Oridonin induces autophagy-mediated cell death in pancreatic cancer by activating the c-Jun N-terminal kinase pathway and inhibiting phosphoinositide 3-kinase signaling
Introduction
Pancreatic cancer (PC) is the third largest contributor to cancer deaths in the United States and has a high mortality rate (1). Despite progress in the understanding of the biology and genetic bases of PC, the prognoses of most patients have remained dire, owing to the disease’s aggressive biology and advanced stage at the time of diagnosis (2,3). Therefore, new and effective therapeutic strategies for the prevention and treatment of PC are urgently needed.
Oridonin, which is isolated from Rabdosia rubescens, is a bioactive diterpenoid that is commonly used in traditional medicines in East Asia. Oridonin has increasingly drawn attention from biomedical researchers for having numerous biological attributes, including anticancer, anti-inflammatory, antimicrobial, and neurological activity, as well as a preventive effect against hepatic fibrosis (4). Multiple proteins and pathways have been implicated in the regulation of oridonin-mediated apoptosis; these include the mechanistic target of rapamycin signaling pathway, p53, the cysteine-dependent aspartate-specific protease family, the mitogen-activated protein kinase (MAPK) family, and the nuclear factor-kappa B (NF-κB) and phosphoinositide 3-kinase (PI3K) signaling pathways (5-9).
Apoptosis is a controlled mechanistic process which ensures homeostatic maintenance of cells in normal tissues and the elimination of disordered cells. Many pathways related to apoptosis are mutated or dysregulated in human cancers (10-12). Apoptosis is characterized by a loss of viability accompanied by cytosolic vacuolization, chromatin condensation, and nuclear DNA fragmentation (13). The dysregulation of apoptotic pathways can result in cancers developing, and targeting these pathways can serve as a potential anticancer strategy, with a large number of cancer treatments already aimed at inducing the apoptotic process in malignant cells (14). Additionally, there are increasing reports that aside from promoting apoptosis, antitumor therapy can induce cell death in a variety of other ways, including by causing mitotic mutations, triggering necrosis and autophagy, and permanently arresting the cell cycle, as well as by promoting phenotypic characteristics associated with aging.
Autophagy is an evolutionarily conserved physiological process which plays a part in the routine degradation and turnover of components in the cytoplasm by lysosomal hydrolases (15,16). Autophagy, which has long been classified as a distinct form of programmed cell death to apoptosis, can be induced by a variety of stress conditions, including starvation, hypoxia, hyperthermia, and oxidative stress. It also sees massive autophagic cytoplasmic vacuolization unaccompanied by microtubule-associated protein 1A/1B-light chain (LC) 3-I conversion to LC3-II (12). Previous data have suggested that autophagy is commonly associated with cell survival; conversely, it has also been suggested that under certain conditions, autophagy contributes to cell death and can lead to apoptosis.
Previous studies revealed that oridonin could inhibit the growth of human pancreatic cancer cells by increasing apoptosis, downregulating the expression of the mRNA inflammation and inhibiting cell migration. Accumulating clinical evidence shows that in the treatment of cancer, natural extracts or traditional herbs appear to induce apoptosis and regulate autophagy (17-19). Oridonin has been shown to suppress PC cell migration and epithelial-mesenchymal transition, and induce SW1990 cell apoptosis via p38 MAPK signaling (20,21). However, to the best of our knowledge, the influence of oridonin on autophagy is unknow, the present study set out to clarify the effects of oridonin in PC.
In the current study, we investigated the antitumor effects of oridonin in an orthotopic model of PC. Oridonin administration inhibited tumor growth, and decreased the expression of Ki-67 and vascular endothelial growth factor (VEGF), which are common markers of tumor proliferation and angiogenesis. We further explored the underlying mechanism of oridonin’s effects on PC cells in vitro. Collectively, our observations show oridonin to be a promising anticancer agent for PC that induces apoptosis and autophagy via modulation of the p38 MAPK and PI3K signaling pathways.
We present the following article in accordance with the ARRIVE reporting checklist (available at https://dx.doi.org/10.21037/atm-21-2630).
Methods
Animals and cell lines
Male C57BL/6 mice (age, 6–7 weeks), supplied by the Experimental Animal Center for Military Medical Sciences, were acclimated for at least 1 week in specific pathogen-free cages before experimentation. We obtained the human PC cell line Panc-1 and mouse PC cell line Panc02 from the American Type Culture Collection (Manassas, VA, USA). The animal experimental protocol in this study was reviewed and approved by the Animal Ethical and Welfare Committee of Tianjin Medical University (approval No. TMUaMEC 2018032), in compliance with the National Institutes of Health guidelines for the care and use of animals.
Orthotopic model of PC
The PC orthotopic model was established as previously described (22). After wholly exposing the pancreatic tail, a 1-mL syringe with a 27-gauge needle was used to inject Panc02 cells (at a density of 5×105, in a 50-µL phosphate-buffered saline suspension). Subsequently, the pancreas was placed back in the abdominal cavity, which was closed by suturing (23). The mice were randomly divided into two groups with 5 mice in each group. From the day 3 after the operation, the mice were treated daily with oridonin (20 mg/kg) or phosphate-buffered saline containing 0.1% dimethyl sulfoxide (DMSO) via intraperitoneal injection for 14 days. At the end of the treatment, mice were sacrificed by decapitation.
