Trehalose enhances bone fracture healing in a rat sleep deprivation model
Introduction
Sleep deprivation (SD) is a common problem. An increase number of adults experience SD in modern society (1). It is reported that approximately one-third of adults get less than 6.5 h of sleep per night (2). While, the American National Sleep Foundation suggests that the healthy grown-ups should sleep for 7–9 h/day. Similarly, many adolescents suffer from sleep problem because of stress or early school start time (3). SD may contribute to various kinds of disorders, such as diabetes (4), cardiovascular disease (5), circadian rhythm problems (6), and metabolic syndrome (7). Several studies have showed that SD may lead to decreased bone mineral density (BMD) (8-11). It has been reported that the individuals who sleep for 6 h or less have significantly lower BMD compared to the ones who sleep for 8 h (8). Similarly, other clinical surveys showed that patients with SD have lower BMD (9-11). An animal study showed that SD affects bone health by decreasing BMD and 25(OH)D (12). The study also demonstrated that SD affected BMD by decreasing osteogenesis and impairing mineralization of the newly formed bones.
A high number of people in the world are involved in fractures, which are the most frequently injuries of the musculoskeletal system (13). Up still now, a large proportion of all fractures still display delayed healing or nonunion (14). Delayed fracture healing is attributed to several risk factors, such as type of injury, fracture treatment, age, gender, comorbidities, alcohol consumption, smoking, and medications (15).
The switch from the initially pro-inflammatory M1 macrophage to the anti-inflammatory M2 phenotype is essential for bone fracture healing (16). We hypothesized that SD, a systemic inflammatory factor, may also affect bone fracture healing, and the medications that could enhance M2 polarization may help accelerate fracture healing.
Trehalose is a natural disaccharide with chemical chaperone activity. As it has the ability to maintain the stability of nucleic acid and protein, trehalose has been widely used as a stabilizer in drugs, foods, and transplantation surgery (17,18). Additionally, trehalose was reported to be bioactive in various kinds of disease (i.e., Alzheimer, osteoarthritis, and osteoporosis) (19-21). Recently, it has been suggested that trehalose has an anti-inflammatory effect and may be a new therapeutic approach for treatment of inflammation (22). However, whether trehalose could enhance the healing of pathological delayed bone fracture union and direct macrophage polarization requires further investigation.
In the present study, an experimental SD with femoral fracture model was used. The effect of SD and trehalose on healing quality of bone fracture, and anti-inflammatory activity of trehalose were evaluated.
Methods
Laboratory animals
All animal care and surgical procedures were carried out in accordance with the guidelines and approved by the Ethics Committee of Drum Tower Hospital, Medical School of Nanjing University, Nanjing, China. Eighteen 300–350 g female Sprague-Dawley rats obtained from the Laboratory Animal Center of Drum Tower Hospital were randomly divided into fracture group (group 1, n=6), fracture + SD group (group 2, n=6), and fracture + SD + Tre group (group 3, n=6). Before the experiments, all animals were housed under the same conditions (humidity, 45%±5%; temperature, 25±2 °C; 12 h/12 h light/dark cycle) for a week. The rats were fed a regular diet twice a day and allowed free access to water during the whole study.
Bone fracture
A mid-femoral transverse osteotomy was created in each animal, as described previously (23,24). Briefly, the surgical procedure was performed under general anesthesia and sterile conditions. A lateral incision was made on the right thigh of the rats to expose the femur. A thin oscillating blade was used to create a mid-femoral transverse osteotomy. The periosteum of the fracture site was removed to avoid fast healing. Then, the articular surface of the distal femur was exposed by a middle incision on the right knee. A 1.1 mm Kirschner wire was inserted in the medullary canal to fix the fracture in a retrograde fashion. The wire was cut to the level of the articular surface of the knee. The incision was closed with absorbable suture.
Experimental SD and trehalose administrated
A modified multiple platform method that was illustrated previously was selected to make the SD model in the study (25,26). Six narrow (6.5 cm in diameter) platforms were placed in the water tanks (60×40×30 cm3) that were made of organic glass. The rats were housed in the platforms. In the SD making group (groups 2 and 3), the water tank was filled with water to a level of 1 cm below the upper surface of the platforms. When the rats reached the paradoxical phase of sleep, which is characterized by muscle atonia, their face would touch the water, and they would wake up. The housing conditions in group 1 were similar, but there was no water in the water tank, and the rats could move freely and fall into asleep. Seven days after bone fracture procedure, the rats were started to get sleep-deprived using the platform for 18 h (starting at 16:00 h) per day for 3 weeks. The rats were allowed to sleep in their normal cage for the remaining time (6 h, 10:00–16:00 h). All rats have free access to water and food. Other conditions were kept constant (humidity, 45%±5%; temperature, 25±2 °C; 12 h/12 h light/dark cycle). The rats in group 3 were injected with trehalose intraperitoneally at 1 g/kg/d since SD procedure began.
