Berberine attenuates atherosclerotic lesions and hepatic steatosis in ApoE-/- mice by down-regulating PCSK9 via ERK1/2 pathway
Introduction
Atherosclerosis is a complex chronic inflammatory and metabolic disease in which aberrant inflammatory responses and dysregulation of lipid metabolism in the arterial walls at predisposed sites plays an important role from the initiation to progression and eventually rupture of the atherosclerotic plaque (1,2). Atherosclerosis and its complications considerably cause increased morbidity and mortality worldwide and account for almost a third of the deaths in the world (3). Till now, dyslipidemia, mainly presented as elevated low-density lipoprotein (LDL) cholesterol (LDL-C) level, and inflammation, usually characterized by a chronic and low-level inflammatory status, have been considered as two important risk factors of atherosclerosis. In fact, a large number of studies clearly showed that a reduction in the circulating levels of total cholesterol (TC) and LDL-C could decrease the risk for atherosclerosis. In addition, it has been demonstrated that inflammation is a key process for the initiation of atherosclerosis in the early stage in which circulating monocytes in the blood adhere to the endothelium and migrate into the subendothelial space (4). Macrophages would be activated and oxidized lipoprotein particles are deposited under endothelial cells. Later, an inflammatory response cascade occurs as a result of endothelium damage. Increased production of pro-inflammatory mediators takes part in the initiation and development of atherosclerosis (5). Although the big progress has been made in the prevention and treatment of atherosclerosis during past several decades, atherosclerotic cardiovascular disease (ASCVD) remains a main cause for human’s mortality throughout the world due to an unsatisfactory status for atherosclerosis treatment (6), suggesting a huge room for improvement.
Several previous studies have shown that traditional Chinese medicine appears an alternative strategy in the prevention and treatment of atherosclerosis (7,8). Berberine (BBR) is an isoquinoline alkaloid isolated from coptis chinensis (9). Published investigations have revealed that BBR is an effective traditional Chinese herbal in treating nearly a dozen of disorders, such as bacterial infection, diabetes, hypertension, and obesity et al. (10-13). Our previous study found that BBR significantly reduced body weight gain and improved lipid profile in high fat diet-fed rat (14). More interestingly, data also showed that BBR improved the dyslipidemia in both animal and human studies, resulting in beneficial effects on atherosclerosis (15,16). Nevertheless, the exact mechanisms regarding the effects of BBR on dyslipidemia and atherosclerosis have not been fully examined. Thereby, the present study aimed to investigate the effects of BBR on lipid and atherosclerosis in ApoE-/- mice and potential mechanisms.
We present the following article in accordance with the ARRIVE reporting checklist (available at https://dx.doi.org/10.21037/atm-20-8106).
Methods
Animals and regents
Male C57BL/6J mice (n=7, 8-week-old) and male ApoE-/- mice (n=28, 8-week-old) were purchased from Beijing Vital River Laboratory Animal Technology Co., Ltd. Berberine was purchased from Sigma-Aldrich (St. Louis, MO, USA). The human hepatoma cell line, HepG2, was obtained from Cell Resource Center, IBMS, CAMS/PUMC (Beijing, China), and U0126 (ERK1/2 inhibitor) was purchased from Cell Signaling Technology (Beverly, MA). Anti-proprotein convertase subtilisin/kexin type 9 (PCSK9), anti-LDL receptor (LDLR), and anti-GAPDH antibodies were obtained from Abcam (Cambridge, UK). Antibodies against phospho-ERK1/2 and total ERK1/2 were purchased from Cell Signaling Technology (Beverly, MA). The experiments were performed under a project license (No. 0000555) granted by the animal experimental center of Fuwai hospital, in compliance with the guidelines of the Care and Use of Laboratory Animals published by the US National Institutes of Health.
