GSK-3β activates NF-κB to aggravate caerulein-induced early acute pancreatitis in mice
Introduction
Acute pancreatitis, usually caused by gallstones and ethanol consumption (1), is an acute inflammatory disease of the exocrine pancreas, characterized by acute abdominal pain and a concomitant rise in serum amylase and lipase concentration (2). Although the majority of cases are mild and self-limited, the overall mortality of acute pancreatitis still remains at 4–8% (2), and can even reach 15% when patients with pancreatitis develop pancreatic or extrapancreatic necrosis and systemic inflammatory response syndrome (SIRS) (3). While the rate of hospitalization for acute pancreatitis has been increasing in the past decades (4), this disease lacks specific and effective interventions because of the little understanding of the early cellular events in the pathophysiology of the initiation of acute pancreatitis, posing a significant challenge to health providers worldwide.
Over the past century, it has been presumed that intra-acinar trypsinogen activation is the key event for the onset of pancreatitis (5,6). However, some researchers found that the progression of local and systemic inflammation could not be relieved when pathological activation of trypsinogen was completely removed (7,8), indicating that there might be another parallel mechanism that mediates the inflammatory response in the early stage of acute pancreatitis. It was then found that intra-acinar NF-κB activation during the early development of acute pancreatitis was independent from trypsinogen activation. Thus, researchers proposed that NF-κB activation might be responsible for local inflammation and the development of the systemic inflammatory response (7,9-12). Furthermore, Huang et al. proved that NF-κB, which was activated early in acinar cells during acute pancreatitis, provoked the expression of multiple proinflammatory genes, and ultimately increased the severity of acute pancreatitis when transgenes that encoded the NF-κB p65 subunit or the inhibitor of κB kinase 2 (IKK2) were introduced to mice (13-18).
In contrast to the observations regarding NF-κB described above, some controversial results have also been reported in a mouse model with constitutive modifications of the NF-κB gene. A study reported that NF-κB signaling-deficient mice developed more severe pancreatitis when induced by caerulein (19). In another study, in which IkBα was specifically deleted in mouse pancreatic tissue, NF-κB was constitutively activated and acute pancreatitis was ameliorated when mice were administered caerulein and L-arginine (20). These conflicting outcomes highly suggest that there are signaling pathways or proteins that engage in NF-κB regulation and further interfere with the development of acute pancreatitis.
Glycogen synthase kinase-3β (GSK-3β), an isoform of glycogen synthase kinase 3 (GSK-3), is a serine/threonine protein kinase which plays critical roles in multiple pathophysiological processes including cell differentiation, cell cycle control, cell motility, microtubule function, tumorigenesis, and apoptosis (21-24). Recently, it was also found to be involved in inflammation processes (25-28). More importantly, GSK-3β-deficient mice (29) showed a similar phenotype to those that lacked the p65 subunit of NF-κB or IKKβ (30,31). Further investigations revealed that the p65 subunit of NF-κB was a direct phosphorylation target of GSK-3β, which consequently affected NF-κB-dependent gene expression in various cells and tissues (32-34). Moreover, other reports also demonstrated that GSK-3β targeted NF-κB signaling in different pathological processes (35-38). As NF-κB activation provoked the expression of multiple inflammatory mediators and cytokines in pancreatic acinar cells and promoted the development of acute pancreatitis, this research used heterozygous GSK-3β deficient mice(GSK-3β+/− mice) to prove the hypothesis that GSK-3β is involved in caerulein-induced early acute pancreatitis through activating the NF-κB signaling pathway.
We present the following article in accordance with the ARRIVE reporting checklist (available at https://dx.doi.org/10.21037/atm-21-5701).
Methods
Generation of transgenic mice
Paired GSK-3β+/− mice, purchased from Jackson laboratory (strain name: B6.129P2-Gsk3βtm1Dgen/J), were housed in a 12-h light and dark cycle in a specific pathogen-free animal facility with a constant temperature (22±1 °C) and free access to tap water and pelleted chow. Male and female GSK-3β+/− mice were mated to obtain single allele GSK-3β deficiency (GSK-3β+/−) offspring because GSK-3β homozygous knockout (GSK-3β−/−) embryos would die at day E13.5 attributed to TNF-α-induced hepatocyte apoptosis. The genotypes of all descendants, which were consistently maintained in a C57BL/6 background, were determined by RT-PCR according to the protocol of the Jackson laboratory, and wild type (WT) littermates were assigned to the control group randomly. Mice allocation, experiment conduction, and data analysis were completed by 3 investigators independently.
A protocol was prepared before the study without registration. The study was approved by the Animal Ethics Committee of Sichuan University West China Medical School (No. 2021737A), and all procedures with animals were conducted according to the guidelines of the local Animal Use and Care Committees of Chengdu and executed according to the National Animal Welfare Law of China.
