Past, current and future management of secondary mitral valve disease: the importance of anatomic staging
Introduction
Mitral valve (MV) repair is superior to replacement for treating primary mitral disease (1-3). In appropriately selected patients, repair is also optimal for secondary disease (4). Mitral valve repair is potentially curative and is associated with long-term survival similar to that seen in patients without heart disease (5,6). When compared to repair, MV replacement is associated with increased operative mortality, stroke and bleeding complications, prosthetic device failure, and decreased long-term survival (6,7). However, the rate of mitral repair is decreasing (6,8). This trend may be explained by the results of Cardiothoracic Surgical Network (CTSNet) trial for treating severe mitral regurgitation (MR) (9). The CTSNet trial demonstrated a 33% rate of recurrent MR 12-months following simple reductive ring annuloplasty. The authors concluded that valve replacement achieved greater freedom from recurrent MR. However, valve distortion from secondary disease was not quantified prior to enrollment in the study and repair was not image-guided. The authors subsequently reported that left ventricular (LV) remodeling following durable repair was superior to valve replacement (10). Like CTSNet, the Percutaneous Repair with the MitraClip Device for Severe Functional/Secondary Mitral Regurgitation (Mitra-FR) trial did not quantify tethering prior to enrollment (11). Percutaneous edge-to-edge repair was used to treat the full spectrum of secondary disease. Neither trial favored MV repair.
Most recently, the Cardiovascular Assessment of MitraClip Percutaneous Therapy for Heart Failure Patients with Functional Mitral Regurgitation (COAPT) trial used transesophageal echocardiography (TEE) prior to enrollment to select patients with valve anatomy that was suitable for percutaneous edge-to-edge repair (12). Using image guidance, the COAPT investigators achieved a 95% freedom from recurrent MR at 12 months and demonstrated improved survival at 24 months. The CTSNet and COAPT trials demonstrate that durable repair for severe secondary mitral regurgitation improves LV remodeling and prolongs survival. These trials also demonstrate that image guidance is mandatory for optimal results. The role and application of image guidance have not been defined. Evidence-based, anatomic staging of secondary mitral disease is urgently needed (13).
Repair of secondary mitral valve disease
Surgical repair of secondary mitral disease is evolving. The historical approach of simple reductive ring annuloplasty for the full spectrum of secondary disease has largely been abandoned due to recurrent MR (2). Recurrent MR is common following ring annuloplasty if significant tethering is present or remodeling continues (14,15). Consequently, the indications for simple ring annuloplasty repair are specific and relatively infrequent (14,16). Valve replacement increases perioperative mortality, increases morbidity and decreases long-term survival (6,7). Therefore, for most patients with secondary MR, the binary approach (simple ring annuloplasty versus valve replacement) is not ideal (2). Several more complex techniques for repair of advanced secondary disease have been validated (4,14,17). These procedures use image guidance and complex reconstructive techniques to repair of the full spectrum of secondary disease.
Contemporary percutaneous MV repair is based on the surgical edge-to-edge repair (18,19). Mitral competence is restored by securing the free edges of the anterior and posterior leaflets together to create a double orifice valve. The early surgical results for treating secondary MR showed 95% freedom from recurrent MR at 18 months (20). Ring annuloplasty and reverse remodeling were both independently associated with durable surgical repair. However, concerns over high gradients and limited durability prevented widespread adoption of the edge-to-edge repair in the surgical community (21). The edge-to-edge technique reduces maximum flow rate across the valve by up to 50% and doubles stress on the leaflet free margin (22). Mitral valve gradients >5 mmHg following percutaneous edge-to-edge repair are associated with increased long-term mortality (23). When compared to percutaneous edge-to-edge repair, surgical repair results in greater freedom from recurrent MR (24). Nonetheless, the percutaneous edge-to-edge technique is effective when imaging is used to carefully select patients with no more than moderate tethering (12).