Hematoxylin and eosin staining
Tumor tissue samples were subjected to fixing in 4% paraformaldehyde for 24 hours, followed by dehydration and paraffin embedding. From the paraffinized tissue blocks, we cut sections of 5-µm thickness before staining them with hematoxylin and eosin (H&E). After staining, the tissue sections were histopathologically examined using a light microscope.
Immunohistochemistry
Tumor tissues were subjected to fixing in 4% paraformaldehyde for 24 hours, followed by dehydration, paraffin embedding, and sectioning at intervals of 5 µm. After deparaffinizing the tissue sections and rehydrating them with xylene and alcohol at graded concentrations (95%, 85%, 75%, 50%), we performed antigen retrieval by microwaving the sections with 5 mmol/L citrate buffer (pH 6.0) for 15 minutes. The sections were firstly incubated for 15 minutes in 3% H2O2 to block endogenous peroxidase activity. After that, we subjected them to blocking with 5% goat serum, followed by incubation with antibodies (Ki-67 and VEGF) at 4 °C overnight. Finally, after 60 minutes’ incubation with a horseradish peroxidase (HRP)-conjugated secondary antibody at room temperature, the sections were treated with a 3,3’-diaminobenzidine solution (ZSGB-BIO, China).
Chemicals and reagents
The oridonin used in this study was obtained from Hao Xuan Biotechnology Co., Ltd. (China) and was determined by high-performance liquid chromatography to have a purity of 98%. To prepare a stock solution, we dissolved oridonin (10 mmol/L) in DMSO, and kept the solution at –20 °C until experimentation. Oridonin was again diluted with culture medium to obtain the final concentration as described previously (0, 5, 10, 20, 40, and 80 µM). Dulbecco’s Modified Eagle Medium and fetal bovine serum were purchased from Gibco.
MTT (3-[4,5-dimethylthiazol-2-yl]-2,5-diphenyltetrazolium bromide) and the Annexin V-FITC/PI staining apoptosis detection kit were purchased from Sungene Biotech (China). Selleck Inc. (Houston, TX, USA) supplied the 3-Methyladenine (3-MA) used. Lipofectamine 3000 was obtained from Invitrogen (Carlsbad, CA, USA). Antibodies against p62, Beclin1, LC3, p38 MAPK, phosphorylated p38 MAPK (p-p38 MAPK), c-Jun N-terminal kinase (JNK), phosphorylated JNK (p-JNK), extracellular regulated kinase (Erk1/2), phosphorylated Erk1/2 (p-Erk1/2), PI3K, Ki-67, VEGF, and β-actin, along with glyceraldehyde 3-phosphate dehydrogenase and HRP-conjugated secondary antibodies, were obtained from Cell Signaling Technology, Inc. (USA).
Cell culture and treatments
Cell cultures were carried out using Dulbecco’s Modified Eagle Medium (HyClone, Beijing, China) containing 10% fetal bovine serum (HyClone) at 37 °C with 5% CO2. There were 3 cell groups: (I) the oridonin group: 24-hour treatment with 20 µM oridonin; (II) the 3-MA group: 24-hour treatment with 20 µM oridonin and 10 µM 3-MA; (III) the control group: no treatment.
Cell viability analysis
The effects of oridonin on Panc-1 and Panc02 cell viability were determined using the MTT assay. Cells were plated in 96-well plates (5×104 cells/well) and incubated for 24 hours at 37 °C, after which they were treated with 0, 5, 10, 20, 40, or 80 µM oridonin for 24 hours. After the addition of new medium and 20 µL of MTT solution (5 mg/mL) to the individual wells, the cells were incubated for 4 hours at 37 °C with 5% CO2. Following that, the medium was discarded, the addition of 200 µL of DMSO was made to each of the wells, and the absorbance was measured.
Assessment of apoptosis using Annexin V-FITC/PI staining
The Annexin V-FITC/PI apoptosis assay kit (Sungene Biotech, China) and flow cytometry were employed to analyze apoptosis. Annexin V has a high affinity for phosphatidylserine (PS), which is located in the cell membrane and becomes exposed in the early stage of apoptosis. Flow cytometry was performed with NovoExpress (ACEA Biosciences, USA) according to the ACEA NovoExpress Software Guide. Annexin V+PI– cells were those in the early stage of apoptosis, and Annexin V+PI+ cells were those in the late stage of apoptosis. In our study, the rate of cell apoptosis was represented as the percentage of early apoptotic + late apoptotic cells.
Western blot analysis
Panc-1 and Panc02 cells were incubated with various concentrations of oridonin formononetin (0, 10, 20, and 40 µM) for 24 hours. After cell harvesting, cold radioimmunoprecipitation buffer (Millipore, USA) containing phosphatase and protease inhibitors, phenylmethanesulfonyl fluoride, and aprotinin (Sigma, USA) was used for cell lysis for 30 minutes. The supernatant was collected by centrifuging lysates for 10 minutes at 12,000 ×g and 4 °C. A bicinchoninic acid protein assay kit was used to determine the concentration of total protein. A total of 30 mg of protein per well was separated by electrophoresis before transfer to a polyvinylidene fluoride (PVDF) membrane (Millipore, USA). Tris-buffered saline (TBS) with 0.1% Tween-20 and 5% nonfat milk was used to block the membrane for 2 hours.