Caerulein pancreatitis induction
To induce pancreatitis with caerulein, WT and heterozygote GSK-3β (GSK-3β+/−) littermate mice (n=8), which were 8 to 10 weeks old and weighed approximately 20 g, were fasted for 12 h with water ad libitum. The mice then received an intraperitoneal injection of 50 µg/kg caerulein (Sigma-Aldrich, St. Louis, MO, USA) in saline. WT and GSK-3β+/− control mice (n=6) received an equal amount of saline. All mice were sacrificed by CO2 asphyxiation 30 min after caerulein injection, and blood serum and tissue samples were collected for further research.
Preparation of serum and tissue samples
Whole blood samples were placed at room temperature for 2 h and then centrifuged for 15 min at 3,000 rpm. The serum was collected and stored at −80 °C for measurement of amylase, lipase, and cytokines. Tissues from the pancreas were removed and placed on ice, immediately frozen in liquid nitrogen, and then stored at −80 °C for PCR and western blot analysis.
Measurement of serum amylase and lipase
Serum amylase and lipase were detected using commercially available amylase and lipase assay kits (Nanjing Jiancheng Bioengineering Institute, Nanjing, China) according to the manufacturer’s instructions.
LiquiChip multiple cytokine assay
Serum cytokines, IL-6 and TNF-α, were simultaneously measured on the LiquiChip 100 workstation (Luminex, Austin, TX, USA) using a commercially available luminex assay kit (R&D, USA) according to the manufacturer’s protocol.
Quantitative real-time reverse transcription-polymerase chain reaction (real-time RT-PCR)
Snap frozen pancreatic tissue was pulverized in liquid nitrogen and total RNA was extracted by the Trizol method with RNAiso Plus (TaKaRa, DaLian, China). First-strand cDNA was synthesized from 5 µg total RNA using oligo-dT primers (18mer) and M-MLV reverse transcriptase (TaKaRa, Dalian, China). For real-time PCR amplification, 1-µL cDNA was used for each PCR amplification in a 25-µL reaction system in triplicate with SYBR Green chemistry using the Icycler IQ Multicolor Real-Time Detection System (Bio-Rad, Hercules, CA, USA). Gene-specific, intron-spanning primers were designed with primer premier 5.0 software and synthesized by TaKaRa Biotechnology Co., Ltd. (Dalian, China). The sequences of the primers are provided as follows: IL-6 (forward 5'-AGT CAC AGA AGG AGT GGC TAA-3', reverse 5'-CAC TAG GTT TGC CGA GTA GA-3'), TNF-α (forward 5'-ACG TCG TAG CAA ACC ACC AA-3', reverse 5'-CTG GGA GTA GAT AAG GTA CA-3'), and GAPDH (forward 5'-CCT CAA GAT TGT CAG CAA T-3', reverse 5'-CCA TCC ACA GTC TTC TGA GT-3'). A standard program of real-time PCR was performed with the first denaturation step at 94 °C for 5 min, 40 cycles of denaturation at 94 °C for 20 s, annealing at 55 °C for 30 s, and extension at 72 °C for 30 s. Relative quantitation of the mRNA level of the target gene was calculated using the 2-ΔΔCt method as described previously (39) and was expressed as fold change compared with WT control.
Western blot assay
For the western blot assay, snap frozen mouse pancreatic tissue was rapidly pulverized in liquid nitrogen and the resulting tissue powder was resuspended in ice-cold RIPA buffer containing 1 mM phenylmethanesulfonyl fluoride (Beyotime, Shanghai, China). Samples were centrifuged at 4 °C for 10 min at 12,000 rpm. Supernatants were collected and the concentration of each sample was determined using the Pierce(R) BCA Protein Assay Kit (Pierce, Rockford, Illinois, USA). Then, 30 µg samples of protein were subjected to 12% sodium dodecyl sulfate/polyacrylamide gel electrophoresis and transferred onto polyvinylidene difluoride membranes (Millipore, Billerica, MA, USA). Non-specific binding to the membrane was blocked by 5% (w/v) dry non-fat milk dissolved in Tris-buffer saline/0.05% Tween-20 (TBST) at room temperature for 1 h. Membranes were then incubated with rabbit monoclonal anti-phospho-NF-κB p65 (ser536) antibody (1:500 dilution, Cell Signaling Technology, Beverly, MA, USA) and anti-β-actin antibody (1:1,000 dilution, Cell Signaling Technology, Beverly, MA, USA), which was used as the internal reference, overnight at 4 °C. Membranes were washed for 10 min 3 times with TBST and then incubated with goat polyclonal anti-rabbit IgG (H+L) antibody conjugated to horseradish peroxidase (1:5,000 dilution, KPL, Maryland, USA) diluted in 5% (w/v) dry non-fat milk in TBST for 1 h at room temperature. Finally, after washing for 10 min 3 times with TBST, membranes were developed using the Alpha Innotech FluorChem imaging system (USA) to visualize the target bands with the enhanced chemiluminescence detection system (Millipore, Billerica, MA, USA), and the semi-quantitative grayscale intensity was measured with Quantity One software (Bio-Rad, Hercules, CA, USA).