Animal treatment
All animals were maintained in an air-conditioned environment with a controlled temperature at 22±2 °C and relative humidity of 50–60% under a 12 hours:12 hours light/dark cycle. After an adaptation period of a week, all mice were divided into the following groups: group 1 [wild type C57BL/6J mice with normal diet (ND), n=7], group 2 (ApoE-/- mice with ND, n=7), group 3 [ApoE-/- mice with high-fat diet (HFD), n=7], group 4 (ApoE-/- mice with HFD and treatment with low dose BBR of 50 mg/kg/d, i.g., n=7), and group 5 (ApoE-/- mice with HFD and treatment with high BBR of 100 mg/kg/d, i.g., n=7). After 16-week treatment, mice were euthanized using 1% sodium pentobarbital (50 mg/kg) after a 4-hour fast. Blood samples were taken from the retro-orbital plexus and centrifuged. The subsequent serum was used to determine blood lipid parameters. The left ventricle was perfused with 4% neutral paraformaldehyde for 1 hour, then the abdominal cavity was opened and the liver, spleen and kidney were removed in turn. And then, the chest cavity was opened, the sternum was removed, the heart was exposed and the heart and aorta were removed.
Serum lipids analysis
According to the manufacturer's instructions, serum was prepared from each blood sample by centrifugation at 3,500 rpm for 10 min. The LDL-C concentration was analyzed by a selective solubilization method (LDL-C Test Kit, Kyowa Medex, Tokyo, Japan) with a coefficient of variation of<5% and a total imprecision of <10%. The high density lipoprotein cholesterol (HDL-C) concentration was determined by a homogeneous method (Determiner L HDL, Kyowa Medex, Tokyo, Japan) with a coefficient of variation of <5% and a total imprecision of <10%. TC and triglyceride (TG) levels were measured with enzymatic assay by the automatic biochemistry analyzer (Hitachi 917, Tokyo, Japan).
Hematoxylin-eosin staining
Mouse liver specimens were processed according to a standard hematoxylin-eosin (HE) staining technique (17). Briefly, liver tissues were fixed by 10% neutral formalin, dehydrated in ethanol, and then embedded. Subsequently, liver sections (4 μm) were stained with HE for pathological changes under an optical microscope.
Oil red O staining
Heart tissues containing the aortic arch were excised from the proximal aortic root to the iliac artery branch and placed in 4% paraformaldehyde environment for 6 hours. The atherosclerotic lesion area of aorta was evaluated by Oil red O staining. Measurement of the lesion size was based on HE staining of paraffin embedded aortic sections. The area and size of the lesion were analyzed by bright-field microscopy (Leica, Wetzlar, Germany) using Image J soft.
Mouse liver tissues were immediately snap-frozen in liquid nitrogen and placed in OCT cryostat embedding compound (Tissue-Tek, Torrance, CA, USA). Frozen liver sections (8 μm) were stained with oil red O according to previous report (18), and the intracellular lipid droplets were observed and assessed by bright-field microscopy (Leica, Wetzlar, Germany).
Real time quantitative PCR (qRT-PCR) assay
SYBR green quantitative real-time polymerase chain reaction (qRT-PCR) was used to detect mRNA levels of PCSK9, LDLR, ABCA1, ABCG1, and SR-B1. The Trizol method was used to extract total RNA from mouse liver tissue. RNA yield and purity was confirmed by measuring the ratio of the absorbance at 260 and 280 nm. cDNA was synthesized using the SuperScript III First-Strand Synthesis System. The qRT-PCR reaction, containing target genes and SYBR Green PCR master mix, was performed on a Bio-Rad CFX connect real-time system (Bio-Rad, USA). The qRT-PCR containing target genes and SYBR Green PCR master mix was carried out on a Bio-Rad CFX connect real-time system (Bio Rad, USA) at 95 °C for 3 min, cycled at 95 °C for 10 s, 56 °C for 30 s and 72 °C for 30 s for 42 cycles. Melt curves were performed from 56.0 °C to 95.0 °C with intervals at 0.5 °C for 5 seconds. Relative RNA levels were determined by analyzing the changes in SYBR Green fluorescence by the 2−ΔΔCT method according to the manufacturer’s instructions. GAPDH was amplified in parallel and the results were used for normalization. The PCR product was confirmed by gel electrophoresis on a 2% agarose gel stained with ethidium bromide. Purity of amplified PCR products was determined by melting point analysis using ICycler software. All experiments were performed in triplicate.
