ID Autophagy Detection Kit was used according to the manufacturer’s instructions (Enzo Life Science, Farmingdale, New York, USA). Briefly, after treatment, N2A cells were carefully washed twice with assay buffer and incubated with Cyto-ID green detection reagent and 4',6-diamidino-2-phenylindole (DAPI) nuclear stain for 30 minutes at 37 °C. Cells were then washed twice with assay buffer. The stained cells were observed by confocal microscopy (Zeiss, Oberkochen, Germany) using a FITC filter (autophagic signal) and a DAPI filter.
Intracerebroventricular (i.c.v) injection
IKKα siRNA or control (Con) siRNA (5.6 µL) mixed with the transfection reagent (1.4 µL, Invitrogen) was delivered into the right ipsilateral ventricle (1.0 mm lateral and 0.5 mm posterior to bregma, 2.5 mm dorsoventral below the skull) according to the stereotaxic map in the mice 1 hour before dMCAO onset. The injection was administrated at a rate of 0.5 µL/min and the needle was retained for another 15 minutes after injection. The bone wound was sealed with bone wax.
Immunofluorescence staining
Mice brain sections (20 µm) were stained with rabbit anti-LC3 (autophagy marker) and DAPI (a nuclear specific marker) and observed with a confocal microscope (Zeiss).
2,3,5-triphenyltetrazolium chloride (TTC) staining
Mice were deeply anesthetized using isoflurane at 24 hours after dMCAO. The brains were removed quickly and cut into seven 1-mm thick slices. The infarct volume was measured by TTC staining as described previously (20).
Magnetic resonance imaging (MRI)
MRI was conducted using a 7.0 T BioClinScan animal system (Bruker, Germany) to assess the extent of brain infarction and water diffusion. The T2 weighted (T2W) sequence consisted of the following parameters: time to repetition/echo time (TR/TE) =3,080 ms/41 ms, field of view (FOV) =24 mm × 30 mm, and slice thickness =0.5 mm. The diffusion weighted sequence was performed using the following parameters: TR/TE =4,500 ms/46 ms, FOV =25 mm × 30 mm, b value =600 s/mm2, number of slices =28. Mice were anesthetized using isoflurane (1.5%) during the MRI experiments at 24 hours after dMCAO. The infarct areas after dMCAO showed high intensity signals on T2W images (24). The infarct volume was calculated based on the T2W images using ImageJ software (National Institutes of Health, USA). The apparent diffusion coefficient (ADC) estimates the water diffusion in the brain (25). The relative ADC (%) was calculated by the ADC values in the peri-infarct area divided by the corresponding area in the contralateral brain.
Modified Garcia score test
The sensorimotor functions in the mice were assessed using the modified Garcia test at 24 hours after dMCAO as previously described (20,26). Briefly, five tests were conducted, including body proprioception, vibrissae touch, limb symmetry, lateral turning, and forelimb walking. For each test, the scores ranged from 0–3.
Terminal deoxynucleotidyl transferase (TdT)-mediated dUTP nick end labeling (TUNEL) staining
TUNEL staining was conducted using the In Situ Cell Death Detection Kit according to the manufacturer’s instructions (Roche, South San Francisco, CA, USA). The nuclei of cells were stained with DAPI as previously described (22).
Statistical analysis
Data are presented as mean ± standard deviation (SD). All statistical analyses were conducted using GraphPad Prism 8.0 software (GraphPad Software Inc., La Jolla, CA, USA). Statistical differences were analyzed using one way analysis of variance (ANOVA) or unpaired t-tests. A P value <0.05 was considered statistically significant.
Results
IKKα played a critical role in regulating autophagy under OGD/R treatment
The expression of IKKα and the presence of autophagy after OGD/R were investigated in vitro. The protein expression of IKKα was significantly increased after OGD/R (Figure 1A,1B). However, the expression of IKKβ did not change under OGD/R treatment (Figure 1A,1C). Meanwhile, the ratio of the specific autophagic key protein LC3B II/I was significantly increased in OGD/R treated N2A cells (Figure 1A,1D). Autophagic activity assessed using the commercial Cyto-ID Autophagy Detection Kit showed significant accumulation of green autophagic fluorescence signals in the perinuclear region in OGD/R treated cells but not in control cells (Figure 1E), suggesting that autophagic activity increased after OGD/R treatment.