Statistical analysis
Experimental data were presented as mean ± SD. Statistical analysis was performed using a non-paired Student’s t-test or one way analysis of variance (ANOVA) followed by Scheffe’s post-hoc test when a significant difference was found among groups (SPSS statistical software 16.0; SPSS Inc., Chicago, IL, USA). P values that were less than 0.05 were considered to indicate a significant statistical difference.
Results
Effect of GSK-3β+/− on the level of serum amylase and lipase
Commercially available amylase and lipase assay kits were used to determine the level of serum amylase and lipase. Although the levels of serum amylase and lipase were decreased in GSK-3β+/− mice compared to WT mice, statistical analysis indicated that there was no significant difference between GSK-3β+/− and WT mice at 30 min after caerulein challenge (P>0.05, Figure 1).
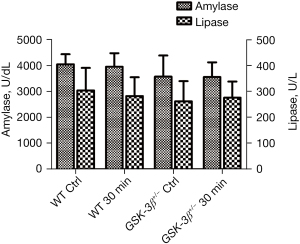
Effect of GSK-3β+/− on the level of serum IL-6 and TNF-α
To determine the level of serum cytokines, IL-6 and TNF-α were simultaneously detected by the LiqiChip multiple cytokine assay system. Compared to the WT control, the levels of serum IL-6 and TNF-α were significantly increased at 30 min after caerulein challenge in WT mice (P<0.01). Moreover, the levels of serum IL-6 and TNF-α in GSK-3β+/− mice were markedly reduced compared with WT mice at 30 min after caerulein challenge (P<0.01). While serum IL-6 and TNF-α were modestly elevated in GSK-3β+/− mice at 30 min after caerulein challenge, only TNF-α was significantly increased compared to the GSK-3β+/− control group (P<0.05, Figure 2A,2B).
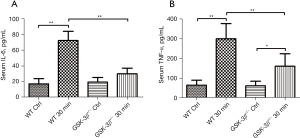
Effect of GSK-3β+/− on the expression of IL-6 and TNF-α mRNA in pancreatic tissue
The expression levels of IL-6 and TNF-α mRNA in pancreatic tissue were detected by real-time RT-PCR. Consistent with the expression trend of serum IL-6 and TNF-α protein, IL-6 and TNF-α mRNA were markedly increased in WT mice at 30 min after caerulein challenge compared to GSK-3β+/− mice and the WT control group (P<0.01). The expression levels of IL-6 and TNF-α mRNA in GSK-3β+/− mice were also moderately increased at 30 min after caerulein challenge compared to the GSK-3β+/− control group, but only the expression of TNF-α mRNA showed a statistically significant difference (P<0.05). Both IL-6 and TNF-α mRNA were significantly decreased compared with WT when challenged by caerulein (P<0.01, Figure 3A,3B).
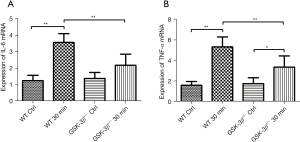
Effect of GSK-3β+/− on the expression of phospho-NF-κB p65 protein
As NF-κB modulates multiple inflammatory mediators and cytokine expression, and the p65 subunit has a phosphorylation site of GSK-3β, we detected phospho-NF-κB p65 (ser536) protein expression in pancreatic tissue by western blot analysis. Phospho-NF-κB p65 (ser536) protein was significantly elevated in WT mice at 30 min after caerulein challenge compared to the WT control group. However, it was slightly elevated in GSK-3β+/− mice, which was striking decreased compared with WT mice, at 30 min after caerulein challenge (Figure 4).
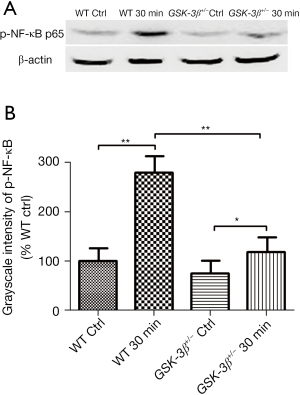
Discussion
As a common clinical emergency, acute pancreatitis has considerable morbidity and mortality. However, specific and effective treatments to ameliorate acute pancreatitis are lacking, which can be attributed to the insufficient understanding of the etiopathogenesis and pathophysiological progression of pancreatitis. Recent research has indicated that intra-acinar trypsinogen and NF-κB activation during the early development of acute pancreatitis might be two key independent factors that result in acinar insult and the progression of local and systemic inflammatory responses (7-12), respectively. However, paradoxical results have been demonstrated from different reports (19,20), implying that the underlying mechanisms of the development of acute pancreatitis, remains to be further investigated.