Recently, newer surgical techniques have been validated for repair of advanced tethering. Image-guided subvalvular procedures—including chordal cutting, papillary approximation/alignment and ventriculoplasty—have been effective for severe tethering (4,14,25). The future of valve repair will likely center on leaflet augmentation because it is the logical extension of naturally occurring adaptive growth and, unlike papillary approximation, does not exacerbate diastolic dysfunction (4,26-28).
Imaging secondary mitral valve disease
Secondary mitral disease can be subdivided into pathoanatomy and pathophysiology. Pathoanatomy is summarized in Carpentier’s classic pathologic triad: disease causes lesions that result in leaflet dysfunction (29). For example, cardiomyopathies cause papillary displacement that results in leaflet motion restricted in systole (Carpentier Type IIIb). Pathoanatomy results in pathophysiology. The pathophysiology of secondary mitral disease is valve regurgitation. The severity of MR can be stratified to identify the need for intervention (30). For example, severe MR is an indication for intervention. However, the pathophysiologic severity does not identify the appropriate intervention. Surgical or percutaneous intervention for secondary mitral disease must be guided by pathoanatomy (4,12,31). This is no different than cancer. For instance, the pathophysiology of bronchogenic carcinoma indicates the need to intervene. But it is the anatomic stage of the disease that determines the most appropriate intervention. Lobectomy is indicated for anatomic stage I neoplastic disease. Similarly, simple reductive ring annuloplasty is indicated for annular dilation with minimal tethering. Like cancer, secondary mitral disease must be anatomically staged and used to guide intervention (13,31). Again, like cancer, separate and unique imaging techniques are required to stratify the pathoanatomy and the pathophysiology of MV disease.
Standardized imaging
Anatomic stage-based intervention requires careful standardization of imaging. The concept of axial imaging for surgical mitral intervention was introduced in 2014 (Figure 1) (4,31). Currently, axial imaging is used almost exclusively for guiding percutaneous intervention (12).
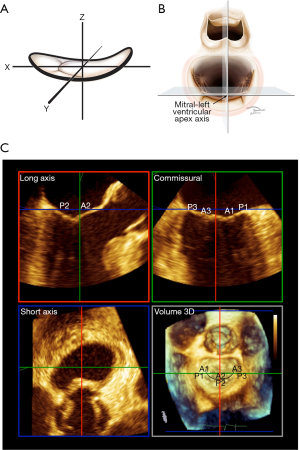
Axial imaging is based on the mathematical description of the MV. During systole, the atrial surface of the valve resembles a riding saddle, which can be modelled as a hyperbolic paraboloid with three cartesian axes, x, y and z. The z-axis extends in an apical-basal basal orientation, from the LV apex to center of the mitral valve (Figure 1). The z-axis is referred to as the mitral-left ventricular apex axis. The x-axis extends from the anterior horn to the posterior mitral annulus and the y-axis extends between the two commissures of the valve. With TEE, the in mid-esophageal long axis and commissural views image the MV along the x- and y-axes respectively, intersecting at the center of the valve and running parallel to the mitral LV apex axis. The basal short axis view runs perpendicular to all three axes at the level of the mitral annulus. The left atrial “surgeon’s” view ensures that the long-axis and commissural views are traversing the center of the valve. Landmark recognition and the elimination of parallax are mandatory. Axial imaging yields highly reproducible results. Leaflet tethering is quantified in axial long axis. The imaging techniques have been described in detail (31). The mid-esophageal four- and two-chamber views have long been known to underestimate tethering and overestimate prolapse (32,33). Consequently, there is little if any role for the four- and two-chamber views in guiding mitral intervention (31). Automated algorithms and artificial intelligence may improve reproducibility and facilitate rapid acquisition of indices in the future (34,35).
When compared to TEE, transthoracic echocardiography underestimates tenting height and overestimates tenting area (36). Current guidelines provide a class I indication for TEE to determine the mechanism of MR and status of the LV (1). Given the minimal risk of TEE examination and substantial mortality of recurrent MR, TEE should be performed well in advance of intervention and used for identifying the best options for management.