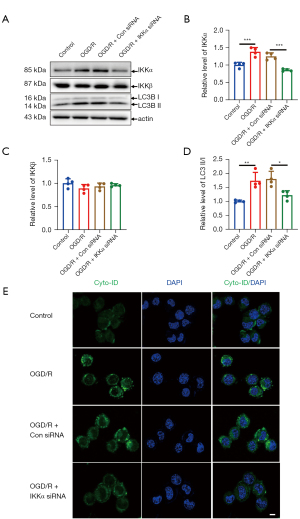
To explore whether IKKα was involved in OGD/R-induced autophagy in vitro, IKKα siRNA or control siRNA was transfected into N2A cells before OGD/R treatment. The LC3 II/I ratio was significantly decreased in OGD/R cells transfection with IKKα siRNA compared to the cells transfected with the control siRNA(Figure 1A,1D). Moreover, Cyto-ID autophagic fluorescence signals were also impaired after knockdown of IKKα expression (Figure 1E). Taken together, these data suggested that IKKα was essential for OGD/R-induced autophagy in vitro.
Inhibition of IKKα reduced infarct volume after dMCAO
To further clarify the effects of IKKα on acute stroke in vivo, IKKα siRNA or control siRNA were administered into the right ventricle of the mice (Figure 2A,2B). TTC staining at 24 hours after dMCAO (Figure 2C,2D) showed that the infarct volume in the IKKα siRNA group (infarct volume: 16.00±3.09 mm3) was significantly decreased compared with the control siRNA group (infarct volume: 24.33±5.34 mm3; P=0.0133). To further confirm the protective role of IKKα inhibition after dMCAO, T2W-MRI were performed to assess the infarct volume at 24 hours after dMCAO (Figure 2E). As shown in Figure 2F, the infarct size was significantly lower in IKKα siRNA-treated mice compared to control siRNA-treated ischemic mice. Moreover, increased relative ADC values in the peri-infarct area of ipsilateral cortex were detected in the IKKα siRNA-treated group (82.15%±1.93%) compared to the control siRNA-treated group (74.03%±3.24%; P=0.0013; Figure 2G). rCBF was monitored before and after dMCAO (Figure 2H). There was no significant difference in rCBF reduction at 24 h after ischemia between IKKα siRNA- and Con siRNA-treated groups (Figure 2I). These results suggested that inhibition of IKKα exerted a protective effect on cerebral brain damage after dMCAO.
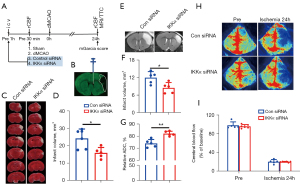
Inhibition of IKKα improved neurological functions after dMCAO
To investigate the effects of IKKα inhibition on neurological functions, sensorimotor deficits were assessed using the modified Garcia test scores at 24 hours after dMCAO. As shown in Figure 3A, vibrissae touch was significantly improved in IKKα siRNA-treated mice compared to control siRNA-treated mice. The IKKα siRNA treated group showed an improved trend with four other sensorimotor functions, including body proprioception, limb symmetry, lateral turning, and forelimb walking (Figure 3B-3E). All five assessments resulted in an increase in the total neurological score in IKKα siRNA-mice compared to control siRNA mice (Figure 3F). These data revealed that inhibition of IKKα improved some aspects of neurological outcomes at 24 hours after dMCAO.