Western blot
Total proteins were extracted from liver (100 mg) derived from ApoE-/-mice. Liver were homogenized in 1ml of RIPA lysis buffer containing protease inhibitors and vortexed on ice for 30 minutes. After centrifugation at 12,000×g for 20 minutes, the supernatant containing total protein extract was collected and protein concentrations were determined by the bicinchoninic acid (BCA) method. Cytosolic proteins were extracted using cytosol protein kits. Equal amounts of proteins (20–40 μg) were electrophoresed on 8–12% SDS-PAGE gels for 2 hours at 90 Voltage and electrotransferred onto PVDF membranes at 125 mA for 1–2 hours. The membranes were blocked with 5% non-fat dry milk in 20 mM Tris-HCl, pH 7.4, 0.15 M NaCl, 0.05% Tween-20 (Tris-Buffered Saline and Tween 20, TBST) for 1 hour at room temperature, and then membranes were incubated overnight at 4 °C with one of the following primary antibodies: anti-PCSK9, anti-LDLR, anti-ABCA1, anti-ABCG1, and anti-SR-BI. After washing with TBST, membranes were incubated with secondary antibodies derived from goat for 1.5 hours at room temperature. The results of western blot were analyzed by the Image J program. The expression of each protein was normalized to the corresponding GAPDH.
Statistical analysis
Data are presented as mean ± standard error of the mean unless otherwise stated. Comparisons between two groups were assessed using an unpaired two-tailed Student t-test. One-way ANOVA combined with Bonferroni’s post hoc test was used among ≥3 groups. P<0.05 was considered statistically significant, and P<0.01 was considered extremely significant. All analyses were performed using SPSS 19.0 (SPSS Inc., Chicago, IL, USA).
Results
BBR improved lipid metabolism in the serum
After BBR treatment for 16 weeks, blood samples were collected for examining TC, TG, LDL-C and HDL-C concentrations. As shown in Figure 1, the serum TC, TG and LDL-C levels in group of ApoE-/- mice fed with HFD were significantly higher than that in group of ApoE-/- mice fed with ND. However, BBR treatment at the doses of 50 and 100 mg/kg/d could significantly decrease serum TC, TG and LDL-C and increased HDL-C levels in ApoE-/- mice fed with HFD.
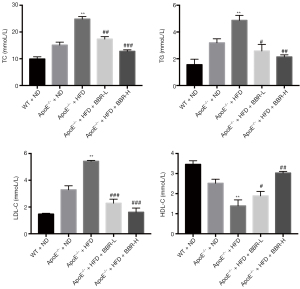
BBR administration reduced atherosclerosis progression in ApoE-/- mice fed with HFD
Data showed that more plaques were found in ApoE-/- mice fed with HFD, while low-dose of BBR (50 mg/kg/d) and high-dose of BBR (100 mg/kg/d) significantly reduced the numbers of plaques (Figure 2).
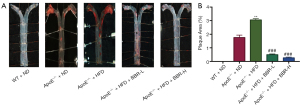
Furthermore, the lipid foam like macrophages and vacuolated fibroid cells were found in aorta by HE staining in ApoE-/- mice fed with HFD (Figure 3). At the same time, the more lipid droplets were detected in ApoE-/- mice fed with HFD compared with that in wild type mice by oil red O staining exanimation. Thereby, the present data suggested that low dose of BBR (50 mg/kg/d) and high dose of BBR (100 mg/kg/d) attenuated aortic lesions in ApoE-/- mice fed with HFD.