GSK-3β, a multifunctional phosphorylase kinase, was discovered to be involved in multiple pathophysiological processes (21-24). Recent research revealed that GSK-3β played various but critical roles in inflammatory reactions (25-28). Our former research about the expression of GSK-3β in the acute edematous pancreatitis shows that both GSK-3βmRNA and protein expression level were associated with the severity of the disease (40). Also, Jin also found that the expression of GSK-3β is elevated in acute necrotizing pancreatitis) (41). It could be assumed that GSK-3β was activated in acute pancreatitis and was associated with the severity of the pancreatitis. The latest data showed that GSK-3β could directly phosphorylate the NF-κB p65 subunit and consequently regulate the transcription of NF-κB-dependent gene transcription (32-34). Moreover, GSK-3β-deficient mice presented a phenotype similar to that of p65 subunit or IKKβ knock-out mice (29-31). As GSK-3β knockout homozygous mice will die mid gestation or as newborns due to severe liver degeneration (29), in this research, we utilized single allele GSK-3β-deficient (GSK-3β+/−) mice to investigate whether GSK-3β interferes with the early development of acute pancreatitis in vivo through the NF-κB signaling pathway. In the western blot assay, pancreatic GSK-3β protein in GSK-3β+/− mice decreased more than 60% compared to WT mice (data not shown), so this research based on heterozygous transgenic mice is reliable. Although serum amylase and lipase levels slightly declined in GSK-3β+/− mice compared to the respective control, we did not detect a significant difference between GSK-3β+/− and WT mice challenged by caerulein. We propose that GSK-3β may not induce intra-acinar trypsinogen activation, which is responsible for acinar injury and consequently increases serum amylase and lipase during early acute pancreatitis (7-9). Meanwhile, we did not observe obvious morphological changes in the pancreatic tissues of all groups by hematoxylin and eosin (HE) staining (data not shown), such as pancreatic acinar necrosis and inflammatory cell infiltration, which to some extent supports the results that came from the amylase and lipase data.
Several lines of evidence revealed that acute pancreatitis was initiated in acinar cells and that SIRS and multiple organ failure were consequences of the synthesis and release of proinflammatory cytokines and chemokines in injured acinar cells (42-45). Therefore, we detected the expression of IL-6 and TNF-α mRNA in pancreatic tissue and measured the levels of serum IL-6 and TNF-α. Consistent with previous research, when using small interfering RNA-mediated knockdown of GSK-3β or a panel of different GSK-3-selective pharmacological inhibitors (46), our data showed that expression levels of IL-6 and TNF-α mRNA in pancreatic tissue were significantly elevated in WT mice at 30 min after caerulein challenge compared with GSK-3β+/− mice and the WT control, which was consistent with the trend of the serum level of IL-6 and TNF-α. The expression levels of IL-6 and TNF-α mRNA in WT mice were increased 2.8 and 3.4 folds, respectively, after caerulein challenge, but in GSK-3β+/− mice the expression of IL-6 and TNF-α mRNA both declined approximately 40% compared with the WT mice. However, serum IL-6 and TNF-α were increased 4.2 and 4.6 folds, respectively, after caerulein challenge, while IL-6 was decreased by approximately 60% and TNF-α was decreased by 50% in GSK-3β+/− mice compared with the WT mice. Despite overwhelming evidence suggesting that the recruitment of inflammatory cells into the pancreas is the predominant source of cytokines (10,47,48), the synthesis and release of cytokines in pancreatic acinar cells often occur within 30 min after caerulein challenge and prior to recognizable pathohistological changes (14,47,49). According to our observation, when increasing cytokines were detected the inflammatory cells have not been recruited. Our pathomorphological results found that no infiltrating inflammatory cells and histological injury were observed by HE staining after 30 min challenge by caerulein. Thus, we proposed that the marked increase of serum IL-6 and TNF-α at the specific time point might not be related to the activation of inflammatory cells but was associated with other pathways. Besides proinflammatory cytokines, it is known that IL-10, an anti-inflammatory cytokine, plays a pivotal role in controlling excessive inflammation and maintaining immune homeostasis. In contrast to previous results which demonstrated that GSK-3β modulated the Toll like receptor (TLR)-induced inflammatory response of blood monocytes and promoted the production of proinflammatory cytokines such as IL-1β, IL-6, TNF, IL-12, and IFN-γ while concurrently suppressing IL-10 secretion (46,50-52), we did not detect the promotion of serum IL-10 in GSK-3β+/− mice after caerulein challenge (data not shown). Moreover, contrary to Jhun et al.’s results (53), we did not find that serum IL-2 was elevated in WT mice challenged by caerulein (data not shown). It is possible that the contrasting results of the serum levels of IL-2 and IL-10 may be caused by virulence factors, the experimental model, and the stage of acute pancreatitis.