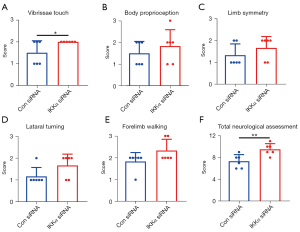
Inhibition of IKKα decreased cell death after dMCAO
The protective role of IKKα inhibition after dMCAO was further assessed using the TUNEL assay. The percentage of TUNEL positive cells in the dMCAO group (15.24%±3.65%) and the control siRNA group (17.66%±5.02%) were significantly increased compared with the sham group (2.11%±2.25%; P<0.001; Figure 4A,4B). Mice treated with IKKα siRNA (7.84%±5.16%) showed significantly decreased TUNEL positive cells compared to the control siRNA group (17.66%±5.02%; P<0.001; Figure 4A,4B). These results suggested that inhibition of IKKα reduced cell death after dMCAO.
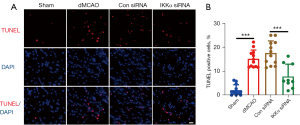
Inhibition of IKKα reduced autophagy activation after dMCAO
To verify the relationship between IKKα and autophagy in vivo, IKKα expression levels and autophagy activation were examined using tissue from the cerebral cortex of mice after dMCAO. As shown in Figure 5A,5B, the levels of IKKα were significantly increased in dMCAO mice and control siRNA treated mice. The ratio of LC3B-II/I also upregulated after dMCAO. The IKKα siRNA was injected into the ipsilateral ventricle of the mice at 1 hour before the onset of ischemia and the ischemia-induced IKKα expression was effectively blocked after dMCAO. Under the same conditions, the conversion of LC3 I/II was significantly reduced compared with that in the control siRNA group (Figure 5A,5C). The expression of IKKβ was not altered after dMCAO nor after IKKα siRNA treatment (Figure 5A,5D).
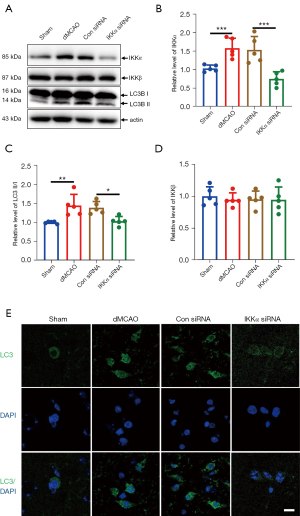
To further determine whether IKKα regulates the level of autophagy after cerebral ischemia, immunostaining of LC3 was assessed. Positive fluorescence (autophagosome) was induced in dMCAO and control siRNA mice. In contrast, LC3 positive signals were hardly seen in the IKKα siRNA group (Figure 5E). These results suggested that IKKα was involved in ischemia-induced autophagy after cerebral ischemia.
Discussion
The role of IKKα, one of the catalytic kinases of the IκB kinase complex, in cerebral ischemia is not fully understood. To the best of our knowledge, this is the first study to report ischemia-induced IKKα upregulation both in vitro, in N2A cells during 1 hour OGD/24 hours reoxygenation-induced ischemic injury, and in vivo, in the peri-infarct area of mice at 24 hours after permanent cerebral ischemia (Figures 1,5). Under the same conditions, the expression of the other catalytic kinase of IKK (IKKβ) did not change after ischemia. Furthermore, inhibition of IKKα provided neuroprotection against cerebral ischemia through decreasing LC3-mediated autophagy both in vitro and in vivo (Figures 2-5).