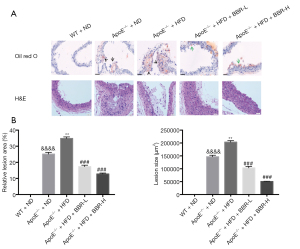
BBR reduced lipid accumulation in the liver
On the other hand, by HE and oil red O staining analysis, data showed a fatty degeneration in the liver section in ApoE-/- mice fed with HFD (Figure 4). And also, the lipid droplets and lipid deposition were also observed in in ApoE-/- mice fed with HFD. BBR treatment at the dose of 50 and 100 mg/kg/d significantly alleviated liver lipid deposition in a dose-dependent manner in those animals.
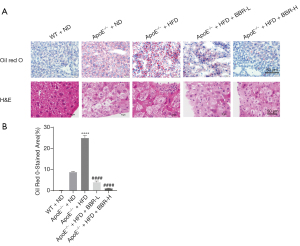
BBR modified the expression of lipid metabolism-related genes and proteins
Compared with wild-type mice, the lower expressions of LDLR, ABCA1, ABCG1 and SR-B1 were found in the liver of ApoE-/- mice fed with HFD. Low dose of BBR (50 mg/kg/d) and high dose of BBR (100 mg/kg/d) could enhance the expressions of LDLR, ABCA1, ABCG1 and SR-B1 in liver of ApoE-/- mice, and decrease the level of PCSK9 (Figure 5).
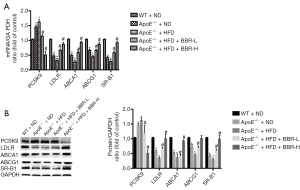
BBR decreased PCSK9 expression by activating the ERK1/2 pathway
HepG2 cells were treated with BBR (0, 5, 25, and 50 μg/mL) for 24 hours. Subsequently, we determined whether BBR could affect the expression of PCSK9 and LDLR in HepG2, and found that BBR exhibited the effects on the up-regulation of LDLR expression, which was accompanied by a steady decline of PCSK9 level (Figure 6A).
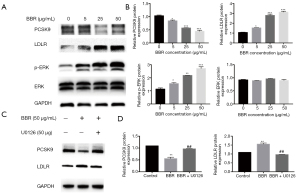
We next examined the possible involvement of MAPK/ERK1/2 signal pathway in BBR-induced changes of PCSK9 expression. As well known, ERK1/2 is one member of MAPKKs family, which an important kinase is involving in many kinds of biological physiological process. To test this hypothesis, HepG2 cells were firstly subjected to serum-starvation for 12 hours, and then stimulated with BBR (0, 5, 25, and 50 μg/mL) for 24 hours. Finally, the phosphorylation of ERK1/2 was analyzed by western blotting using anti-phospho-ERK1/2 antibody. Data showed that exposure of HepG2 cells to BBR resulted in enhanced levels of ERK1/2 phosphorylation in a dose-dependent manner, which started at concentration of 5 μg/mL (Figure 6A,6B). The further studies indicated that the decrease in PCSK9 expression caused by BBR was partly reversed by the ERK1/2 inhibitor U0126 (50 µM), suggesting that an ERK1/2 pathway might be involved in such effect (Figure 6C,6D). Briefly, BBR decreased PCSK9 expression in HepG2 cells through activating the MAPK/ERK1/2 signal pathway.
Discussion
In the present study, we investigated the effects of BBR on hepatic steatosis as well as atherosclerosis and its potential mechanisms in ApoE-/- mice. The main findings were followings: (I) BBR improved lipid metabolism, presented as lowering TC, TG, and LDL-C in ApoE-/- mice fed with HFD; (II) BBR significantly reduced the formation of hepatic steatosis (liver lipid deposition) and aortic atheroma; (III) BBR could enhance the expressions of cholesterol reverse transport related genes and down-regulate expression of PCSK9, which was associated with the activation of MAPK/ERK1/2 signaling pathway. These findings were partly novel, and might improve our understandings of the beneficial effects of BBR on lipid metabolism, hepatic steatosis, and atherosclerotic progression.