Secondary mitral regurgitation is bi-phasic with peak regurgitant flows in early and late systole (37,38). Identification of mid-systole is burdensome and intrinsically ambiguous. Arrhythmias including atrial fibrillation further complicate the consistent identification of mid-systole. For these reasons, late systole should be used for analysis.
The mitral annulus is a fibrous ring that surrounds the leaflets and is an important anatomic feature for both surgical and percutaneous interventions. Imaging for mitral intervention requires proper identification of the anatomic mitral analysis. The atrial surface of the valve identifies the plane of the annulus. The mitral annulus is 1–2 mm peripheral to the leaflet hinge point. Tenting heights, tenting areas, and leaflet closing angles should all be based on the anatomic annulus in long-axis.
Currently the literature appears to favor the end-systolic long-axis view using transesophageal echocardiography in the lightly sedated patient. Three-dimensional post-acquisition analysis greatly facilitates accurate stratification of disease (4).
Indices of secondary disease
The anatomic indices of secondary disease include direct and indirect measurements. Direct indices quantify leaflet dysfunction. They are anatomic measurements taken directly from the valve and are the most important parameters for guiding surgical and percutaneous repair (4,12,14). These indices include tenting height, tenting area, anterior leaflet closing angle, posterior leaflet closing angle, and anterior leaflet inversion angle (Figure 2A). Coaptive length, coaptive gap and interpapillary distances may also be useful. Indirect indices are less precise and include ventricular dimensions, contractility, scar burden, and restrictive diastology. Indirect indices are important for selecting palliative options such as surgical or percutaneous valve replacement, transplantation, ventricular assist devices, or medical therapy alone (39,40).
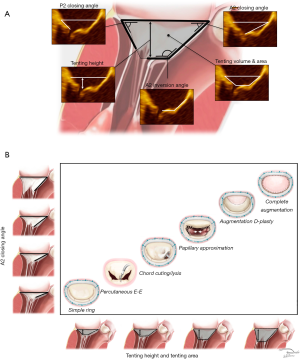
Staging
Stage I identifies patients with minimal tethering. Tenting height is less than a centimeter below the plane of the annulus and the tenting area is less than one centimeter squared. Stage I is relatively infrequent and the dominant lesion appears to be annular dilation from a basil myopathy secondary to atrial fibrillation (atrial functional MR) (4,14,16,35,41). Patients with Stage I disease and stable myopathies can be expected to achieve long-term freedom from recurrent MR using either simple reductive ring annuloplasty or percutaneous edge-to-edge repair. Surgeons who choose a binary approach consisting of simple reductive ring annuloplasty repair or valve replacement, should limit repair to Stage I disease. The combined lesions of a global myopathy from chronic MR superimposed on annular dilation and bileaflet prolapse from Barlow disease (Carpentier type II + IIIb = I) can also present as Stage I (31,42). These combined lesions must be recognized prior to intervention because of the increased risk of systolic anterior motion following simple reductive ring annuloplasty.
Stage II identifies moderate leaflet tethering. Tenting height is still less than a centimeter but tenting area exceeds a centimeter squared. There is no significant leaflet angular distortion although anterior leaflet inversion from tethering of the secondary chordae tendonae may be present. Mitral repair can be accomplished with simple subvalvular techniques such as anterior secondary chord lysis. These procedures are always combined with reductive ring annuloplasty (4,17,43-45). The percutaneous edge-to-edge repair is also effective for Stage II disease.
Stage III identifies advanced tethering. The tenting height exceeds one and the tenting area is typically greater than two centimeters squared. All leaflet angles demonstrate severe distortion. Advanced tethering is associated with nearly uniform failure of both simple reductive ring annuloplasty and percutaneous edge-to-edge repair. This is reflected in the 2016 European Guidelines and the 2017 American College of Cardiology (ACC) Expert Consensus. Complex surgical procedures are required for MV reconstruction (2,46). The surgical edge-to-edge repair combined with ring annuloplasty has demonstrated excellent freedom from recurrent MR but the results from percutaneous edge-to-edge repair are poor (24).