Previous research has indicated that GSK-3β participates in the regulation of the eukaryotic transcription factor NF-κB, which modulates many diverse cellular processes, including promoting proinflammatory cytokine synthesis and release (29,32,54-56). Several researches used GSK-3β inhibitors to investigate the role of it in acute pancreatitis to explore the relationship between GSK-3β and NF-κB pathway. It was found that 4-benzyl-2-methyl-1,2,4-thiadiazolidine-3,5-dione (TDZD-8), an inhibitor of GSK-3β, could ameliorate the severity of sodium taurocholate-induced severe acute pancreatitis (SAP) pancreatitis in the rat model and reduced the expression level of NF-κB (57). Similar results also have been found in cerulein-induced acute pancreatitis mice model (58). And different inhibitors showed consistent result that GSK-3β inhibition could alleviate acute pancreatitis through NF-κB pathway (41). It is well known that the NF-κB/HIF-1α pathway involves in intestinal barrier dysfunction. Further research showed that NF-κB could be activated to induce the intestinal mucosal barrier injury in mice with acute pancreatitis (59). So it could be critical to further investigate the role of NF-κB since it is not only function in the progression of acute pancreatitis but also involves in the systematic complications of acute pancreatitis. In this research, we utilized a phosphor-NF-κB p65 (ser536) monoclonal antibody to investigate whether GSK-3β modulated the NF-κB signal pathway by western blot assay. Our data showed that phospho-NF-κB p65 protein expression was significantly increased in WT mice, but in GSK-3β+/− mice the protein expression was decreased approximately 60% compared to WT mice at 30 min after caerulein challenge, which was consistent with previous reports (52,60-62).
This study also shows some limitations. Although the animal model was highly effective for investigating the mechanisms of acute pancreatitis, it would be more convincing to establish a GSK-3β full deletion model. This may be achieved by using the technique of temporal and spatial control of gene expression in the mouse model, which has been established in congenital lung diseases (63).
Conclusions
In summary, our research, for the first time, proved that GSK-3β might activate NF-κB in pancreatic acinar cells and consequently promote the synthesis and release of proinflammatory cytokines by acinar cells at the early stage of the development of acute pancreatitis after caerulein challenge in vivo using GSK-3β heterozygote transgenic mice. Consequently, proinflammatory cytokines secreted by acinar cells might recruit inflammatory cells into pancreatic tissue and then promote and aggravate acute pancreatitis. Thus, we conclude that GSK-3β may be an ideal therapeutic target in the early stage of acute pancreatitis, and a GSK-3β inhibitor may provide beneficial effects in prophylaxis, surgical intervention, and endoscopic retrograde cholangiopancreatography (ERCP)-induced acute pancreatitis.
Acknowledgments
Funding: This study was supported by grants from the National Natural Science Fund of China (NSFC projects 81500486 and 81800575) and 1.3.5 project for disciplines of excellence, West China Hospital, Sichuan University (2016105 and ZYGD20006).
Footnote
Reporting Checklist: The authors have completed the ARRIVE reporting checklist. Available at https://dx.doi.org/10.21037/atm-21-5701
Data Sharing Statement: Available at https://dx.doi.org/10.21037/atm-21-5701
Conflicts of Interest: All authors have completed the ICMJE uniform disclosure form (available at https://dx.doi.org/10.21037/atm-21-5701). YL and ZZ report that this study was supported by grants from the National Natural Science Fund of China (NSFC projects 81500486 and 81800575) and 1.3.5 project for disciplines of excellence, West China Hospital, Sichuan University (2016105 and ZYGD20006). The other authors have no conflicts of interest to declare.
Ethical Statement: The authors are accountable for all aspects of the work in ensuring that questions related to the accuracy or integrity of any part of the work are appropriately investigated and resolved. The study was approved by the Animal Ethics Committee of Sichuan University West China Medical School (No. 2021737A), and all procedures with animals were conducted according to the guidelines of the local Animal Use and Care Committees of Chengdu and executed according to the National Animal Welfare Law of China.
Open Access Statement: This is an Open Access article distributed in accordance with the Creative Commons Attribution-NonCommercial-NoDerivs 4.0 International License (CC BY-NC-ND 4.0), which permits the non-commercial replication and distribution of the article with the strict proviso that no changes or edits are made and the original work is properly cited (including links to both the formal publication through the relevant DOI and the license). See: https://creativecommons.org/licenses/by-nc-nd/4.0/.