Atherosclerosis is a multifactorial, long-lasting, chronic process in human, which is calculated by year. Consequently, animal models in which more rapid changes occur can be useful for the study of this process. Among these animal models, ApoE-/- mice is cheap, easily productive, and reliable by which give insight into the human process. It has been demonstrated that ApoE-/- mice show impaired clearing of plasma lipoproteins and develop atherosclerosis in a short time, and hence they are an excellent model in which to assess the impact of dietary factors, pharmacological therapy and developing new drugs (19). Previous studies have suggested that the levels of TC, TG and LDL-C were significantly higher in HFD-fed ApoE-/- mice than that in WT mice. And also, atherosclerotic plaques were obvious in the aortic arch, thoracic and abdominal aorta regions in HFD-fed ApoE-/- mice (20). In the present study, results showed that serum lipid levels including TC, LDL-C and TG in ApoE-/- mice fed with HFD were significantly higher than those fed with ND in ApoE-/- mice and WT mice. Additionally, the serum HDL-C level in ApoE-/- mice fed with HFD was lower than that in other groups. Besides, a marked formation of atheromatous plaque and significant lipid accumulation were also found in ApoE-/- mice fed with HFD, suggesting that this model is suitable tool for studying the impact of BBR on lipid-related metabolic disorders and the development of atherosclerosis.
Previous studies have suggested that BBR possesses the beneficial actions on both lipid metabolism and atherosclerosis. Data suggested that BBR exerted protective effects against atherosclerosis by modulating various pathological and physiological process, which is definitely associated with lipid modification presented as the decrease in serum TC, TG, and LDL-C levels (21). In fact, BBR could reduce serum TC, TG, and LDL-C levels in several animal models including hyperlipidemic rat, ApoE-/- mice fed FHD, ApoE-/- mice with hyperhomocysteinemia (20). In addition, the meta-analyses showed that BBR increased HDL-C concentration in patients with diabetes mellitus, hyperlipidemia and hypertension following BBR treatment (15,22). In line with previous studies, our results showed that low dose of BBR (50 mg/kg/d) and high dose of BBR (100 mg/kg/d) significantly decreased the levels of serum TC, TG and LDL-C, while increase the concentration of HDL-C in ApoE-/- mice fed with HFD, which is associated with a significant attenuation of the formation of aortic atheroma in a dose-dependent manner.
Nevertheless, several previous studies showed that the lipid improvement by BBR was related to PCSK9 (23,24). In fact, PCSK9 is a key regulator of cholesterol homeostasis that controls LDLR levels on the surface of hepatocytes and the best known function of PCSK9 is the post-translational regulation of LDLR in hepatocytes, representing the major route for LDL-C clearance from the blood circulation (25). The overall trends in vitro and in vivo findings have been in favor of a PCSK9-lowering effect for BBR, which is through enhanced LDLR density on the surface of hepatocytes (23,26). In vitro study also showed that both PCSK9 and HNF1a protein levels were decreased in BBR-treated HepG2 cells (27). The present study supported the notion that BBR significantly decreased PCSK9 expression in both ApoE-/- mice fed with HFD and HepG2 cells. However, the pathway regarding the effects of BBR on PCSK9 is not fully understood. We hypothesized that it might through the activation of the MAPK/ERK1/2 signaling pathway. The results from HepG2 cell study tested that BBR decreased PCSK9 expression by activating the ERK1/2 pathway. Moreover, in the ApoE-/- mice fed with HFD, we demonstrated that BBR increased the protein expressions of ABCA1, ABCG1 and SR-B1 in liver of ApoE-/- mice. All these proteins were related to the process of reverse cholesterol transport. Thereby, our findings might provide additional information regarding the potential role of BBR in anti-atherosclerosis process besides of its anti-inflammatory action (28,29) reducing oxidative stress (6,30,31), and enhancing cholesterol efflux (32-34), which was summarized in Figure 7.