Stage IV identifies patients with severe tethering and severe ventricular remodeling. These patients are likely to have recurrent MR following all repairs and may derive no benefit from mitral intervention of any type. Indirect indices are more important for identifying Stage IV disease. A basilar aneurysm, restrictive diastology or proportionate MR may also be present (47). The 2016 American Association for Thoracic Surgery and 2017 American Heart Association/ACC Guidelines indicate that MV replacement is reasonable if significant preoperative tethering is present (2,48). In patients with true end-stage heart failure, MR may no longer be of prognostic significance (49).
As demonstrated in Figure 2B, axial imaging can be used to anatomically stage MV disease. The direct indices provide the basis for stage-based intervention across the full spectrum of secondary disease.
Conclusions
Severe regurgitation from secondary mitral disease increases mortality (30,50). Durable repair improves LV remodeling and survival (10,12). Conversely, valve replacement is associated with increased operative mortality, bleeding and embolic complications, prosthetic device failure, and decreased long-term survival (6,7).
There is evidence to support a simple anatomic staging system for secondary mitral disease. Standardized imaging is required for staging. The first three stages use direct indices to quantify minimal, moderate and severe tethering. Each stage is associated with increasing procedural complexity for interventional success. The fourth stage is associated with failure of all repair techniques.
The historical approach of simple reductive ring annuloplasty for the full spectrum of secondary disease has been abandoned and the binary approach is far from ideal (2,9). Using image guidance, several surgical techniques for have been validated for repairing advanced disease (4,14,17). They allow for comprehensive reconstruction of the full spectrum of secondary MV disease.
Acknowledgments
Funding: None.
Footnote
Conflicts of Interest: All authors have completed the ICMJE uniform disclosure form (available at http://dx.doi.org/10.21037/atm.2020.03.82). The series “Structural Heart Disease: The Revolution” was commissioned by the editorial office without any funding or sponsorship. The authors have no other conflicts of interest to declare.
Ethical Statement: The authors are accountable for all aspects of the work in ensuring that questions related to the accuracy or integrity of any part of the work are appropriately investigated and resolved.
Open Access Statement: This is an Open Access article distributed in accordance with the Creative Commons Attribution-NonCommercial-NoDerivs 4.0 International License (CC BY-NC-ND 4.0), which permits the non-commercial replication and distribution of the article with the strict proviso that no changes or edits are made and the original work is properly cited (including links to both the formal publication through the relevant DOI and the license). See: https://creativecommons.org/licenses/by-nc-nd/4.0/.
References
- Nishimura RA, Otto CM, Bonow RO, et al. 2014 AHA/ACC guideline for the management of patients with valvular heart disease: a report of the American College of Cardiology/American Heart Association Task Force on Practice Guidelines. J Am Coll Cardiol 2014;63:e57-185. [Crossref] [PubMed]
- Nishimura RA, Otto CM, Bonow RO, et al. 2017 AHA/ACC Focused Update of the 2014 AHA/ACC Guideline for the Management of Patients With Valvular Heart Disease: A Report of the American College of Cardiology/American Heart Association Task Force on Clinical Practice Guidelines. J Am Coll Cardiol 2017;70:252-89. [Crossref] [PubMed]
- Shuhaiber J, Anderson RJ. Meta-analysis of clinical outcomes following surgical mitral valve repair or replacement. Eur J Cardiothorac Surg 2007;31:267-75. [Crossref] [PubMed]
- Drake DH, Zimmerman KG, Hepner AM, et al. Echo-guided mitral repair. Circ Cardiovasc Imaging 2014;7:132-41. [Crossref] [PubMed]
- Badhwar V, Peterson ED, Jacobs JP, et al. Longitudinal outcome of isolated mitral repair in older patients: results from 14,604 procedures performed from 1991 to 2007. Ann Thorac Surg 2012;94:1870-7; discussion 1877-9.