References
- Roberts SE, Morrison-Rees S, John A, et al. The incidence and aetiology of acute pancreatitis across Europe. Pancreatology 2017;17:155-65. [Crossref] [PubMed]
- Boxhoorn L, Voermans RP, Bouwense SA, et al. Acute pancreatitis. Lancet 2020;396:726-34. [Crossref] [PubMed]
- van Santvoort HC, Bakker OJ, Bollen TL, et al. A conservative and minimally invasive approach to necrotizing pancreatitis improves outcome. Gastroenterology 2011;141:1254-63. [Crossref] [PubMed]
- Lankisch PG, Apte M, Banks PA. Acute pancreatitis. Lancet 2015;386:85-96. [Crossref] [PubMed]
- Chiari H. Über die Selbstverdauung des menschlichen Pankreas. Z. Heilkunde 1896;17:69-96.
- Hofbauer B, Saluja AK, Lerch MM, et al. Intra-acinar cell activation of trypsinogen during caerulein-induced pancreatitis in rats. Am J Physiol 1998;275:G352-62. [PubMed]
- Saluja A, Dudeja V, Dawra R, et al. Early Intra-Acinar Events in Pathogenesis of Pancreatitis. Gastroenterology 2019;156:1979-93. [Crossref] [PubMed]
- Halangk W, Lerch MM, Brandt-Nedelev B, et al. Role of cathepsin B in intracellular trypsinogen activation and the onset of acute pancreatitis. J Clin Invest 2000;106:773-81. [Crossref] [PubMed]
- Ji B, Gaiser S, Chen X, et al. Intracellular trypsin induces pancreatic acinar cell death but not NF-kappaB activation. J Biol Chem 2009;284:17488-98. [Crossref] [PubMed]
- Chen X, Ji B, Han B, et al. NF-kappaB activation in pancreas induces pancreatic and systemic inflammatory response. Gastroenterology 2002;122:448-57. [Crossref] [PubMed]
- Baumann B, Wagner M, Aleksic T, et al. Constitutive IKK2 activation in acinar cells is sufficient to induce pancreatitis in vivo. J Clin Invest 2007;117:1502-13. [Crossref] [PubMed]
- Aleksic T, Baumann B, Wagner M, et al. Cellular immune reaction in the pancreas is induced by constitutively active IkappaB kinase-2. Gut 2007;56:227-36. [Crossref] [PubMed]
- Huang H, Liu Y, Daniluk J, et al. Activation of nuclear factor-κB in acinar cells increases the severity of pancreatitis in mice. Gastroenterology 2013;144:202-10. [Crossref] [PubMed]
- Gukovsky I, Gukovskaya AS, Blinman TA, et al. Early NF-kappaB activation is associated with hormone-induced pancreatitis. Am J Physiol 1998;275:G1402-14. [PubMed]
- Satoh A, Shimosegawa T, Fujita M, et al. Inhibition of nuclear factor-kappaB activation improves the survival of rats with taurocholate pancreatitis. Gut 1999;44:253-8. [Crossref] [PubMed]
- Steinle AU, Weidenbach H, Wagner M, et al. NF-kappaB/Rel activation in cerulein pancreatitis. Gastroenterology 1999;116:420-30. [Crossref] [PubMed]
- Han B, Ji B, Logsdon CD. CCK independently activates intracellular trypsinogen and NF-kappaB in rat pancreatic acinar cells. Am J Physiol Cell Physiol 2001;280:C465-72. [Crossref] [PubMed]
- Altavilla D, Famulari C, Passaniti M, et al. Attenuated cerulein-induced pancreatitis in nuclear factor-kappaB-deficient mice. Lab Invest 2003;83:1723-32. [Crossref] [PubMed]
- Algül H, Treiber M, Lesina M, et al. Pancreas-specific RelA/p65 truncation increases susceptibility of acini to inflammation-associated cell death following cerulein pancreatitis. J Clin Invest 2007;117:1490-501. [Crossref] [PubMed]
- Neuhöfer P, Liang S, Einwächter H, et al. Deletion of IκBα activates RelA to reduce acute pancreatitis in mice through up-regulation of Spi2A. Gastroenterology 2013;144:192-201. [Crossref] [PubMed]
- Doble BW, Woodgett JR. GSK-3: tricks of the trade for a multi-tasking kinase. J Cell Sci 2003;116:1175-86. [Crossref] [PubMed]
- Jope RS, Johnson GV. The glamour and gloom of glycogen synthase kinase-3. Trends Biochem Sci 2004;29:95-102. [Crossref] [PubMed]
- Kockeritz L, Doble B, Patel S, et al. Glycogen synthase kinase-3--an overview of an over-achieving protein kinase. Curr Drug Targets 2006;7:1377-88. [Crossref] [PubMed]