The membrane was subjected to further incubation with primary antibodies against different proteins at 4 °C overnight. After being washed 3 times with TBS with Tween-20, the membrane was subjected to subsequent incubation with the appropriate HRP-conjugated secondary antibody at room temperature for 1 hour. Electrochemiluminescence detection reagents (Beyotime, China) were then added to develop the bands. Protein expression levels were calculated on the basis of the relative band intensities, with β-actin or glyceraldehyde 3-phosphate dehydrogenase serving as a control.
Cell transfection and fluorescence microscopy
Lipofectamine 3000™ was used to transfect the Panc-1 and Panc02 cell lines with the green fluorescent protein (GFP)-LC3 plasmid in line with the protocol supplied by the manufacturer. Following treatment in the presence or absence of 20 µM oridonin and 10 µM 3-MA, cellular GFP-LC3 puncta formation was analyzed by fluorescence microscopy. The formation of at least 5 countable puncta was interpreted as autophagosomal accumulation.
Statistical analysis
Experiments were repeated 3 times. Student’s t test was used for comparisons between two groups, and one-way analysis of variance (ANOVA) was used to compare multiple groups. In the analysis of differences among multiple groups, Tukey’s Honestly Significant Difference test was applied following one-way ANOVA. In all figures, error bars indicate standard deviations. The intensity of Ki-67 and VEGF expression was quantitated using the NIH Image J software (http://rsb.info.nih.gov/ij/). SPSS 19.0 and GraphPad Prism Version 5.0 software were employed for all statistical analyses.
Results
Oridonin attenuates orthotopic PC in mice
In our investigation of the antitumor effects of oridonin, we developed an orthotopic C57BL/6 mouse model of PC. Compared with the control group, tumor formation was significantly suppressed in mice pretreated with oridonin (Figure 1A,B,C) (P<0.05). The H&E staining results revealed a larger number of apoptosis- and necrosis-associated pathologic changes in tumor tissues from the oridonin group compared to control tissues (Figure 1D). Immunohistological staining revealed the levels of Ki-67 and VEGF, which serve as indicators of cancer proliferation and angiogenesis, to be decreased in the oridonin treatment group (Figure 1E).
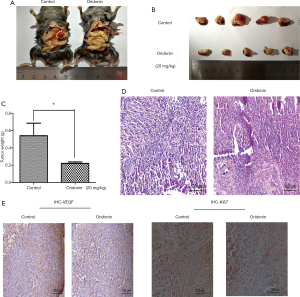
Oridonin inhibits the viability of Panc-1 and Panc02 cells
We further examined the effects of oridonin in PC by exposing the Panc-1 and Panc02 cell lines to different concentrations of oridonin (0, 5, 10, 20, 40, and 80 µM) for 24 hours. Cell viability was determined using an MTT assay. As shown in Figure 2, oridonin inhibited cell growth in a dose-dependent manner. In the oridonin treatment group, we observed significant suppression of cells’ proliferation abilities as well as a decrease in the proliferation index, compared with the control group. The viability of Panc-1 and Panc02 cells in the oridonin group was significantly decreased at a concentration of 5 µM (P<0.05, Figure 2A,B). Also, the IC50 values of Panc-1 and Panc02 cells were both approximately 20 µM.
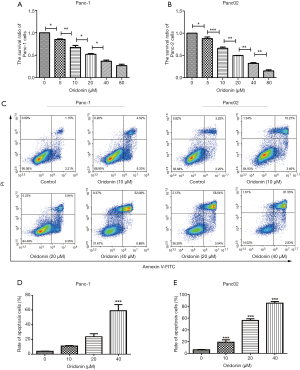
Effects of oridonin on Panc-1 and Panc02 cell apoptosis
We exposed Panc-1 and Panc02 cells to 0, 10, 20, or 40 µM oridonin for 24 hours, and the results can be seen in Figure 2C, D, and E. While Panc02 cell apoptosis was significantly decreased in the 10 µM oridonin group (P<0.001), only a high concentration of oridonin (40 µM) significantly induced Panc-1 cell apoptosis (P<0.001).
Effects of oridonin on autophagy activation in Panc-1 and Panc02 cells
Because autophagy is a contributor to cell death, our next experiment aimed to establish whether oridonin can induce PC cell autophagy. In an attempt to uncover the alternative mechanism underlying oridonin-caused cell death, cells were treated with different concentrations of oridonin (0, 10, 20, or 40 µM) for 24 hours, and the expression of the autophagy-related proteins Beclin-1, LC3, and p62 was examined through western blot. Oridonin administration elevated the quantity of LC3B II protein and led to the upregulation of P62 and Beclin-1 expression (Figure 3A,B). Next, oridonin-induced autophagy in PC cells was blocked using 3-MA, which is the most widely used drug for inhibiting autophagy. The results suggested that oridonin-induced cellular autophagic activity in PC cells may exert a protective effect (Figure 3C,D). Autophagy characteristically sees an increase in acidic vesicular organelles, with a correlative increase in autophagosomes (24). To further explore oridonin-induced autophagy, an Ad-GFP-RFP-LC3 assay was carried out. LC3 localization during autophagosome formation was examined by assessing GFP-LC3 expression in Panc-1 and Panc02 cells. The cells were subjected to transfection with LC3-GFP plasmids and treatment with 20 µM oridonin with or without 3-MA for 24 hours. We found that oridonin induced significant increases in GFP-LC3 puncta formation in both Panc-1 and Panc02 cells (Figure 3E,F) (P<0.001).