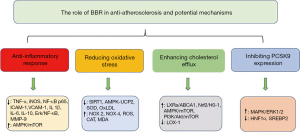
Another finding in the present study is that BBR markedly reduced hepatic steatosis, which was in agreement with previous studies. Zhu et al. demonstrated that BBR plus lifestyle intervention could more effectively reduced liver fat content than lifestyle intervention alone and exhibited a trend of toward lower liver fat content compared with pioglitazone plus lifestyle intervention in a clinical trial (35). An animal study suggested that BBR attenuated non-alcoholic hepatic steatosis through the AMPK-SREBP-1c-SCD1 pathway (36). Other investigation performed by Sun et al. indicated that BBR attenuated hepatic steatosis and enhanced energy expenditure in mice by inducing autophagy and fibroblast growth factor 21 (37). In addition, BBR improved lipid metabolism and glucogenesis in non-alcoholic fatty liver disease (NAFLD) rat model, as well as obviously attenuated the ectopic liver fat accumulation (38). In our present study, the data showed that BBR significantly reduced liver fat deposition in ApoE-/- mice fed with HFD, which may be an explanation why BBR can exert a beneficial effect on NAFLD.
Conclusions
In conclusion, the current study has showed that BBR significantly ameliorated the extent of HFD-induced atherosclerosis in ApoE-/- mice, improve the lipid profiles and hepatic fat accumulation, which appears associated with the down-regulation of PCSK9 mediated by the MAPK/ERK1/2 signal pathway. These findings may provide additional information regarding the clinical implications of BBR in the future.
Acknowledgments
Funding: This article was partly supported by Capital Health Development Fund (201614035), CAMS Major Collaborative Innovation Project (2016-I2M-1-011), and PUMC Youth Fund (3332018200).
Footnote
Reporting Checklist: The authors have completed the ARRIVE reporting checklist. Available at https://dx.doi.org/10.21037/atm-20-8106
Data Sharing Statement: Available at https://dx.doi.org/10.21037/atm-20-8106
Peer Review File: Available at https://dx.doi.org/10.21037/atm-20-8106
Conflicts of Interest: All authors have completed the ICMJE uniform disclosure form (available at https://dx.doi.org/10.21037/atm-20-8106). The authors have no conflicts of interest to declare.
Ethical Statement: The authors are accountable for all aspects of the work in ensuring that questions related to the accuracy or integrity of any part of the work are appropriately investigated and resolved. The experiments were performed under a project license (No. 0000555) granted by the Animal experimental center of Fuwai hospital, in compliance with the guidelines of the Care and Use of Laboratory Animals published by the US National Institutes of Health.
Open Access Statement: This is an Open Access article distributed in accordance with the Creative Commons Attribution-NonCommercial-NoDerivs 4.0 International License (CC BY-NC-ND 4.0), which permits the non-commercial replication and distribution of the article with the strict proviso that no changes or edits are made and the original work is properly cited (including links to both the formal publication through the relevant DOI and the license). See: https://creativecommons.org/licenses/by-nc-nd/4.0/.
References
- Ross R. Atherosclerosis--an inflammatory disease. N Engl J Med 1999;340:115-26. [Crossref] [PubMed]
- Escárcega RO, Lipinski MJ, García-Carrasco M, et al. Inflammation and atherosclerosis: Cardiovascular evaluation in patients with autoimmune diseases. Autoimmun Rev 2018;17:703-8. [Crossref] [PubMed]