There is accumulating evidence that IKKα has crucial NF-κB-dependent and NF-κB-independent functions under various physiological and pathological conditions, such as inflammation, cellular stress, and aging (4,27,28). Our previous data showed that IKKα regulated vascular endothelial growth factor (VEGF) expression through a c-Fos-dependent, but not IKKβ/NF-κB-independent manner in UVB-induced photodamage (7). In addition, IKKα has been reported to independently inhibit the transcription of maspin, a metastasis suppressor, thereby mediating tumor metastasis. Moreover, IKKα knockdown decreased β-catenin expression without altering NF-κB activity and inhibited cell growth in multiple myeloma cells (4). These results revealed that IKKα may trigger a battery of NF-κB-independent downstream substrates. The role of IKKα in cerebral ischemia is not fully understood. After transient focal cerebral ischemia, IKKα activation resulted in phosphorylation of histone H3 in response to oxidative stress (9). IKKα is degraded via selective autophagy in primary microglia under OGD/R conditions (10). To improve the quality of preclinical studies, the Stroke Therapy Academic Industry Roundtable (STAIR) recommends that both transient and permanent occlusion models should be performed in the preclinical studies, especially permanent occlusion models should be adopted first (29). In the current study, IKKα, but not IKKβ, was significantly induced in N2A cells after OGD/R treatment and mice suffered permanent cerebral ischemia (Figures 1,5). Application of IKKα siRNA exerted a protective role against brain damage through reducing cerebral infarct volume, improving sensorimotor functions, and decreasing cell death (Figures 2-4).
The ADC measured by MRI reflects the degree of diffusion of water molecules (24). In pathological conditions, a decrease in ADC values is considered to be associated with the reduction of extracellular space caused by cellular swelling (30). As indicated in Figure 2G, knockdown of IKKα expression significantly increased the relative ADC in the peri-infarct area at 24 hours after dMCAO. This result demonstrated that IKKα may contribute to cell death through altered water mobility and related cytotoxic edema after acute cerebral ischemia. However, the relationship between IKKα and cell swelling warrants further investigation.
In response to nutrient deprivation, IKKα induces the expression of the essential pro-autophagic gene, Beclin-1. Moreover, loss of NF-κB does not affect starvation-induced expression of the autophagic gene (31). However, a previous study from phosphatase and tensin homolog deleted on chromosome ten (PTEN)-null prostate cancer cells showed that IKKα controlled mTOR activation, which in turn inhibited autophagy, with less involvement by IKKβ (4). Thus, the role of IKKα in regulating autophagy may vary in different settings.
The potential mechanisms by which IKKα mediates ischemia-induced LC3B expression is unclear at present. It is possible that IKKα directly binds with LC3B through the classical LC3-interacting region (LIR) motif (W/F/Y-X-X-V/L/I) (32). AMP-activated protein kinase (AMPK) is a vital sensor of ATP/ADP energy balance in cells and triggers autophagy when activated (33). Inhibiting the expression of IKK decreases the activation of AMPK and the conversion of LC3-I to LC3-II in intestinal epithelial cells. We observed AMPK phosphorylation in the peri-infarct area of mice with acute cerebral ischemia (data not shown). It is therefore possible that the association between IKKα and LC3B is mediated by AMPK and warrants further investigation.
Conclusions
In conclusion, this study clearly elucidated a novel role of IKKα in regulating the activation of autophagy after acute cerebral ischemia. These finding may assist in the development of novel therapeutic strategies for alleviating brain injury by targeting IKKα.
Acknowledgments
Funding: This work was supported by the National Natural Science Foundation of China (Nos. 81701138, 81871021, 82171268); the Beijing Hospitals Authority Youth Programme (No. QML20180505); the Beijing Excellent Talents (No. 2017000021469G249); and the National Science and Technology Major Project (No. 2017ZX09304018).
Footnote
Reporting Checklist: The authors have completed the ARRIVE reporting checklist. Available at https://atm.amegroups.com/article/view/10.21037/atm-22-517/rc
Data Sharing Statement: Available at https://atm.amegroups.com/article/view/10.21037/atm-22-517/dss
Conflicts of Interest: All authors have completed the ICMJE uniform disclosure form (available at https://atm.amegroups.com/article/view/10.21037/atm-22-517/coif). The authors have no conflicts of interest to declare.
Ethical Statement: The authors are accountable for all aspects of the work in ensuring that questions related to the accuracy or integrity of any part of the work are appropriately investigated and resolved. All experimental procedures were performed under a project license (No. 201902039) by the Animal Welfare Ethics Committee of Beijing Neurosurgical Institute and were performed in accordance with the guidelines of the National Institutes of Health on the care and use of laboratory animals.
