HIF-1α regulated tongue squamous cell carcinoma cell growth via regulating VEGF expression in a xenograft model
Introduction
Tongue squamous cell carcinoma (TSCC) is the most common type of the oral cancers (1). And its incidence is prone to increase recently. In the past several decades, many kinds of therapies such as operation, chemo- and radio-therapy were used for treating TSCC patients, but the prognosis was not satisfactory, the five-year survival rate is no more than 50% (2). Therefore, it is important to find out new special bio-markers, which are closely related to bionomic characteristics, prognosis, and its mechanisms that regulate tumorigenesis and tumor growth in tongue squamous cell carcinoma cells.
Hypoxia-inducible factor-1 (HIF-1) is a heterodimer consisting of hypoxia-inducible factor-1 alpha (HIF-1α) and HIF-1β (3). HIF-1α is an important factor contributed to the tumor aggressiveness, metastasis and mortality by controlling oncogenic and tumor suppressor gene pathways (4-6). And it regulates the expression of many genes involved in adaptive responses to oxygen deprivation and stimulates the development of new blood vessels by releasing various angiogenic-stimulating cytokines and growth factors (7-9). Vascular endothelial growth factor (VEGF) is a significant mediator in the vascularization, proliferation, invasion and metastasis of tumor, and its receptors play an important role in tumor angiogenesis (10,11). Sometimes, VEGF may be secreted from cells under hypoxia conditions and bind to its receptors on endothelial cells, then activate endothelial cell division and angiogenesis (12).
In recent years, the roles that HIF-1α and VEGF play in tumorigenesis have been given special attention. HIF-1α and VEGF expression was up-regulated in a variety of tumors. In addition, HIF-1α can stimulate the expression of VEGF. Some questions about the roles of these proteins in TSCC have not yet been explored: how does their expression affect the growth of TSCC? What is their relationship in TSCC? And what is the specific mechanism responsible for those effects? In this study, we first transfected HIF-1α specific siRNA in TSCC cell lines in vitro and investigated the expression of HIF-1α and VEGF. And then, we evaluated the role of HIF-1α in tumor formation in nude mice inoculated subcutaneously with TSCC cells.
Materials and methods
Cell culture
The human tongue cancer cell lines (CAL-27), obtained from the Ninth People’s Hospital, School of Medicine, Shanghai Jiao Tong University, Shanghai, China were thawed and maintained in DMEM a high glucose culture media (Gibco USA) with 10% fetal bovine serum (FBS) (Gibco USA). Cells were cultured at 37 °C with 5% CO2 in a constant temperature cell incubator, and moved into new media approximately every 3 days.
Cell transfection
Negative control siRNA (synthesised by the Guangzhou Ruibo Company) fluorescently labeled with cy3 was transfected into CAL-27 cells. The day before transfection, cells were seeded onto 24-well plates with a density of 1×105 per well in DMEM containing high glucose and no antibiotics to achieve normal growth. On the day of transfection, RNAiMAX liposome suspension (Invitrogen, USA) was used to dilute siRNA and mixed with the CAL-27 cells in OPTI-MEM I culture media without serum (Invitrogen, USA). Eight hours after transfection the medium was replaced. And 24 hours later fluorescence microscopy was used to monitor the transfection rate of tumor cells. Negative control siRNA and HIF-1α-specific-siRNA (Sense: 5'-CTGATGACCAGCAACTTGA-3') were transfected into CAL-27 cells as mentioned above.
Realtime-PCR
Negative control siRNA and HIF-1α-specific-siRNA, both synthesized by the Guangzhou Ruibo Company, were transfected into CAL-27 cells respectively. The cells were divided into three groups. The control group was transfected with negative control siRNA and its mRNA was extracted 48 h after transfection. The other two groups were transfected with HIF-1α-specific-siRNA, which have been verified against the HIF-1α gene (13). The total RNA was extracted 36 and 48 h after transfection. Reverse transcription reaction was carried out with M-MLV reverse transcriptase and dNTP which bought from PROMEGA. Real-time PCR was done using SYBR Master Mixture kit (TAKARA JPN) for the extracted cellular RNA (each group repeated three times and quantitative analysis for the mean).
The primer sequences were as follows:
hACTIN-F: CATGTACGTTGCTATCCAGGC;
hACTIN-R: CTCCTTAATGTCACGCACGAT;
HIF-1A-F: GCACAGGCCACATTCACGTATAT;
HIF-1A-R: GGTTCACAAATCAGCACCAAGC;
VEGF-F: GAGATGAGCTTCCTACAGCACAAC;
VEGF-R: GTACAAACAAATGCTTTCTCCGC.