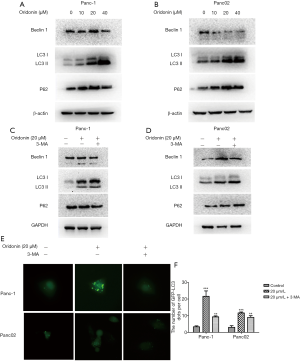
Oridonin mediates autophagy in PC cells via activating the JNK signaling pathway and inhibiting the PI3K pathway
MAPKs play a vital role in antiproliferative events (25). A number of studies have demonstrated that oridonin can inhibit the PI3K pathway, which contributes to the growth, migration, and invasion of various cancers (26-28). To elucidate the involvement of the p38 MAPK and PI3K pathways in oridonin-induced autophagy, we analyzed the expression levels of p-JNK, JNK, p-Erk1/2, Erk1/2, and PI3K after treatment with different concentrations of oridonin (20 and 40 µM) for 24 hours.
As shown in Figure 4A and B, after 24 hours of oridonin stimulation, dose-dependent increases in the phosphorylation levels of JNK and Erk1/2 were detected in both Panc-1 and Panc02 cells. Moreover, oridonin led to decreases in the protein expression of PI3K in both Panc-1 and Panc02 cells. Taken together, these results indicate that oridonin can inhibit PC cell proliferation, promote tumor apoptosis and autophagy, and increase the protein expression of p-JNK, p-Erk, and p-p38MAPK, while reducing the protein expression of PI3K. Thus, we speculate that the effects of oridonin on the proliferation, apoptosis, and autophagy of PC cells are mediated via simultaneous inhibition of the PI3K pathway and activation of the JNK pathway (Figure 5).
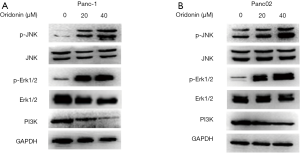
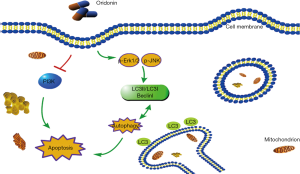
Discussion
PC is a kind of cancer with high malignant degree and mortality. Although surgery is the main means to achieve cure, the whole process management of resectable pancreatic cancer is still a long-term puzzle for clinicians due to the particularity of anatomical location and tumor microenvironment (TME) (29,30). Natural products have proved to be rich sources for drug discovery. Research to identify natural products that are capable of inducing tumor cell apoptosis is developing rapidly, and so far, many monomeric compounds in natural drugs have been shown to have this ability (31). In view of the fact that PC has a dense interstitial TME, few interstitial blood vessels, and high hydrostatic pressure, lymphocytes are not easy to migrate around cancer cells to play a role, and drug therapy cannot get the proper part. These comprehensive effects promote the occurrence of tumors and the progress of diseases (32,33). Oridonin has attracted increasing attention in the in vivo and in vitro study of various cancers for its antitumor activity in cellular processes including proliferation, cell cycle arrest, and apoptosis (34-36). However, the mechanism of oridonin’s inhibitory effect on PC progression has yet to be fully explained.
The results of the present research indicate that the apoptosis caused by oridonin has the effect of suppressing PC cell viability; thus, it is a natural monomeric compound that potentially holds promise for cancer therapy. In our orthotopic PC mouse model, we observed that oridonin restricted tumor growth, which was in line with our in vitro experimental findings. Moreover, we found that some of the mechanisms of the antitumor effects of oridonin are related apoptosis and autophagy, and involve signaling pathways.
Apoptosis is a gene-controlled, active cell death process, the central characteristic of which is the degradation of intracellular components by caspases or cysteine proteolytic enzymes (37). Studying the occurrence and regulation of apoptosis can aid in explaining the many biological phenomena and causes of disease (38,39). Annexin V, a calcium-dependent phospholipid-binding protein, serves as a helpful marker of cells with exposed phosphatidylserine, when labeled with detectable tags (40). Thus, during cancer treatment, Annexin V serves as a predictor of tumor cell necrosis (41). In our study, Panc-1 and Panc02 cells were treated with 0, 10, 20, or 40 µM oridonin for 24 hours. The proportion of Annexin V+PI+ Panc02 cells was found to be significantly increased, and that of Annexin V+PI+ Panc-1 cells was also significantly increased by treatment with 40 µM oridonin. The H&E results revealed tumor tissues from oridonin-treated mice to display an increased number of apoptosis- and necrosis-related pathologic changes (e.g., nuclei of very light blue or without any blue, and smaller cells, with shrinkage and deformities) compared to those from untreated mice. Immunohistological staining also revealed diminished levels of Ki-67 and VEGF in the oridonin treatment group. Together, these in vivo and in vitro experimental results showed that oridonin induces cell apoptosis and inhibits tumor growth in PC. However, the mechanism of the antitumor effects of oridonin remained unclear; thus, we next explored this mechanism.