Open Access Statement: This is an Open Access article distributed in accordance with the Creative Commons Attribution-NonCommercial-NoDerivs 4.0 International License (CC BY-NC-ND 4.0), which permits the non-commercial replication and distribution of the article with the strict proviso that no changes or edits are made and the original work is properly cited (including links to both the formal publication through the relevant DOI and the license). See: https://creativecommons.org/licenses/by-nc-nd/4.0/.
References
- Wang YJ, Li ZX, Gu HQ, et al. China Stroke Statistics 2019: A Report From the National Center for Healthcare Quality Management in Neurological Diseases, China National Clinical Research Center for Neurological Diseases, the Chinese Stroke Association, National Center for Chronic and Non-communicable Disease Control and Prevention, Chinese Center for Disease Control and Prevention and Institute for Global Neuroscience and Stroke Collaborations. Stroke Vasc Neurol 2020;5:211-39. [Crossref] [PubMed]
- Thiebaut AM, Gauberti M, Ali C, et al. The role of plasminogen activators in stroke treatment: fibrinolysis and beyond. Lancet Neurol 2018;17:1121-32. [Crossref] [PubMed]
- Hacke W, Kaste M, Bluhmki E, et al. Thrombolysis with alteplase 3 to 4.5 hours after acute ischemic stroke. N Engl J Med 2008;359:1317-29. [Crossref] [PubMed]
- Hinz M, Scheidereit C. The IκB kinase complex in NF-κB regulation and beyond. EMBO Rep 2014;15:46-61. [Crossref] [PubMed]
- Chariot A. The NF-kappaB-independent functions of IKK subunits in immunity and cancer. Trends Cell Biol 2009;19:404-13. [Crossref] [PubMed]
- Huang WC, Hung MC. Beyond NF-κB activation: nuclear functions of IκB kinase α. J Biomed Sci 2013;20:3. [Crossref] [PubMed]
- Dong W, Li Y, Gao M, et al. IKKα contributes to UVB-induced VEGF expression by regulating AP-1 transactivation. Nucleic Acids Res 2012;40:2940-55. [Crossref] [PubMed]
- Herrmann O, Baumann B, de Lorenzi R, et al. IKK mediates ischemia-induced neuronal death. Nat Med 2005;11:1322-9. [Crossref] [PubMed]
- Song YS, Kim MS, Kim HA, et al. Oxidative stress increases phosphorylation of IkappaB kinase-alpha by enhancing NF-kappaB-inducing kinase after transient focal cerebral ischemia. J Cereb Blood Flow Metab 2010;30:1265-74. [Crossref] [PubMed]
- Li X, Xia Q, Mao M, et al. Annexin-A1 SUMOylation regulates microglial polarization after cerebral ischemia by modulating IKKα stability via selective autophagy. Sci Adv 2021;7:eabc5539. [Crossref] [PubMed]
- Abounit K, Scarabelli TM, McCauley RB. Autophagy in mammalian cells. World J Biol Chem 2012;3:1-6. [Crossref] [PubMed]
- Yao RQ, Ren C, Xia ZF, et al. Organelle-specific autophagy in inflammatory diseases: a potential therapeutic target underlying the quality control of multiple organelles. Autophagy 2021;17:385-401. [Crossref] [PubMed]
- Li W, He P, Huang Y, et al. Selective autophagy of intracellular organelles: recent research advances. Theranostics 2021;11:222-56. [Crossref] [PubMed]
- Damme M, Suntio T, Saftig P, et al. Autophagy in neuronal cells: general principles and physiological and pathological functions. Acta Neuropathol 2015;129:337-62. [PubMed]
- Loos B, du Toit A, Hofmeyr JH. Defining and measuring autophagosome flux—concept and reality. Autophagy 2014;10:2087-96. [Crossref] [PubMed]