- Vassileva CM, Boley T, Markwell S, et al. Meta-analysis of short-term and long-term survival following repair versus replacement for ischemic mitral regurgitation. Eur J Cardiothorac Surg 2011;39:295-303. [Crossref] [PubMed]
- Dangas GD, Weitz JI, Giustino G, et al. Prosthetic Heart Valve Thrombosis. J Am Coll Cardiol 2016;68:2670-89. [Crossref] [PubMed]
- Gammie JS, Sheng S, Griffith BP, et al. Trends in mitral valve surgery in the United States: results from the Society of Thoracic Surgeons Adult Cardiac Surgery Database. Ann Thorac Surg 2009;87:1431-7; discussion 1437-9. [Crossref] [PubMed]
- Acker MA, Parides MK, Perrault LP, et al. Mitral-valve repair versus replacement for severe ischemic mitral regurgitation. N Engl J Med 2014;370:23-32. [Crossref] [PubMed]
- Acker MA, Dagenais F, Goldstein D, et al. Severe ischemic mitral regurgitation: Repair or replace? J Thorac Cardiovasc Surg 2015;150:1425-7. [Crossref] [PubMed]
- Obadia JF, Messika-Zeitoun D, Leurent G, et al. Percutaneous Repair or Medical Treatment for Secondary Mitral Regurgitation. N Engl J Med 2018;379:2297-306. [Crossref] [PubMed]
- Stone GW, Lindenfeld J, Abraham WT, et al. Transcatheter Mitral-Valve Repair in Patients with Heart Failure. N Engl J Med 2018;379:2307-18. [Crossref] [PubMed]
- Drake DH, Zimmerman KG, Sidebotham DA. Transcatheter Mitral-Valve Repair in Patients with Heart Failure. N Engl J Med 2019;380:1978-9. [Crossref] [PubMed]
- Harmel EK, Reichenspurner H, Girdauskas E. Subannular reconstruction in secondary mitral regurgitation: a meta-analysis. Heart 2018;104:1783-90. [Crossref] [PubMed]
- De Bonis M, Lapenna E, Verzini A, et al. Recurrence of mitral regurgitation parallels the absence of left ventricular reverse remodeling after mitral repair in advanced dilated cardiomyopathy. Ann Thorac Surg 2008;85:932-9. [Crossref] [PubMed]
- Kihara T, Gillinov AM, Takasaki K, et al. Mitral regurgitation associated with mitral annular dilation in patients with lone atrial fibrillation: an echocardiographic study. Echocardiography 2009;26:885-9. [Crossref] [PubMed]
- Calafiore AM, Refaie R, Iaco AL, et al. Chordal cutting in ischemic mitral regurgitation: a propensity-matched study. J Thorac Cardiovasc Surg 2014;148:41-6. [Crossref] [PubMed]
- Alfieri O, Maisano F, De Bonis M, et al. The double-orifice technique in mitral valve repair: a simple solution for complex problems. J Thorac Cardiovasc Surg 2001;122:674-81. [Crossref] [PubMed]
- Fucci C, Sandrelli L, Pardini A, et al. Improved results with mitral valve repair using new surgical techniques. Eur J Cardiothorac Surg 1995;9:621-6; discuss 626-7.