- Forde JE, Dale TC. Glycogen synthase kinase 3: a key regulator of cellular fate. Cell Mol Life Sci 2007;64:1930-44. [Crossref] [PubMed]
- Jope RS, Yuskaitis CJ, Beurel E. Glycogen synthase kinase-3 (GSK3): inflammation, diseases, and therapeutics. Neurochem Res 2007;32:577-95. [Crossref] [PubMed]
- Wang H, Brown J, Martin M. Glycogen synthase kinase 3: a point of convergence for the host inflammatory response. Cytokine 2011;53:130-40. [Crossref] [PubMed]
- Dugo L, Collin M, Thiemermann C. Glycogen synthase kinase 3beta as a target for the therapy of shock and inflammation. Shock 2007;27:113-23. [Crossref] [PubMed]
- Cortés-Vieyra R, Bravo-Patiño A, Valdez-Alarcón JJ, et al. Role of glycogen synthase kinase-3 beta in the inflammatory response caused by bacterial pathogens. J Inflamm (Lond) 2012;9:23. [Crossref] [PubMed]
- Hoeflich KP, Luo J, Rubie EA, et al. Requirement for glycogen synthase kinase-3beta in cell survival and NF-kappaB activation. Nature 2000;406:86-90. [Crossref] [PubMed]
- Beg AA, Sha WC, Bronson RT, et al. Embryonic lethality and liver degeneration in mice lacking the RelA component of NF-kappa B. Nature 1995;376:167-70. [Crossref] [PubMed]
- Li Q, Van Antwerp D, Mercurio F, et al. Severe liver degeneration in mice lacking the IkappaB kinase 2 gene. Science 1999;284:321-5. [Crossref] [PubMed]
- Schwabe RF, Brenner DA. Role of glycogen synthase kinase-3 in TNF-alpha-induced NF-kappaB activation and apoptosis in hepatocytes. Am J Physiol Gastrointest Liver Physiol 2002;283:G204-11. [Crossref] [PubMed]
- Buss H, Dörrie A, Schmitz ML, et al. Phosphorylation of serine 468 by GSK-3beta negatively regulates basal p65 NF-kappaB activity. J Biol Chem 2004;279:49571-4. [Crossref] [PubMed]
- Itoh S, Saito T, Hirata M, et al. GSK-3α and GSK-3β proteins are involved in early stages of chondrocyte differentiation with functional redundancy through RelA protein phosphorylation. J Biol Chem 2012;287:29227-36. [Crossref] [PubMed]
- Steinbrecher KA, Wilson W 3rd, Cogswell PC, et al. Glycogen synthase kinase 3beta functions to specify gene-specific, NF-kappaB-dependent transcription. Mol Cell Biol 2005;25:8444-55. [Crossref] [PubMed]
- Götschel F, Kern C, Lang S, et al. Inhibition of GSK3 differentially modulates NF-kappaB, CREB, AP-1 and beta-catenin signaling in hepatocytes, but fails to promote TNF-alpha-induced apoptosis. Exp Cell Res 2008;314:1351-66. [Crossref] [PubMed]
- Ougolkov AV, Fernandez-Zapico ME, Savoy DN, et al. Glycogen synthase kinase-3beta participates in nuclear factor kappaB-mediated gene transcription and cell survival in pancreatic cancer cells. Cancer Res 2005;65:2076-81. [Crossref] [PubMed]
- Tang QL, Xie XB, Wang J, et al. Glycogen synthase kinase-3β, NF-κB signaling, and tumorigenesis of human osteosarcoma. J Natl Cancer Inst 2012;104:749-63. [Crossref] [PubMed]
- Schmittgen TD, Livak KJ. Analyzing real-time PCR data by the comparative C(T) method. Nat Protoc 2008;3:1101-8. [Crossref] [PubMed]
- Chen LH, Zhou ZG, Jiang JJ, et al. Expression of glycogen synthase kinase-3B in pancreatic tissue of acute edematous pancreatitis rats. Sichuan Da Xue Xue Bao Yi Xue Ban 2011;42:194-8. [PubMed]
- Jin H, Yang X, Zhao K, et al. Glycogen synthase kinase-3 beta inhibitors protectagainst the acute lung injuries resulting from acute necrotizing pancreatitis. Acta Cir Bras 2019;34:e201900609 [Crossref] [PubMed]
- Gukovskaya AS, Gukovsky I, Zaninovic V, et al. Pancreatic acinar cells produce, release, and respond to tumor necrosis factor-alpha. Role in regulating cell death and pancreatitis. J Clin Invest 1997;100:1853-62. [Crossref] [PubMed]
- Grady T, Liang P, Ernst SA, et al. Chemokine gene expression in rat pancreatic acinar cells is an early event associated with acute pancreatitis. Gastroenterology 1997;113:1966-75. [Crossref] [PubMed]