Radiological analysis
The rats were sacrificed 4 weeks after surgery, and the lower limbs were harvested. After removing the soft tissue, the specimens were X-rayed (MX-20; Faxitron Biooptics, Tucson, USA) to evaluate bone union. The limbs were laterally placed and exposed to a voltage of 45 kV for 5 s. The callus area was measured by two independent observers.
The fracture callus were scanned with a micro CT system (uCT-80, Scanco Medical, Bassersdorf, Switzerland). The micro-CT scanner was set at a voltage of 70 kVp and a current of 114 µA to collect images at a resolution of 15.6 um per pixel. Serial cross-sectional images of the callus areas were collected to perform three-dimensional histomorphometric analysis. Several architectural and densitometry parameters were determined: BMD, mineralized volume fraction (BV/TV), trabecular thickness (Tb.Th), trabecular number (Tb.N), and trabecular separation (Tb.Sp) (27).
Histological analysis
After X-ray and micro CT scan, the specimens were fixed in 4% paraformaldehyde and then decalcified in a 5% EDTA solution. Afterward, the specimens were dehydrated with a series of ethanol rinses and embedded in paraffin. Then, longitudinal sections (5 µm thickness) were prepared using a microtome (Leica RM2165, Leica, Germany). Representative sections were stained with haematoxylin eosin (H&E) and Masson staining. The imagines were scanned using case viewer software 3.3 (JAVS, Inc., Louisville, USA).
Enzyme linked immunosorbent assay (ELISA)
Blood was collected from the rat hearts after anesthesia, and a total of 0.5–1.5 mL of blood was collected. Serum samples were obtained by centrifugation of blood at 3,000 rpm for 10 minutes and stored at −80 °C before analysis. The plasma concentrations of the biomarkers serum interleukin-1 beta (IL-1β) and tumor necrosis factor-alpha (TNF-α) were measured using an assay (Proteintech, Chicago, USA) according to the manufacturer's instructions.
Cell culture
RAW264.7 murine macrophage cells (Institute of Life Science Cell Culture Center, Shanghai, China) were cultured in α-minimal essential medium (α-MEM) supplemented with 10% fetal bovine serum and penicillin (100 U/mL) at 37 °C in a 5% CO2 atmosphere.
Cell viability assay
Cell viability assay of trehalose on RAW264.7 cells was assessed by CCK-8 assay according to the manufacturer’s instructions. Cells were cultured in 96-well plates in α-MEM and incubated with different concentrations (0, 25, 50, 100 and 200 mM) of trehalose for 24 hours. CCK-8 reagents were added in each well for 3 hours at 37 °C and the absorbance was measured at 450 nm as an index of cell viability. For each of these experiments at least four parallel measurements were carried out.
Quantitative real-time polymerase chain reaction (qPCR)
Total RNA was extracted from RAW264.7 plated in 6-well plates using RNA-Quick Purification Kit (ES Science, Shanghai, China). HiScriptIIQ RT SuperMix for qPCR (Vazyme Biotech, Nanjing, China) was used to synthesize cDNA from total RNA. The PCR reactions were carried out on a light cycler instrument (LightCycler 480-II, Roche, Mannheim, Germany) using ChamQTM SYBR Colour qPCR Master Mix (Vazyme Biotech). Primers are described in Table 1. All data were normalized to β-actin. The relative quantification of RNA expression was performed using the 2-ΔΔCT method.
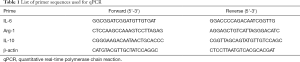
Full table
Statistical analysis
The data are expressed as mean ± standard deviation. One-way ANOVA (and nonparametric) test was used to assess the parameters among the three groups. P value ≤0.05 was considered a significant difference. The data were graphed using GraphPad Prism software version 7 (GraphPad Software Inc., CA, USA).
Results
No rats dropped out before the end of the experiment. No adverse effect of trehalose was observed. The body weights of all the rats increased overtime. However, the rats in group 2 gained much less weight than the other two groups (Figure 1).