Western blotting
Cells and tissue samples were harvested and lysed in RIPA buffer with protease inhibitors (Applygen Technologies, Inc., Beijing, China). Concentration was examined by the BCA Protein Assay. Proteins were separated on a sodium dodecyl sulfate polyacrylamide gel electrophoresis (SDS-PAGE) and transferred to a polyvinylidene difluoride membrane. The membranes were blocked in 5% non-fat dry milk for 1h and incubated in antibodies against HIF-1α, VEGF, and GAPDH (Dako, Carpinteria, California, USA) separately at 4 °C overnight. Following incubation with peroxidase-linked secondary antibodies, proteins were visualized by enhanced chemiluminescence reagent. Image J was used for quantification of Western blotting results.
Tumor xenografts
Ten female BALB/c-nu mice of 8 weeks old were prepared for tumor implantation. The BALB/c-nu mice were purchased from Shanghai Super-B&K laboratory animal Corp. Ltd. All animals were maintained in a sterile environment on a daily 12-h light/12-h dark cycle. CAL-27 cells (2×106/mouse) were injected subcutaneously into the flanks of the nude mice. When the tumor became palpable, the mice were divided into two groups randomly. The experimental group was intratumorally injected with 4 μg chemically synthesized siRNA of HIF-1α (synthesised by the Shanghai Jima Company) and RNAiMAX every day. The control group is treated with the same amount of negative control siRNA and RNAiMAX. Tumor volume was calculated every other day for 2 weeks according to this formula (14), TV (mm3) = length × width2 ×0.5. Tumor xenografts were harvested and weighted two weeks after the first siRNA injection. All the experiment protocol has been approved by the animal care and use committee in the Fifth Affiliated Hospital of Sun Yat-sen University.
Immunohistochemistry
Cryosections were cut at a thickness of 4 μm and stained with H&E. Immunohistochemical staining CD34 (Dako, Carpinteria, California, USA) was performed. Microvessel densities (MVD) determination was based on the criteria of the Weidner method: Under a low-power objective lens, the entire section was examined to search for regions of high capillary density, referred to as hot spots. A 200× objective lens was then used and the clusters of vascular endothelial cells with brown staining was counted. The final microvessel density was the average of three different microscopic fields (15).
Statistical analysis
All statistical analyses were performed by the SPSS WIN program package 13.0 (SPSS Inc., Chicago, IL, USA). We used the one-way anova test to analyze the microvessel densities, PCR and tumor xenografts results between experimental and control groups. Differences were considered statistically significant for a P value <0.05.
Results
High transfection efficiency of chemically synthesized siRNA by liposome
Chemically synthesized siRNA fluorescently labeled with cy3 was transmitted into the CAL-27 cells effectively by liposome. The cells with fluorescence were counted under a fluorescent inverted microscope. The transfection rate was analyzed by comparing the number of fluorescent cells with total cells. And we found that the transfection rate was over 90%.
HIF-1α siRNA significantly down-regulated the HIF-1α and VEGF expression in CAL-27 cells
PCR amplification curve was observed with fluorescence quantitative PCR. Our data showed that the HIF-1α and VEGF mRNA expression were significantly suppressed in the experimental groups 36 and 48 h after transfection (Figure 1A). Previous studies have showed that the same siRNA sequence effectively suppressed protein expression of HIF-1α in vivo or in vitro (16,17). Moreover, knocking down HIF-1α expression by siRNA induced apoptosis in tongue squamous cell carcinoma cells (18). Our data (Figure 1B) showed that HIF-1α siRNA significantly suppressed the protein expression of HIF-1α and VEGF in CAL-27 cells at 48 h comparing with control siRNA (P<0.05).
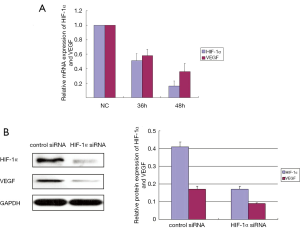
HIF-1α siRNA suppressed growth of TSCC xenografts
All the xenografted tumors were conformed as squamous cell carcinoma. Tremendously reduced tumor size and tumor weight were observed in mice treated with HIF-1α siRNA comparing with control siRNA (P<0.05) (Figure 2A-D). The tumor and surrounding tissue boundaries is clearer, and no obvious infiltration was found in the experimental group. However, the tumor in control group grew more rapidly and obviously infiltrated muscle. HIF-1α siRNA significantly suppressed the protein expression of HIF-1α and VEGF in xenografted tissue samples comparing with control siRNA (P<0.05) (Figure 2E). Immunochemical staining CD34 showed that the microvessel densities in tumor could be suppressed by target siRNA of HIF-1α (Table 1) (Figure 3).