- Lee GY, Kim JH, Oh GT, et al. Molecular targeting of atherosclerotic plaques by a stabilin-2-specific peptide ligand. J Control Release 2011;155:211-7. [Crossref] [PubMed]
- Kim WS, Lee YS, Cha SH, et al. Berberine improves lipid dysregulation in obesity by controlling central and peripheral AMPK activity. Am J Physiol Endocrinol Metab 2009;296:E812-9. [Crossref] [PubMed]
- Wang Y, Huang Y, Lam KS, et al. Berberine prevents hyperglycemia-induced endothelial injury and enhances vasodilatation via adenosine monophosphate-activated protein kinase and endothelial nitric oxide synthase. Cardiovasc Res 2009;82:484-92. [Crossref] [PubMed]
- Caliceti C, Rizzo P, Ferrari R, et al. Novel role of the nutraceutical bioactive compound berberine in lectin-like OxLDL receptor 1-mediated endothelial dysfunction in comparison to lovastatin. Nutr Metab Cardiovasc Dis 2017;27:552-63. [Crossref] [PubMed]
- Li TT, Wang ZB, Li Y, et al. The mechanisms of traditional Chinese medicine underlying the prevention and treatment of atherosclerosis. Chin J Nat Med 2019;17:401-12. [Crossref] [PubMed]
- Zhang J, Zhang Q, Liu G, et al. Therapeutic potentials and mechanisms of the Chinese traditional medicine Danshensu. Eur J Pharmacol 2019;864:172710 [Crossref] [PubMed]
- Kong W, Wei J, Abidi P, et al. Berberine is a novel cholesterol-lowering drug working through a unique mechanism distinct from statins. Nat Med 2004;10:1344-51. [Crossref] [PubMed]
- Wang K, Feng X, Chai L, et al. The metabolism of berberine and its contribution to the pharmacological effects. Drug Metab Rev 2017;49:139-57. [Crossref] [PubMed]
- Tarasiuk A, Pawlik L, Fichna J. Berberine as a potential therapeutic agent in the treatment of acute pancreatitis Postepy Biochem 2019;65:224-30. [Crossref] [PubMed]
- Chang W. Non-coding RNAs and Berberine: A new mechanism of its anti-diabetic activities. Eur J Pharmacol 2017;795:8-12. [Crossref] [PubMed]
- Cicero AF, Baggioni A. Berberine and Its Role in Chronic Disease. Adv Exp Med Biol 2016;928:27-45. [Crossref] [PubMed]
- Jia YJ, Xu RX, Sun J, et al. Enhanced circulating PCSK9 concentration by berberine through SREBP-2 pathway in high fat diet-fed rats. J Transl Med 2014;12:103. [Crossref] [PubMed]
- Ju J, Li J, Lin Q, et al. Efficacy and safety of berberine for dyslipidaemias: A systematic review and meta-analysis of randomized clinical trials. Phytomedicine 2018;50:25-34. [Crossref] [PubMed]
- Wei S, Zhang M, Yu Y, et al. Berberine Attenuates Development of the Hepatic Gluconeogenesis and Lipid Metabolism Disorder in Type 2 Diabetic Mice and in Palmitate-Incubated HepG2 Cells through Suppression of the HNF-4α miR122 Pathway. PLoS One 2016;11:e0152097 [Crossref] [PubMed]
- Chong BF, Murphy JE, Kupper TS, et al. E-selectin, thymus- and activation-regulated chemokine/CCL17, and intercellular adhesion molecule-1 are constitutively coexpressed in dermal microvessels: a foundation for a cutaneous immunosurveillance system. J Immunol 2004;172:1575-81. [Crossref] [PubMed]
- Lerat H, Honda M, Beard MR, et al. Steatosis and liver cancer in transgenic mice expressing the structural and nonstructural proteins of hepatitis C virus. Gastroenterology 2002;122:352-65. [Crossref] [PubMed]
- Han SG, Howatt DA, Daugherty A, et al. Atherogenic and pulmonary responses of ApoE- and LDL receptor-deficient mice to sidestream cigarette smoke. Toxicology 2012;299:133-8. [Crossref] [PubMed]
- Chang XX, Yan HM, Xu Q, et al. The effects of berberine on hyperhomocysteinemia and hyperlipidemia in rats fed with a long-term high-fat diet. Lipids Health Dis 2012;11:86. [Crossref] [PubMed]