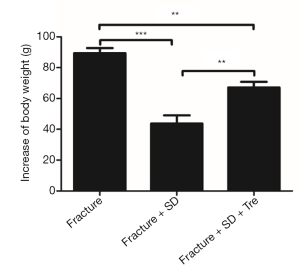
Radiological analysis
X-ray
X-rays taken at 4 weeks after surgery showed that the cortical gap was still apparent in group 2, indicating delayed bone union. By contrast, cortical gap disappeared at the fracture site in group 1 and group 3, and bridging callus formation was observed (Figure 2).
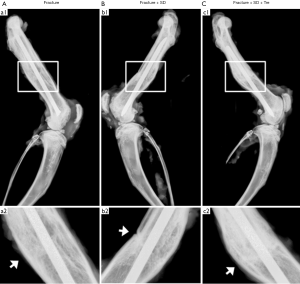
Micro CT
The micro CT images of the callus area in group 2 showed much worse bone reconstruction. While, the callus areas in groups 1 and 3 were reconstructed with intact cortical bone and nearly normal trabecular bone structure (Figure 3A,B,C). The BMD and BV/TV of the fracture callus site in group 2 were significantly less than the values in the other two groups, whereas the Tb.Sp was significantly higher (Figure 3D,E,F). The Tb.Th of group 2 was less than the other two groups, although no significant difference was detected (Figure 3G). The Tb.N in group 2 was significantly less than that of group 1; no significant difference was observed between group 2 and group 3 (Figure 3H).
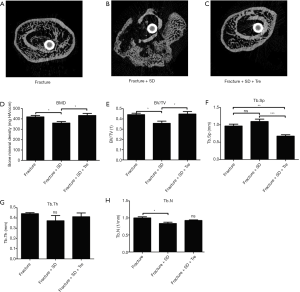
Histological analysis
Groups 1 and 3 showed newly formed bone tissue at the fracture sites. Thick callus consisting of newly formed bone and chondrocytes could be observed in the two groups, resulting in bone union. In contrast, fibrotic tissue and chondrocytes could be observed in group 2, with no bridging bone formation. In group 2 inter-trabecula lacunas existed with mild inflammatory cell infiltration. However, no obvious inflammatory cell infiltration was observed in groups 1 and 3 (Figure 4).
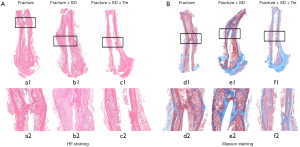
Serum IL-1β and TNF-α
The concentration of serum TNF-α and IL-1β was significantly higher in group 2 compared with the other two groups (Figure 5). No significant difference was observed between groups 1 and 3 (Figure 5).
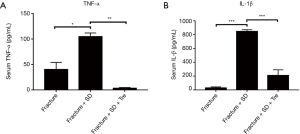
Trehalose affects cell viability of RAW264.7 cells in vitro
Cytotoxicity of trehalose on RAW264.7 cells was assessed by CCK-8 assay. RAW264.7 cell proliferation was significantly decreased with the high concentration of 100 mM trehalose, while the cell viability was not affected by trehalose at low concentrations (Figure 6A).
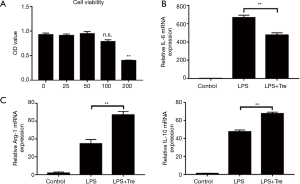
Trehalose regulates M1 and M2 inflammatory macrophage polarization in LPS-stimulated RAW264.7 cells
It is noteworthy that the level of M1 phenotype marker IL-6 increased significantly when the RAW264.7 cells were cultured in LPS medium compared with the control. However, trehalose effectively inhibited LPS-induced IL-6 expression (Figure 6B). By contrast, pro-inflammatory cytokines Arg-1 and IL-10 in M2 macrophages were markedly increased induced by LPS, trehalose still increased these levels when the cells were treated with a combination of LPS and trehalose (Figure 6C).
Discussion
Several studies have reported the effect of SD on bone, mainly focused on the association between SD and BMD (8-12). Both clinical surveys (8-11) and animal laboratory studies (12) showed that short sleep duration resulted in lower BMD and worse bone mass accrual. Several mechanisms have been proposed as possible explanation for the decreased BMD. Higher cortisol levels observed during SD may lead to decreased bone formation and BMD (28). SD also alters the levels of several hormones, proteins, and minerals that may play a pivotal role in bone metabolism, such as osteocalcin, growth hormone, insulin-like-growth factor, parathyroid hormone, and so on (29,30). Bone metabolism also deeply influenced the process of fracture healing. Maybe, SD had adverse effect on fracture healing through the similar mechanisms.