Initially, autophagy and apoptosis were believed to differ in terms of their morphology, biochemical indicators, and related molecules and mechanisms. However, overwhelming evidence uncovered that the two processes can antagonize or promote each other, and can occur successively or simultaneously in the same cell. The same inducing factors of autophagy and apoptosis can be found in different cell lines; the molecules involved in autophagy and apoptosis may also be cross-linked, and these molecules play positive or negative roles in autophagy and apoptosis (42). Autophagy plays important physiological roles in cancers, and its dysfunction has been linked to various diseases. A growing body of evidence shows that autophagy plays a dual role in cancer therapy by protecting cell survival or contributing to cell death (43). LC3I conversion to LC3II plays a role in forming autophagosomes; therefore, LC3II typically serves as an autophagic marker (44). We found oridonin-induced PC cell autophagy to be reflected in a rise in the quantity of GFP-LC3 puncta and the enhancement of LC3B-I conversion to LC3B-II compared to the control group. This observation indicated that oridonin induces apoptosis and autophagy in PC cell lines.
Autophagy also depends on the involvement of a series of autophagy-related proteins (Atgs); thus far, at least 37 Atgs have been identified in mammalian cells (2). Beclin1, the mammalian ortholog of yeast Atg6, is a key player in inducing autophagy and autophagosome formation. In this study Beclin1 activation was detected in Panc-1 cells. p62, also known as SQSTM1, is expressed in a variety of cells and tissues and can be used as a scaffold protein in a variety of signal transduction processes. It binds to LC3 and ubiquitinated substrates, which are then integrated into autophagosomes and degraded in autophagolysosomes. Thus, the protein expression of p62 can be used as an indicator of changes in autophagic ability (45).
Our data showed that at a high concentration such as 40 µM, oridonin could ameliorate the downregulation of p62 and further promote the upregulation of LC3-II/I. These effects are attributable to high concentrations of oridonin effectively blocking autophagy at the lysosomal degradation step (46). Autophagic flux detection is one of the most reliable indicators of autophagic activity. One study demonstrated that in combination with autophagy inhibitors, the detection of the LC3II/I ratio is the gold standard for autophagic flux analysis (47). Oridonin was observed to enhance the conversion of LC3B-I to LC3B-II and increase the expression of Beclin-1. The expression of p62 was inconsistent between the two cell lines, which may be due to discrepancies between the cell lines caused by differences in genus and tissue sources. However, considering all the results, this inconsistency does not affect the final conclusion. Similarly, cells in PC tissue had significantly increased levels of LC3-II (the autophagosome-bound form of LC3) and increased autophagic flux, indicating that autophagy was actively promoted in these tumors/cell lines in response to oridonin treatment. These results indicate that autophagy activation may be an important mechanism for the antitumor effects of oridonin.
The involvement of MAPKs in multiple physiological and pathological processes has been evidenced by a growing bank of research (48). JNK, a stress-responsive kinase, is closely related to cell growth, differentiation, apoptosis, tumorigenesis, and other signaling pathways (49,50). At the molecular level, oridonin is a bioactive diterpenoid isolated from Rabdosia rubescens. It activates MAPK signaling pathways, which have critical regulatory involvement in cell growth, apoptosis, and autophagy (51). There have been few studies on the mechanism of tumor apoptosis induced by monomers in traditional Chinese medicines. Previous research has shown that vitexin inhibits hepatocellular carcinoma growth by inducing apoptosis and suppressing autophagy, both of which occur via the JNK/MAPK pathway (52). In the present study, we observed that oridonin could increase the phosphorylation levels of JNK and Erk1/2 in PC cells, and inhibit the protein expression of PI3K. These results are consistent with those of previous studies (53-57).
Autophagy induced by chemotherapy drugs can not only promote the apoptosis of tumor cells, but also serve as the survival mechanism of anti-apoptosis of tumor cells (58). Radiotherapy can activate autophagy of pancreatic cancer cells and induce autophagy apoptosis (59). Gemcitabine combined with radiotherapy has a synergistic effect in inducing autophagy. After using drugs to inhibit autophagy, it is necessary to increase the dose of gemcitabine to achieve the previous tumor inhibition level, which also indicates that inducing autophagy can inhibits pancreatic cancer cells (60). The mechanism of 5-fu combined with omeprazole in promoting apoptosis of pancreatic cancer cells also is to induce autophagy (61). The latest research shows that after inhibiting the corresponding signal molecules in Kras/ERK signal pathway, the energy metabolism and mitochondrial function of tumor cells were inhibited, and the autophagy of tumor cells was obviously enhanced. Because the products of autophagy catabolism can provide necessary nutrients to maintain the normal metabolism of tumors, the treatment methods targeting this signal pathway cannot obviously inhibit the growth of tumor cells (62). Generally speaking, under the intervention of chemotherapy, radiotherapy and other treatment measures, the autophagy becomes more complex because of the activation of a certain regulatory mechanism, which can not only promote the growth of tumor, but also inhibit the killing of tumor cells. This may be caused by different ways and mechanisms of inducing autophagy, and may also be determined by related gene states. In the present study, the in vivo results showed that oridonin inhibited the growth of PC in an orthotopic mouse model, and we further probed the mechanism of the antitumor effects of oridonin. Taken together, our data show that oridonin induces apoptosis and autophagy through activating the JNK pathway and inhibiting the PI3K pathway.
In summary, our study is the first to evidence the antitumor and autophagy-activating effects of oridonin in PC cells in vitro and in vivo, and the molecular mechanisms potentially underlying them. We propose that the effects of oridonin on PC cell apoptosis and autophagy are mediated through its simultaneous inhibition of the PI3K pathway and activation of the JNK pathway.