- Li H, He C, Wang J, et al. Berberine activates peroxisome proliferator-activated receptor gamma to increase atherosclerotic plaque stability in Apoe-/- mice with hyperhomocysteinemia. J Diabetes Investig 2016;7:824-32. [Crossref] [PubMed]
- Lan J, Zhao Y, Dong F, et al. Meta-analysis of the effect and safety of berberine in the treatment of type 2 diabetes mellitus, hyperlipemia and hypertension. J Ethnopharmacol 2015;161:69-81. [Crossref] [PubMed]
- Formisano E, Pasta A, Cremonini AL, et al. Efficacy of Nutraceutical Combination of Monacolin K, Berberine, and Silymarin on Lipid Profile and PCSK9 Plasma Level in a Cohort of Hypercholesterolemic Patients. J Med Food 2020;23:658-66. [Crossref] [PubMed]
- Lupo MG, Macchi C, Marchianò S, et al. Differential effects of red yeast rice, Berberis aristata and Morus alba extracts on PCSK9 and LDL uptake. Nutr Metab Cardiovasc Dis 2019;29:1245-53. [Crossref] [PubMed]
- Momtazi AA, Banach M, Pirro M, et al. Regulation of PCSK9 by nutraceuticals. Pharmacol Res 2017;120:157-69. [Crossref] [PubMed]
- Pirillo A, Catapano AL. Berberine, a plant alkaloid with lipid- and glucose-lowering properties: From in vitro evidence to clinical studies. Atherosclerosis 2015;243:449-61. [Crossref] [PubMed]
- Dong B, Li H, Singh AB, et al. Inhibition of PCSK9 transcription by berberine involves down-regulation of hepatic HNF1α protein expression through the ubiquitin-proteasome degradation pathway. J Biol Chem 2015;290:4047-58. [Crossref] [PubMed]
- Zhao Y, Tian X, Liu G, et al. Berberine protects myocardial cells against anoxia-reoxygenation injury via p38 MAPK-mediated NF-κB signaling pathways. Exp Ther Med 2019;17:230-6. [PubMed]
- Fan X, Wang J, Hou J, et al. Berberine alleviates ox-LDL induced inflammatory factors by up-regulation of autophagy via AMPK/mTOR signaling pathway. J Transl Med 2015;13:92. [Crossref] [PubMed]
- Yousefian M, Shakour N, Hosseinzadeh H, et al. The natural phenolic compounds as modulators of NADPH oxidases in hypertension. Phytomedicine 2019;55:200-13. [Crossref] [PubMed]
- Zhu X, Yue H, Guo X, et al. The Preconditioning of Berberine Suppresses Hydrogen Peroxide-Induced Premature Senescence via Regulation of Sirtuin 1. Oxid Med Cell Longev 2017;2017:2391820 [Crossref] [PubMed]
- Lee TS, Pan CC, Peng CC, et al. Anti-atherogenic effect of berberine on LXRalpha-ABCA1-dependent cholesterol efflux in macrophages. J Cell Biochem 2010;111:104-10. [Crossref] [PubMed]
- Uitz E, Bahadori B, McCarty MF, et al. Practical strategies for modulating foam cell formation and behavior. World J Clin Cases 2014;2:497-506. [Crossref] [PubMed]
- Kou JY, Li Y, Zhong ZY, et al. Berberine-sonodynamic therapy induces autophagy and lipid unloading in macrophage. Cell Death Dis 2017;8:e2558 [Crossref] [PubMed]
- Yan HM, Xia MF, Wang Y, et al. Efficacy of Berberine in Patients with Non-Alcoholic Fatty Liver Disease. PLoS One 2015;10:e0134172 [Crossref] [PubMed]
- Zhu X, Bian H, Wang L, et al. Berberine attenuates nonalcoholic hepatic steatosis through the AMPK-SREBP-1c-SCD1 pathway. Free Radic Biol Med 2019;141:192-204. [Crossref] [PubMed]
- Sun Y, Xia M, Yan H, et al. Berberine attenuates hepatic steatosis and enhances energy expenditure in mice by inducing autophagy and fibroblast growth factor 21. Br J Pharmacol 2018;175:374-87. [Crossref] [PubMed]
- Zhao L, Cang Z, Sun H, et al. Berberine improves glucogenesis and lipid metabolism in nonalcoholic fatty liver disease. BMC Endocr Disord 2017;17:13. [Crossref] [PubMed]