We speculated that the reasons lie in the effect of SD on fracture healing are multifactorial. First, circadian rhythm disruption, which is a well-accepted consequence of SD, would be expected to affect bone formation and bone metabolism by influencing the secretion of several hormones, proteins, and minerals (31). It was described that SD may result in growth hormones deficit (32). Growth hormone is an important regulator of postnatal growth and bone formation (33,34). Vitamin D, an important hormone for bone health has been reported to be associated with SD. Clinical research showed that low serum 25(OH)D level in older men is related with short sleep duration and low sleep efficiency (35). An animal study reported that rats in the SD group had significantly decreased levels of serum 25(OH)D (25). IGF-1, which is important for periosteal expansion was also found a lower concentration with SD patients (36). Whereas, SD may promote the release of cortisol, a catabolic hormone that is associated with decreased BMD and increased fracture risk (37,38). On the other hand, it was reported that the skeleton may be a ductless gland that could produce a hormone, which influences bone mass and bone metabolism (39,40). And, the endocrine function of the bone may be disturbed by SD. Second, SD may lead to negative energy balance. We observed that the body weight gains of rats in fracture + SD group were attenuated. Similar with our results, previous study showed that SD rats lost 15% of their body weight, whereas, the food intake increased 2 to 3 folds (41). The negative energy balance may have adverse effect on bone formation. It is reported that negative energy may have inverse effect on osteocalcin concentrations (42). Another study showed that the rats with negative energy balance in the SD group had decreased bone mass (25). Other examples of hypermetabolism, such as induced by thyroid hormone administration also contributed to osteopenia (43). Third, SD may result in systemic inflammation (44). This inflammation may be related to decreased bone formation and osteoporosis. An animal study using a rat model of bone loss induced by SD observed upregulation of proinflammatory mediators such as serum IL-1β, cyclooxygenase (COX)-2, and TNF-α (45). Other studies have demonstrated high levels of circulating cytokines and cortisol were associated with increased fracture risk, altered bone metabolism, and low BMD (46,47). The present study also showed SD significantly upregulated serum IL-1β and TNF-α, which is consistent with previous studies.
Trehalose may promote bone fracture healing by suppressing systemic inflammation in the present study. In fact, previous studies demonstrated that trehalose may inhibit the inflammatory cascade (48). The present study also demonstrated that trehalose treatment suppresses pro-inflammatory cytokines IL-6 release by enhancing M2 macrophage polarization (mediated by increases in Arg-1 and IL-10 expression) in RAW264.7 cells following LPS stimulation. Although a relative predominance of M1:M2 cells in callus is still not understood (49), induction of M2 macrophages was found to significantly enhance bone formation, which appears to be good to fracture healing and successful regeneration (50). Shifting the inflammatory reaction towards M2 upon treatment of trehalose could improve the healing outcome and prevent delayed bone regeneration resulted from SD.
There are several limitations to the present study. First, no biomechanical study was performed to assess the fracture healing quality in the present study. Second, the period of 3 weeks SD was relatively short, and the extent of restrictive sleep duration was not considered. Third, the effect of oral administration trehalose was not investigated in the present study, although a recent study showed that trehalose would be digested into glucose molecules in mammalian digestive tract, and would lose effectiveness when taken orally. Different groups of varied sleep duration should be established in the future studies. And, the exact mechanism of trehalose needs more investigation.
In conclusion, this study revealed that SD had adverse effect on bone fracture healing. Sleep quality and duration should be improved to accelerate recovery of the patients with bone fracture. Trehalose may be good to the bone fracture ones with sleep problem by suppressing inflammation and enhancing M2 polarization.
Acknowledgments
We thank the ones who give kindly advice to our study.
Funding: This work was supported by National Science Foundation of China (NSFC, 81802196), Natural Science Foundation of Jiangsu Province, China (BK20180127), Projects of International Cooperation and Exchanges NSFC [81420108021], Key Program of NSFC [81730067], Excellent Young Scholars NSFC [81622033], Jiangsu Provincial Key Medical Center Foundation, Jiangsu Provincial Medical Outstanding Talent Foundation, Jiangsu Provincial Medical Youth Talent Foundation and Jiangsu Provincial Key Medical Talent Foundation.
Footnote
Conflicts of interest: The authors have no conflicts of interest to declare.