Acknowledgments
Funding: This work was supported by funding from the National Natural Science Foundation of China (grant No. 81602496), the Natural Science Foundation of Tianjin (grant Nos. 18JCQNJC111000 and 18JCQNJC13400), and the Joint Construction Project of Medical Science and Technology of Henan Province (grant No. LHGJ20190635).
Footnote
Reporting Checklist: The authors have completed the ARRIVE reporting checklist. Available at https://dx.doi.org/10.21037/atm-21-2630
Data Sharing Statement: Available at https://dx.doi.org/10.21037/atm-21-2630
Conflicts of Interest: All authors have completed the ICMJE uniform disclosure form (available at https://dx.doi.org/10.21037/atm-21-2630). The authors have no conflicts of interest to declare.
Ethical Statement: The authors are accountable for all aspects of the work in ensuring that questions related to the accuracy or integrity of any part of the work are appropriately investigated and resolved. The animal experimental protocol in this study was reviewed and approved by the Animal Ethical and Welfare Committee of Tianjin Medical University (approval No. TMUaMEC 2018032), in compliance with the National Institutes of Health guidelines for the care and use of animals.
Open Access Statement: This is an Open Access article distributed in accordance with the Creative Commons Attribution-NonCommercial-NoDerivs 4.0 International License (CC BY-NC-ND 4.0), which permits the non-commercial replication and distribution of the article with the strict proviso that no changes or edits are made and the original work is properly cited (including links to both the formal publication through the relevant DOI and the license). See: https://creativecommons.org/licenses/by-nc-nd/4.0/.
References
- Siegel RL, Miller KD, Jemal A. Cancer Statistics, 2017. CA Cancer J Clin 2017;67:7-30. [Crossref] [PubMed]
- Paquette M, El-Houjeiri L, Pause A. mTOR Pathways in Cancer and Autophagy. Cancers (Basel) 2018;10:18. [Crossref] [PubMed]
- Zhang Q, Zeng L, Chen Y, et al. Pancreatic Cancer Epidemiology, Detection, and Management. Gastroenterol Res Pract 2016;2016:8962321 [Crossref] [PubMed]
- Xu J, Wold EA, Ding Y, et al. Therapeutic Potential of Oridonin and Its Analogs: From Anticancer and Antiinflammation to Neuroprotection. Molecules 2018;23:474. [Crossref] [PubMed]
- Wang YY, Lv YF, Lu L, et al. Oridonin inhibits mTOR signaling and the growth of lung cancer tumors. Anticancer Drugs 2014;25:1192-200. [Crossref] [PubMed]
- Shi M, Lu XJ, Zhang J, et al. Oridonin, a novel lysine acetyltransferases inhibitor, inhibits proliferation and induces apoptosis in gastric cancer cells through p53- and caspase-3-mediated mechanisms. Oncotarget 2016;7:22623-31. [Crossref] [PubMed]
- Oh HN, Seo JH, Lee MH, et al. Oridonin induces apoptosis in oral squamous cell carcinoma probably through the generation of reactive oxygen species and the p38/JNK MAPK pathway. Int J Oncol 2018;52:1749-59. [Crossref] [PubMed]
- Cheng Y, Qiu F, Ye YC, et al. Autophagy inhibits reactive oxygen species-mediated apoptosis via activating p38-nuclear factor-kappa B survival pathways in oridonin-treated murine fibrosarcoma L929 cells. FEBS J 2009;276:1291-306. [Crossref] [PubMed]
- Qing K, Jin Z, Fu W, et al. Synergistic effect of oridonin and a PI3K/mTOR inhibitor on the non-germinal center B cell-like subtype of diffuse large B cell lymphoma. J Hematol Oncol 2016;9:72. [Crossref] [PubMed]
- Steller H. Mechanisms and genes of cellular suicide. Science 1995;267:1445-9. [Crossref] [PubMed]
- Kim JH, Bae YC, Lee JW, et al. Effects of ginsenoside Rg3 on apoptosis in A375.S2 melanoma cells. Transl Cancer Res 2019;8:357-66. [Crossref]
- Portugal J, Bataller M, Mansilla S. Cell death pathways in response to antitumor therapy. Tumori 2009;95:409-21. [Crossref] [PubMed]
- White E. Life, death, and the pursuit of apoptosis. Genes Dev 1996;10:1-15. [Crossref] [PubMed]
- Thompson CB. Apoptosis in the pathogenesis and treatment of disease. Science 1995;267:1456-62. [Crossref] [PubMed]
- Wang CY, Ma S, Bi SJ, et al. Enhancing autophagy protects platelets in immune thrombocytopenia patients. Ann Transl Med 2019;7:134. [Crossref] [PubMed]
- Vicencio JM, Galluzzi L, Tajeddine N, et al. Senescence, apoptosis or autophagy? When a damaged cell must decide its path--a mini-review. Gerontology 2008;54:92-9. [Crossref] [PubMed]