- De Bonis M, Lapenna E, La Canna G, et al. Mitral valve repair for functional mitral regurgitation in end-stage dilated cardiomyopathy: role of the "edge-to-edge" technique. Circulation 2005;112:I402-8. [PubMed]
- Bhudia SK, McCarthy PM, Smedira NG, et al. Edge-to-edge (Alfieri) mitral repair: results in diverse clinical settings. Ann Thorac Surg 2004;77:1598-606. [Crossref] [PubMed]
- Lau KD, Diaz-Zuccarini V, Scambler P, et al. Fluid-structure interaction study of the edge-to-edge repair technique on the mitral valve. J Biomech 2011;44:2409-17. [Crossref] [PubMed]
- Neuss M, Schau T, Isotani A, et al. Elevated Mitral Valve Pressure Gradient After MitraClip Implantation Deteriorates Long-Term Outcome in Patients With Severe Mitral Regurgitation and Severe Heart Failure. JACC Cardiovasc Interv 2017;10:931-9. [Crossref] [PubMed]
- De Bonis M, Taramasso M, Lapenna E, et al. MitraClip therapy and surgical edge-to-edge repair in patients with severe left ventricular dysfunction and secondary mitral regurgitation: mid-term results of a single-centre experience. Eur J Cardiothorac Surg 2016;49:255-62. [Crossref] [PubMed]
- Pausch J, Harmel E, Sinning C, et al. Standardized subannular repair for type IIIb functional mitral regurgitation in a minimally invasive mitral valve surgery setting. Eur J Cardiothorac Surg 2019;56:968-75. [Crossref] [PubMed]
- Obase K, Matsumaru I, Miura T, et al. Echocardiographic visualization and quantification of mitral complex during mitral repair for severe functional mitral regurgitation. J Thorac Cardiovasc Surg 2017;154:1252-5. [Crossref] [PubMed]
- Obase K, Weinert L, Hollatz A, et al. Elongation of chordae tendineae as an adaptive process to reduce mitral regurgitation in functional mitral regurgitation. Eur Heart J Cardiovasc Imaging 2016;17:500-9. [Crossref] [PubMed]
- Debonnaire P, Al Amri I, Leong DP, et al. Leaflet remodelling in functional mitral valve regurgitation: characteristics, determinants, and relation to regurgitation severity. Eur Heart J Cardiovasc Imaging 2015;16:290-9. [Crossref] [PubMed]
- Carpentier A, Adams DH, Filsoufi F. Carpentier’s Reconstructive Valve Surgery. 1st ed. Philadelphia, PA: Elsevier, 2010.
- Bartko PE, Arfsten H, Heitzinger G, et al. A Unifying Concept for the Quantitative Assessment of Secondary Mitral Regurgitation. J Am Coll Cardiol 2019;73:2506-17. [Crossref] [PubMed]
- Drake DH, Zimmerman KG, Sidebotham DA. Transesophageal Echocardiography for Surgical Repair of Mitral Regurgitation. In: Otto CM. editor. Practice of Clinical Echocardiography. 5th ed. Philadelphia, PA: Elsevier, 2016:343-73.
- Levine RA, Triulzi MO, Harrigan P, et al. The relationship of mitral annular shape to the diagnosis of mitral valve prolapse. Circulation 1987;75:756-67. [Crossref] [PubMed]
- Magne J, Pibarot P, Dagenais F, et al. Preoperative posterior leaflet angle accurately predicts outcome after restrictive mitral valve annuloplasty for ischemic mitral regurgitation. Circulation 2007;115:782-91. [Crossref] [PubMed]
- Wijdh-den Hamer IJ, Bouma W, Lai EK, et al. The value of preoperative 3-dimensional over 2-dimensional valve analysis in predicting recurrent ischemic mitral regurgitation after mitral annuloplasty. J Thorac Cardiovasc Surg 2016;152:847-59. [Crossref] [PubMed]
- Kim DH, Heo R, Handschumacher MD, et al. Mitral Valve Adaptation to Isolated Annular Dilation: Insights Into the Mechanism of Atrial Functional Mitral Regurgitation. JACC Cardiovasc Imaging 2019;12:665-77. [Crossref] [PubMed]