Ethical Statement: All animals procedures were accordance with the guidelines and approved by the Ethics Committee of Drum Tower Hospital, Medical School of Nanjing University, Nanjing, China (Ethical approval number: 20171002).
References
- Worthman CM, Brown RA. Sleep budgets in a globalizing world: Biocultural interactions influence sleep sufficiency among Egyptian families. Soc Sci Med 2013;79:31-9. [Crossref] [PubMed]
- Bonnet MH, Arand DL. We are chronically sleep deprived. Sleep 1995;18:908-11. [Crossref] [PubMed]
- Yang CK, Kim JK, Patel SR, et al. Age-related changes in sleep/wake patterns among Korean teenagers. Pediatrics 2005;115:250-6. [Crossref] [PubMed]
- Heianza Y, Kato K, Fujihara K, et al. Role of sleep duration as a risk factor for Type 2 diabetes among adults of different ages in Japan: the Niigata Wellness Study. Diabet Med 2014;31:1363-7. [Crossref] [PubMed]
- Dominguez-Rodriguez A, Abreu-Gonzalez P. The link between sleep duration and inflammation: Effects on cardiovascular disease. Int J Cardiol 2014;173:600-1. [Crossref] [PubMed]
- McEwen BS. Sleep deprivation as a neurobiologic and physiologic stressor: Allostasis and allostatic load. Metabolism 2006;55:S20-3. [Crossref] [PubMed]
- Troxel WM, Buysse DJ, Matthews KA, et al. Sleep symptoms predict the development of the metabolic syndrome. Sleep 2010;33:1633-40. [Crossref] [PubMed]
- Fu X, Zhao X, Lu H, et al. Association between sleep duration and bone mineral density in Chinese women. Bone 2011;49:1062-6. [Crossref] [PubMed]
- Yen CM, Kuo CL, Lin MC, et al. Sleep disorders increase the risk of osteoporosis: a nationwide population-based cohort study. Sleep Med 2014;15:1339-44. [Crossref] [PubMed]
- Casazza K, Hanks LJ, Fernandez JR. Shorter sleep may be a risk factor for impaired bone mass accrual in childhood. J Clin Densitom 2011;14:453-7. [Crossref] [PubMed]
- Zakhem E, El Hage R, Zunquin G, et al. Sleep quality is a determinant of hip bone mineral density in a group of young Lebanese men. J Med Liban 2014;62:213-6. [PubMed]
- Xu X, Liang W, Chen L, et al. Effects of chronic sleep deprivation on bone mass and bone metabolism in rats. J Orthop Surg Res 2016;11:87. [Crossref] [PubMed]
- Claes L, Recknagel S, Ignatius A. Fracture healing under healthy and inflammatory conditions. Nat Rev Rheumatol 2012;8:133-43. [Crossref] [PubMed]
- Cadet ER, Yin B, Schulz B, et al. Proximal humerus and humeral shaft nonunions. J Am Acad Orthop Surg 2013;21:538-47. [PubMed]
- Gaston MS, Simpson AH. Inhibition of fracture healing. J Bone Joint Surg Br 2007;89:1553-60. [Crossref] [PubMed]
- Brown BN, Londono R, Tottey S, et al. Macrophage phenotype as a predictor of constructive remodeling following the implantation of biologically derived surgical mesh materials. Acta Biomater 2012;8:978-87. [Crossref] [PubMed]
- Richards AB, Krakowka S, Dexter LB, et al. Trehalose: a review of properties, history of use and human tolerance, and results of multiple safety studies. Food Chem Toxicol 2002;40:871-98. [Crossref] [PubMed]
- Kang XL, Shen H. Pigmentation of Skin Graft Is Improved by Cryopreservation of Human Skin With Trehalose. J Oral Maxillofac Surg 2012;70:1464-72. [Crossref] [PubMed]
- Castillo K, Nassif M, Valenzuela V, et al. Trehalose delays the progression of amyotrophic lateral sclerosis by enhancing autophagy in motoneurons. Autophagy 2013;9:1308-20. [Crossref] [PubMed]
- Sergin I, Evans T, Zhang X, et al. Exploiting macrophage autophagy-lysosomal biogenesis as a therapy for atherosclerosis. Nat Commun 2017;8:15750. [Crossref] [PubMed]
- Tang Q, Zheng G, Feng Z, et al. Trehalose ameliorates oxidative stress-mediated mitochondrial dysfunction and ER stress via selective autophagy stimulation and autophagic flux restoration in osteoarthritis development. Cell Death Dis 2017;8:e3081. [Crossref] [PubMed]