- Wang P, Shao BZ, Deng Z, et al. Autophagy in ischemic stroke. Prog Neurobiol 2018;163-164:98-117. [Crossref] [PubMed]
- Papadakis M, Hadley G, Xilouri M, et al. Tsc1 (hamartin) confers neuroprotection against ischemia by inducing autophagy. Nat Med 2013;19:351-7. [Crossref] [PubMed]
- Wang P, Guan YF, Du H, et al. Induction of autophagy contributes to the neuroprotection of nicotinamide phosphoribosyltransferase in cerebral ischemia. Autophagy 2012;8:77-87. [Crossref] [PubMed]
- Jiang WW, Huang BS, Han Y, et al. Sodium hydrosulfide attenuates cerebral ischemia/reperfusion injury by suppressing overactivated autophagy in rats. FEBS Open Bio 2017;7:1686-95. [Crossref] [PubMed]
- Dong W, Zhao S, Wen S, et al. A preclinical randomized controlled study of ischemia treated with Ginkgo biloba extracts: Are complex components beneficial for treating acute stroke? Curr Res Transl Med 2020;68:197-203. [Crossref] [PubMed]
- Liu X, Liu J, Zhao S, et al. Interleukin-4 Is Essential for Microglia/Macrophage M2 Polarization and Long-Term Recovery After Cerebral Ischemia. Stroke 2016;47:498-504. [Crossref] [PubMed]
- Liu X, Wen S, Zhao S, et al. Mild Therapeutic Hypothermia Protects the Brain from Ischemia/Reperfusion Injury through Upregulation of iASPP. Aging Dis 2018;9:401-11. [Crossref] [PubMed]
- Dong W, Qi Z, Liang J, et al. Reduction of zinc accumulation in mitochondria contributes to decreased cerebral ischemic injury by normobaric hyperoxia treatment in an experimental stroke model. Exp Neurol 2015;272:181-9. [Crossref] [PubMed]
- Xu R, Bai Y, Min S, et al. In vivo Monitoring and Assessment of Exogenous Mesenchymal Stem Cell-Derived Exosomes in Mice with Ischemic Stroke by Molecular Imaging. Int J Nanomedicine 2020;15:9011-23. [Crossref] [PubMed]
- Ohene Y, Harrison IF, Nahavandi P, et al. Non-invasive MRI of brain clearance pathways using multiple echo time arterial spin labelling: an aquaporin-4 study. Neuroimage 2019;188:515-23. [Crossref] [PubMed]
- Liu Z, Ran Y, Huang S, et al. Curcumin Protects against Ischemic Stroke by Titrating Microglia/Macrophage Polarization. Front Aging Neurosci 2017;9:233. [Crossref] [PubMed]
- Tilstra JS, Clauson CL, Niedernhofer LJ, et al. NF-κB in Aging and Disease. Aging Dis 2011;2:449-65. [PubMed]
- Antonia RJ, Hagan RS, Baldwin AS. Expanding the View of IKK: New Substrates and New Biology. Trends Cell Biol 2021;31:166-78. [Crossref] [PubMed]
- Fisher M, Feuerstein G, Howells DW, et al. Update of the stroke therapy academic industry roundtable preclinical recommendations. Stroke 2009;40:2244-50. [Crossref] [PubMed]
- Badaut J, Ashwal S, Adami A, et al. Brain water mobility decreases after astrocytic aquaporin-4 inhibition using RNA interference. J Cereb Blood Flow Metab 2011;31:819-31. [Crossref] [PubMed]
- Comb WC, Cogswell P, Sitcheran R, et al. IKK-dependent, NF-κB-independent control of autophagic gene expression. Oncogene 2011;30:1727-32. [Crossref] [PubMed]
- Petherick KJ, Williams AC, Lane JD, et al. Autolysosomal β-catenin degradation regulates Wnt-autophagy-p62 crosstalk. EMBO J 2013;32:1903-16. [Crossref] [PubMed]
- Li Y, Chen Y. AMPK and Autophagy. Adv Exp Med Biol 2019;1206:85-108. [Crossref] [PubMed]