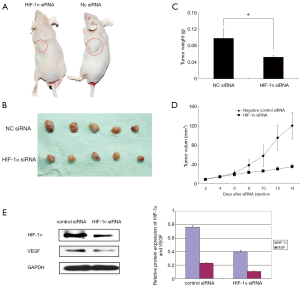
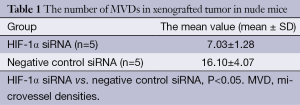
Full table
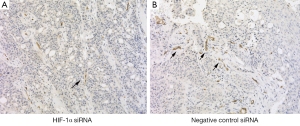
Discussion
Vascular networks are important for angiogenesis and it participates in the development, maintain of normal tissues and many pathological and physiological processes (19,20). Based upon the tumor angiogenesis theory suggested by Folkman (21), the development of solid tumors can be divided into two steps. The first is a pre-angiogenic phase in which the tumor grows slowly. But when blood vessels grow into tumors, it then enters the second step. At that time, the tumor grows rapidly and metastasis easily. Because the new blood vessels in the tumor provide adequate nutrients and remove much metabolic products, while also accelerating the formation of solid tumors (19,22). Weidner’s (23) study show that tumor MVD could serve as a reliable factor in patients with breast carcinoma for clinical outcome. MVD detection is usually used to determine angiogenesis. And it reflects the correlation of the angiogenesis and the invading behavior of tumours. So the prognostic value of MVD was soon verified in many different types of tumors, including oral tumors (11,24).
VEGF is one of participants in the complex angiogenic response, but it is an important activator of vascular endothelial cells. Moreover, VEGF and its receptors was therapeutic target for treatment of oral squamous cell carcinoma (25). It is reported that in some tumors VEGF and MVD is regulated by HIF-1α (26). In our study we transfected HIF-1α-specific-siRNA in TSCC cell lines in vitro and investigated the expression of HIF-1α and VEGF. We can found that when HIF-1α-specific-siRNA is transfected into CAL-27 cells, the mRNA expression of HIF-1α and VEGF decreased at 36 and 48 h (P<0.05). VEGF can be regulated by many genes (11), we believed that HIF-1α may be the major one in TSCC cells. In previous study, HIF-1α expression upregulated in injured gastric mucosal and esophageal ulcers, moreover the upregulation of HIF-1α correlated with VEGF gene activation and initiation of angiogenesis (27). And the reason for this is that when the tissue is injured it is under the hypoxic environment, HIF-1α accumulates in endothelial cells inducing VEGF expression. Once the tumor grows, the marginal tissue will necrotized, which makes a hypoxic conditions similar to the injury. Then it will promoted VEGF expression and angiogenesis. Because HIF-1α tries to inhibit apoptosis and cell death by inducing the expression of genes that induce mutations such as VEGF, at the same time, it will result in invasion and metastasis. All this chain of actions easily linked to the malignant alteration through the generation and procession of cancers (28). In order to further study the correlation between HIF-1α and VEGF, we suppressed HIF-1α expression by siRNA in TSCC cells, and found it downregulated VEGF expression. These results implied that HIF-1α may regulate VEGF expression, but the specific mechanism needs further study.
Finally, our results in TSCC xenografts experiment suggested that silencing HIF-1α inhibited the growth and angiogenesis of TSCC cells, which encouraged us to establish a siRNA-based therapy platform. Because the RNA interference (RNAi) technique is one of the most commonly used genetic treatment methods and it may be widely used in the future for targeting angiogenic factors. Therefore HIF-1α may serve as an effective indictor for patient’s survival and act as an important target for the tumor therapy.
Conclusions
HIF-1α regulated VEGF expression, and they play important roles in TSCC cell tumor growth. HIF-1α and VEGF may become new therapeutic targets for tongue squamous cell carcinoma.
Acknowledgements
This research has been approved by the appropriate animal care and use committee; the cell line was donated by Qin Xu of Shanghai Jiao Tong University. This work was supported by Medical Research Foundation of Guangdong province (Grant No. B2012146); Medical Technological Foundation of Guangzhou city (Grant No. 201102A213002); The Technological Foundation of Zhuhai city (Grant No. 2011B040102028); Science and Technology Project of Guangdong Province (No. 20120318015); The Youth physician Scientific Research Foundation of The Fifth Affiliated Hospital of Sun Yat-sen University (J Liang).
Disclosure: The authors declare no conflict of interest.