- Sun Y. The role of Chinese medicine in clinical oncology. Chin J Integr Med 2014;20:3-10. [Crossref] [PubMed]
- Chen KC, Sun MF, Chen HY, et al. Potential smoothened inhibitor from traditional Chinese medicine against the disease of diabetes, obesity, and cancer. Biomed Res Int 2014;2014:873010 [Crossref] [PubMed]
- Guerin M, Qian C, Zhong Q, et al. Translational oncology toward benefiting cancer patients: the Sun Yat-sen University Cancer Center experience. Sci China Life Sci 2016;59:1057-62. [Crossref] [PubMed]
- Liu QQ, Chen K, Ye Q, et al. Oridonin inhibits pancreatic cancer cell migration and epithelial-mesenchymal transition by suppressing Wnt/β-catenin signaling pathway. Cancer Cell Int 2016;16:57. [Crossref] [PubMed]
- Bu HQ, Liu DL, Wei WT, et al. Oridonin induces apoptosis in SW1990 pancreatic cancer cells via p53- and caspase-dependent induction of p38 MAPK. Oncol Rep 2014;31:975-82. [Crossref] [PubMed]
- Jiang YJ, Lee CL, Wang Q, et al. Establishment of an orthotopic pancreatic cancer mouse model: cells suspended and injected in Matrigel. World J Gastroenterol 2014;20:9476-85. [Crossref] [PubMed]
- Wang B, Zheng X, Liu J, et al. Osthole inhibits pancreatic cancer progression by directly exerting negative effects on cancer cells and attenuating tumor-infiltrating M2 macrophages. J Pharmacol Sci 2018;137:290-8. [Crossref] [PubMed]
- Zhou H, Shen T, Shang C, et al. Ciclopirox induces autophagy through reactive oxygen species-mediated activation of JNK signaling pathway. Oncotarget 2014;5:10140-50. [Crossref] [PubMed]
- Oseini AM, Roberts LR. PDGFRalpha: a new therapeutic target in the treatment of hepatocellular carcinoma? Expert Opin Ther Targets 2009;13:443-54. [Crossref] [PubMed]
- Sun B, Wang G, Liu H, et al. Oridonin inhibits aberrant AKT activation in breast cancer. Oncotarget 2018;9:23878-89. [Crossref] [PubMed]
- Yang J, Ren X, Zhang L, et al. Oridonin inhibits oral cancer growth and PI3K/Akt signaling pathway. Biomed Pharmacother 2018;100:226-32. [Crossref] [PubMed]
- Li CY, Wang Q, Shen S, et al. Oridonin inhibits migration, invasion, adhesion and TGF-β1-induced epithelial-mesenchymal transition of melanoma cells by inhibiting the activity of PI3K/Akt/GSK-3β signaling pathway. Oncol Lett 2018;15:1362-72. [PubMed]
- Kleeff J, Korc M, Apte M, et al. Pancreatic cancer. Nat Rev Dis Primers 2016;2:16022. [Crossref] [PubMed]
- Wolfgang CL, Herman JM, Laheru DA, et al. Recent progress in pancreatic cancer. CA Cancer J Clin 2013;63:318-48. [Crossref] [PubMed]
- Samuelsen JT, Dahl JE, Karlsson S, et al. Apoptosis induced by the monomers HEMA and TEGDMA involves formation of ROS and differential activation of the MAP-kinases p38, JNK and ERK. Dent Mater 2007;23:34-9. [Crossref] [PubMed]
- Jurcak N, Zheng L. Signaling in the microenvironment of pancreatic cancer: Transmitting along the nerve. Pharmacol Ther 2019;200:126-34. [Crossref] [PubMed]
- Rucki AA, Foley K, Zhang P, et al. Heterogeneous Stromal Signaling within the Tumor Microenvironment Controls the Metastasis of Pancreatic Cancer. Cancer Res 2017;77:41-52. [Crossref] [PubMed]
- Yao Z, Wang L, Wu X, et al. Enhanced Procoagulant Activity on Blood Cells after Acute Ischemic Stroke. Transl Stroke Res 2017;8:83-91. [Crossref] [PubMed]
- Xia R, Chen SX, Qin Q, et al. Oridonin Suppresses Proliferation of Human Ovarian Cancer Cells via Blockage of mTOR Signaling. Asian Pac J Cancer Prev 2016;17:667-71. [Crossref] [PubMed]
- Liu DL, Bu HQ, Jin HM, et al. Enhancement of the effects of gemcitabine against pancreatic cancer by oridonin via the mitochondrial caspase-dependent signaling pathway. Mol Med Rep 2014;10:3027-34. [Crossref] [PubMed]
- Musumeci G, Castrogiovanni P, Trovato FM, et al. Biomarkers of Chondrocyte Apoptosis and Autophagy in Osteoarthritis. Int J Mol Sci 2015;16:20560-75. [Crossref] [PubMed]
- Ashkenazi A. Targeting death and decoy receptors of the tumour-necrosis factor superfamily. Nat Rev Cancer 2002;2:420-30. [Crossref] [PubMed]
- Evan GI, Vousden KH. Proliferation, cell cycle and apoptosis in cancer. Nature 2001;411:342-8. [Crossref] [PubMed]
- Vermes I, Haanen C, Steffens-Nakken H, et al. A novel assay for apoptosis. Flow cytometric detection of phosphatidylserine expression on early apoptotic cells using fluorescein labelled Annexin V. J Immunol Methods 1995;184:39-51. [Crossref] [PubMed]