- Halangk W, Lerch MM. Early events in acute pancreatitis. Gastroenterol Clin North Am 2004;33:717-31. [Crossref] [PubMed]
- Bhatia M, Wong FL, Cao Y, et al. Pathophysiology of acute pancreatitis. Pancreatology 2005;5:132-44. [Crossref] [PubMed]
- Martin M, Rehani K, Jope RS, et al. Toll-like receptor-mediated cytokine production is differentially regulated by glycogen synthase kinase 3. Nat Immunol 2005;6:777-84. [Crossref] [PubMed]
- Fink GW, Norman JG. Specific changes in the pancreatic expression of the interleukin 1 family of genes during experimental acute pancreatitis. Cytokine 1997;9:1023-7. [Crossref] [PubMed]
- Bhatia M, Brady M, Shokuhi S, et al. Inflammatory mediators in acute pancreatitis. J Pathol 2000;190:117-25. [Crossref] [PubMed]
- Norman JG, Fink GW, Franz MG. Acute pancreatitis induces intrapancreatic tumor necrosis factor gene expression. Arch Surg 1995;130:966-70. [Crossref] [PubMed]
- Hu X, Paik PK, Chen J, et al. IFN-gamma suppresses IL-10 production and synergizes with TLR2 by regulating GSK3 and CREB/AP-1 proteins. Immunity 2006;24:563-74. [Crossref] [PubMed]
- Zhang P, Katz J, Michalek SM. Glycogen synthase kinase-3beta (GSK3beta) inhibition suppresses the inflammatory response to Francisella infection and protects against tularemia in mice. Mol Immunol 2009;46:677-87. [Crossref] [PubMed]
- Dugo L, Collin M, Allen DA, et al. GSK-3beta inhibitors attenuate the organ injury/dysfunction caused by endotoxemia in the rat. Crit Care Med 2005;33:1903-12. [Crossref] [PubMed]
- Jhun BS, Oh YT, Lee JY, et al. AICAR suppresses IL-2 expression through inhibition of GSK-3 phosphorylation and NF-AT activation in Jurkat T cells. Biochem Biophys Res Commun 2005;332:339-46. [Crossref] [PubMed]
- Demarchi F, Bertoli C, Sandy P, et al. Glycogen synthase kinase-3 beta regulates NF-kappa B1/p105 stability. J Biol Chem 2003;278:39583-90. [Crossref] [PubMed]
- Németh ZH, Deitch EA, Szabó C, et al. Lithium induces NF-kappa B activation and interleukin-8 production in human intestinal epithelial cells. J Biol Chem 2002;277:7713-9. [Crossref] [PubMed]
- Takada Y, Fang X, Jamaluddin MS, et al. Genetic deletion of glycogen synthase kinase-3beta abrogates activation of IkappaBalpha kinase, JNK, Akt, and p44/p42 MAPK but potentiates apoptosis induced by tumor necrosis factor. J Biol Chem 2004;279:39541-54. [Crossref] [PubMed]
- Zhao K, Chen C, Shi Q, et al. Inhibition of glycogen synthase kinase-3β attenuates acute kidney injury in sodium taurocholate-induced severe acute pancreatitis in rats. Mol Med Rep 2014;10:3185-92. [Crossref] [PubMed]
- Cuzzocrea S, Malleo G, Genovese T, et al. Effects of glycogen synthase kinase-3beta inhibition on the development of cerulein-induced acute pancreatitis in mice. Crit Care Med 2007;35:2811-21. [PubMed]
- Deng W, Abliz A, Xu S, et al. Severity of pancreatitis-associated intestinal mucosal barrier injury is reduced following treatment with the NADPH oxidase inhibitor apocynin. Mol Med Rep 2016;14:3525-34. [Crossref] [PubMed]
- Cuzzocrea S, Crisafulli C, Mazzon E, et al. Inhibition of glycogen synthase kinase-3beta attenuates the development of carrageenan-induced lung injury in mice. Br J Pharmacol 2006;149:687-702. [Crossref] [PubMed]
- Cuzzocrea S, Di Paola R, Mazzon E, et al. Glycogen synthase kinase 3beta inhibition reduces the development of nonseptic shock induced by zymosan in mice. Shock 2007;27:97-107. [Crossref] [PubMed]
- Dugo L, Abdelrahman M, Murch O, et al. Glycogen synthase kinase-3beta inhibitors protect against the organ injury and dysfunction caused by hemorrhage and resuscitation. Shock 2006;25:485-91. [Crossref] [PubMed]
- Miao Q, Chen H, Luo Y, et al. Abrogation of mesenchyme-specific TGF-β signaling results in lung malformation with prenatal pulmonary cysts in mice. Am J Physiol Lung Cell Mol Physiol 2021;320:L1158-68. [Crossref] [PubMed]
(English Language Editor: C. Betlazar-Maseh)