References
- Jemal A, Siegel R, Ward E, et al. Cancer statistics, 2007. CA Cancer J Clin 2007;57:43-66. [PubMed]
- Sessions DG, Spector GJ, Lenox J, et al. Analysis of treatment results for oral tongue cancer. Laryngoscope 2002;112:616-25. [PubMed]
- Semenza GL. Targeting HIF-1 for cancer therapy. Nat Rev Cancer 2003;3:721-32. [PubMed]
- López-Lázaro M. Hypoxia-inducible factor 1 as a possible target for cancer chemoprevention. Cancer Epidemiol Biomarkers Prev 2006;15:2332-5. [PubMed]
- Tanimoto K, Yoshiga K, Eguchi H, et al. Hypoxia-inducible factor-1alpha polymorphisms associated with enhanced transactivation capacity, implying clinical significance. Carcinogenesis 2003;24:1779-83. [PubMed]
- Lee JY, Choi JY, Lee KM, et al. Rare variant of hypoxia-inducible factor-1alpha (HIF-1A) and breast cancer risk in Korean women. Clin Chim Acta 2008;389:167-70. [PubMed]
- Alidoosti M, Ghaedi M, Soleimani A, et al. Study on the role of environmental parameters and HIF-1A gene polymorphism in coronary collateral formation among patients with ischemic heart disease. Clin Biochem 2011;44:1421-4. [PubMed]
- Shohet RV, Garcia JA. Keeping the engine primed: HIF factors as key regulators of cardiac metabolism and angiogenesis during ischemia. J Mol Med (Berl) 2007;85:1309-15. [PubMed]
- Rey S, Semenza GL. Hypoxia-inducible factor-1-dependent mechanisms of vascularization and vascular remodelling. Cardiovasc Res 2010;86:236-42. [PubMed]
- Yan L, Chen WL, Zeng SG, et al. Inhibition of VEGF expression in tongue squamous cancer cells via RNA interference silencing of iNOS gene. Int J Oral Maxillofac Surg 2009;38:369-73. [PubMed]
- Ou Yang KX, Liang J, Huang ZQ. Association of clinicopathologic parameters with the expression of inducible nitric oxide synthase and vascular endothelial growth factor in mucoepidermoid carcinoma. Oral Dis 2011;17:590-6. [PubMed]
- Semenza G. Signal transduction to hypoxia-inducible factor 1. Biochem Pharmacol 2002;64:993-8. [PubMed]
- Wei L, Lu J, Feng L, et al. HIF-1alpha accumulation upregulates MICA and MICB expression on human cardiomyocytes and enhances NK cell cytotoxicity during hypoxia-reoxygenation. Life Sci 2010;87:111-9. [PubMed]
- Li J, Huang H, Sun L, et al. MiR-21 indicates poor prognosis in tongue squamous cell carcinomas as an apoptosis inhibitor. Clin Cancer Res 2009;15:3998-4008. [PubMed]
- Weidner N. Intratumor microvessel density as a prognostic factor in cancer. Am J Pathol 1995;147:9-19. [PubMed]
- Chiche J, Ilc K, Laferrière J, et al. Hypoxia-inducible carbonic anhydrase IX and XII promote tumor cell growth by counteracting acidosis through the regulation of the intracellular pH. Cancer Res 2009;69:358-68. [PubMed]
- Jeong JK, Seo JS, Moon MH, et al. Hypoxia-inducible factor-1 α regulates prion protein expression to protect against neuron cell damage. Neurobiol Aging 2012;33:1006.e1-10.
- Zhang Q, Zhang ZF, Rao JY, et al. Treatment with siRNA and antisense oligonucleotides targeted to HIF-1alpha induced apoptosis in human tongue squamous cell carcinomas. Int J Cancer 2004;111:849-57. [PubMed]
- Shibuya M. Role of VEGF-flt receptor system in normal and tumor angiogenesis. Adv Cancer Res 1995;67:281-316. [PubMed]
- Folkman J, Shing Y. Angiogenesis. J Biol Chem 1992;267:10931-4. [PubMed]
- Folkman J. Tumor angiogenesis: therapeutic implications. N Engl J Med 1971;285:1182-6. [PubMed]
- Hanahan D, Folkman J. Patterns and emerging mechanisms of the angiogenic switch during tumorigenesis. Cell 1996;86:353-64. [PubMed]
- Weidner N, Folkman J, Pozza F, et al. Tumor angiogenesis: a new significant and independent prognostic indicator in early-stage breast carcinoma. J Natl Cancer Inst 1992;84:1875-87. [PubMed]
- Kumamoto H, Ohki K, Ooya K. Association between vascular endothelial growth factor (VEGF) expression and tumor angiogenesis in ameloblastomas. J Oral Pathol Med 2002;31:28-34. [PubMed]
- Yazici YD, Kim S, Jasser SA, et al. Antivascular therapy of oral tongue squamous cell carcinoma with PTK787. Laryngoscope 2005;115:2249-55. [PubMed]
- Fong GH. Mechanisms of adaptive angiogenesis to tissue hypoxia. Angiogenesis 2008;11:121-40. [PubMed]
- Ahluwalia A, Tarnawski AS. Critical role of hypoxia sensor--HIF-1α in VEGF gene activation. Implications for angiogenesis and tissue injury healing. Curr Med Chem 2012;19:90-7. [PubMed]
- Kizaka-Kondoh S, Tanaka S, Hanada H, et al. The HIF-1-active microenvironment: an environmental target for cancer therapy. Adv Drug Deliv Rev 2009;61:623-32. [PubMed]