- Grayburn PA, She L, Roberts BJ, et al. Comparison of Transesophageal and Transthoracic Echocardiographic Measurements of Mechanism and Severity of Mitral Regurgitation in Ischemic Cardiomyopathy (from the Surgical Treatment of Ischemic Heart Failure Trial). Am J Cardiol 2015;116:913-8. [Crossref] [PubMed]
- Schwammenthal E, Chen C, Benning F, et al. Dynamics of mitral regurgitant flow and orifice area. Physiologic application of the proximal flow convergence method: clinical data and experimental testing. Circulation 1994;90:307-22. [Crossref] [PubMed]
- Topilsky Y, Vaturi O, Watanabe N, et al. Real-time 3-dimensional dynamics of functional mitral regurgitation: a prospective quantitative and mechanistic study. J Am Heart Assoc 2013;2:e000039. [Crossref] [PubMed]
- Ereminiene E, Vaskelyte J, Benetis R, et al. Ischemic mitral valve repair: predictive significance of restrictive left ventricular diastolic filling. Echocardiography 2005;22:217-24. [Crossref] [PubMed]
- Kusunose K, Obuchowski NA, Gillinov M, et al. Predictors of Mortality in Patients With Severe Ischemic Cardiomyopathy Undergoing Surgical Mitral Valve Intervention. J Am Heart Assoc 2017. [Crossref] [PubMed]
- Tang Z, Fan YT, Wang Y, et al. Mitral Annular and Left Ventricular Dynamics in Atrial Functional Mitral Regurgitation: A Three-Dimensional and Speckle-Tracking Echocardiographic Study. J Am Soc Echocardiogr 2019;32:503-13. [Crossref] [PubMed]
- Otani K, Takeuchi M, Kaku K, et al. Evidence of a vicious cycle in mitral regurgitation with prolapse: secondary tethering attributed to primary prolapse demonstrated by three-dimensional echocardiography exacerbates regurgitation. Circulation 2012;126:S214-21. [Crossref] [PubMed]
- Messas E, Pouzet B, Touchot B, et al. Efficacy of chordal cutting to relieve chronic persistent ischemic mitral regurgitation. Circulation 2003;108 Suppl 1:II111-5. [Crossref] [PubMed]
- Borger MA, Murphy PM, Alam A, et al. Initial results of the chordal-cutting operation for ischemic mitral regurgitation. J Thorac Cardiovasc Surg 2007;133:1483-92. [Crossref] [PubMed]
- Szymanski C, Bel A, Cohen I, et al. Comprehensive annular and subvalvular repair of chronic ischemic mitral regurgitation improves long-term results with the least ventricular remodeling. Circulation 2012;126:2720-7. [Crossref] [PubMed]
- De Bonis M, Al-Attar N, Antunes M, et al. Surgical and interventional management of mitral valve regurgitation: a position statement from the European Society of Cardiology Working Groups on Cardiovascular Surgery and Valvular Heart Disease. Eur Heart J 2016;37:133-9. [Crossref] [PubMed]
- Grayburn PA, Sannino A, Packer M. Proportionate and Disproportionate Functional Mitral Regurgitation: A New Conceptual Framework That Reconciles the Results of the MITRA-FR and COAPT Trials. JACC Cardiovasc Imaging 2019;12:353-62. [Crossref] [PubMed]
- Kron IL, LaPar DJ, Acker MA, et al. 2016 update to The American Association for Thoracic Surgery (AATS) consensus guidelines: Ischemic mitral valve regurgitation. J Thorac Cardiovasc Surg 2017;153:e97-114. [Crossref] [PubMed]
- Goliasch G, Bartko PE, Pavo N, et al. Refining the prognostic impact of functional mitral regurgitation in chronic heart failure. Eur Heart J 2018;39:39-46. [Crossref] [PubMed]
- Grigioni F, Enriquez-Sarano M, Zehr KJ, et al. Ischemic mitral regurgitation: long-term outcome and prognostic implications with quantitative Doppler assessment. Circulation 2001;103:1759-64. [Crossref] [PubMed]