- Verheij M. Clinical biomarkers and imaging for radiotherapy-induced cell death. Cancer Metastasis Rev 2008;27:471-80. [Crossref] [PubMed]
- Mukhopadhyay S, Panda PK, Sinha N, et al. Autophagy and apoptosis: where do they meet? Apoptosis 2014;19:555-66. [Crossref] [PubMed]
- Wu Y, Wang X, Guo H, et al. Synthesis and screening of 3-MA derivatives for autophagy inhibitors. Autophagy 2013;9:595-603. [Crossref] [PubMed]
- Giménez-Xavier P, Francisco R, Santidrián AF, et al. Effects of dopamine on LC3-II activation as a marker of autophagy in a neuroblastoma cell model. Neurotoxicology 2009;30:658-65. [Crossref] [PubMed]
- Pankiv S, Clausen TH, Lamark T, et al. p62/SQSTM1 binds directly to Atg8/LC3 to facilitate degradation of ubiquitinated protein aggregates by autophagy. J Biol Chem 2007;282:24131-45. [Crossref] [PubMed]
- Grimaldi A, Santini D, Zappavigna S, et al. Antagonistic effects of chloroquine on autophagy occurrence potentiate the anticancer effects of everolimus on renal cancer cells. Cancer Biol Ther 2015;16:567-79. [Crossref] [PubMed]
- Tanida I, Minematsu-Ikeguchi N, Ueno T, et al. Lysosomal turnover, but not a cellular level, of endogenous LC3 is a marker for autophagy. Autophagy 2005;1:84-91. [Crossref] [PubMed]
- Hung AC, Tsai CH, Hou MF, et al. The synthetic β-nitrostyrene derivative CYT-Rx20 induces breast cancer cell death and autophagy via ROS-mediated MEK/ERK pathway. Cancer Lett 2016;371:251-61. [Crossref] [PubMed]
- Ebelt ND, Cantrell MA, Van Den Berg CL. c-Jun N-Terminal Kinases Mediate a Wide Range of Targets in the Metastatic Cascade. Genes Cancer 2013;4:378-87. [Crossref] [PubMed]
- Mu L, Sui W, Lin Y, et al. Androgen attenuates antitumor effects of gastric cancer cells by bone marrow mesenchymal stem cells via restricting the JNK signaling activation. Transl Cancer Res 2019;8:917-27. [Crossref]
- Yu Y, Fan SM, Ye YC, et al. The tyrphostin AG1478 augments oridonin-induced A431 cell apoptosis by blockage of JNK MAPK and enhancement of oxidative stress. Free Radic Res 2012;46:1393-405. [Crossref] [PubMed]
- He JD, Wang Z, Li SP, et al. Vitexin suppresses autophagy to induce apoptosis in hepatocellular carcinoma via activation of the JNK signaling pathway. Oncotarget 2016;7:84520-32. [Crossref] [PubMed]
- Burmi RS, Maginn EN, Gabra H, et al. Combined inhibition of the PI3K/mTOR/MEK pathway induces Bim/Mcl-1-regulated apoptosis in pancreatic cancer cells. Cancer Biol Ther 2019;20:21-30. [Crossref] [PubMed]
- Xue R, Meng Q, Lu D, et al. Mitofusin2 Induces Cell Autophagy of Pancreatic Cancer through Inhibiting the PI3K/Akt/mTOR Signaling Pathway. Oxid Med Cell Longev 2018;2018:2798070 [Crossref] [PubMed]
- Mirzoeva OK, Hann B, Hom YK, et al. Autophagy suppression promotes apoptotic cell death in response to inhibition of the PI3K-mTOR pathway in pancreatic adenocarcinoma. J Mol Med (Berl) 2011;89:877-89. [Crossref] [PubMed]
- Lin HW, Hsieh MJ, Yeh CB, et al. Coronarin D induces apoptotic cell death through the JNK pathway in human hepatocellular carcinoma. Environ Toxicol 2018;33:946-54. [Crossref] [PubMed]
- Almasi S, Kennedy BE, El-Aghil M, et al. TRPM2 channel-mediated regulation of autophagy maintains mitochondrial function and promotes gastric cancer cell survival via the JNK-signaling pathway. J Biol Chem 2018;293:3637-50. [Crossref] [PubMed]
- Görgülü K, Diakopoulos KN, Kaya-Aksoy E, et al. The Role of Autophagy in Pancreatic Cancer: From Bench to the Dark Bedside. Cells 2020;9:1063. [Crossref] [PubMed]
- Mukubou H, Tsujimura T, Sasaki R, et al. The role of autophagy in the treatment of pancreatic cancer with gemcitabine and ionizing radiation. Int J Oncol 2010;37:821-8. [PubMed]
- Pardo R, Lo Ré A, Archange C, et al. Gemcitabine induces the VMP1-mediated autophagy pathway to promote apoptotic death in human pancreatic cancer cells. Pancreatology 2010;10:19-26. [Crossref] [PubMed]
- Udelnow A, Kreyes A, Ellinger S, et al. Omeprazole inhibits proliferation and modulates autophagy in pancreatic cancer cells. PLoS One 2011;6:e20143 [Crossref] [PubMed]
- Bryant KL, Stalnecker CA, Zeitouni D, et al. Combination of ERK and autophagy inhibition as a treatment approach for pancreatic cancer. Nat Med 2019;25:628-40. [Crossref] [PubMed]