- He Q, Wang Y, Lin W, et al. Trehalose alleviates PC12 neuronal death mediated by lipopolysaccharide-stimulated BV-2 cells via inhibiting nuclear transcription factor NF-κB and AP-1 activation. Neurotox Res 2014;26:430-9. [Crossref] [PubMed]
- Tägil M, Mcdonald M, Morse A, et al. Intermittent PTH(1-34) does not increase union rates in open rat femoral fractures and exhibits attenuated anabolic effects compared to closed fractures. Bone 2010;46:852-9. [Crossref] [PubMed]
- Schindeler A, Yu N, Cheng T, et al. Local Delivery of the Cationic Steroid Antibiotic CSA-90 Enables Osseous Union in a Rat Open Fracture Model of Staphylococcus aureus Infection. J Bone Joint Surg Am 2015;97:302-9. [Crossref] [PubMed]
- Xu X, Wang L, Zhang Y, et al. Effects of chronic sleep deprivation on glucose homeostasis in rats. Sleep Biol Rhythms 2016;14:321-8. [Crossref] [PubMed]
- Ma C, Wu G, Wang Z, et al. Effects of chronic sleep deprivation on the extracellular signal-regulated kinase pathway in the temporomandibular joint of rats. PLoS One 2014;9:e107544. [Crossref] [PubMed]
- Morgan EF, Mason ZD, Chien KB, et al. Micro-computed tomography assessment of fracture healing: relationships among callus structure, composition, and mechanical function. Bone 2009;44:335-44. [Crossref] [PubMed]
- Weinstein RS, Jilka RL, Parfitt AM, et al. Inhibition of osteoblastogenesis and promotion of apoptosis of osteoblasts and osteocytes by glucocorticoids. Potential mechanisms of their deleterious effects on bone. J Clin Invest 1998;102:274-82. [Crossref] [PubMed]
- Blumsohn A, Herrington K, Hannon RA, et al. The effect of calcium supplementation on the circadian rhythm of bone resorption. J Clin Endocrinol Metab 1994;79:730-5. [PubMed]
- Chapotot F, Gronfier C, Spiegel K, et al. Relationships between intact parathyroid hormone 24-hour profiles, sleep-wake cycle, and sleep electroencephalographic activity in man. J Clin Endocrinol Metab 1996;81:3759-65. [PubMed]
- Antle MC, Mistlberger RE. Circadian clock resetting by sleep deprivation without exercise in the Syrian hamster. J Neurosci 2000;20:9326-32. [Crossref] [PubMed]
- Lieb K, Reincke M, Riemann D, et al. Sleep deprivation and growth-hormone secretion. Lancet 2000;356:2096-7. [Crossref] [PubMed]
- Andreassen TT, Oxlund H. The Influence of Combined Parathyroid Hormone and Growth Hormone Treatment on Cortical Bone in Aged Ovariectomized Rats. J Bone Miner Res 2000;15:2266-75. [Crossref] [PubMed]
- Grubbe MC, Thomsen JS, Nyengaard JR, et al. Growth hormone mitigates loss of periosteal bone formation and muscle mass in disuse osteopenic rats. J Musculoskelet Neuronal Interact 2014;14:473-83. [PubMed]
- Massa J, Stone K, Wei E, et al. Vitamin D and Actigraphic Sleep Outcomes in Older Community-Dwelling Men: The MrOS Sleep Study. Sleep 2015;38:251-7. [Crossref] [PubMed]
- Nieminen P, Löppönen T, Tolonen U, et al. Growth and biochemical markers of growth in children with snoring and obstructive sleep apnea. Pediatrics 2002;109:e55. [Crossref] [PubMed]
- Mussolino ME. Depression and hip fracture risk: the NHANES I epidemiologic follow-up study. Public Health Rep 2005;120:71-5. [Crossref] [PubMed]
- Bostock S, Steptoe A. Influences of early shift work on the diurnal cortisol rhythm, mood and sleep: Within-subject variation in male airline pilots. Psychoneuroendocrinology 2013;38:533-41. [Crossref] [PubMed]
- Vervloet MG, Massy ZA, Brandenburg VM, et al. Bone: a new endocrine organ at the heart of chronic kidney disease and mineral and bone disorders. Lancet Diabetes Endocrinol 2014;2:427-36. [Crossref] [PubMed]
- Guntur AR, Rosen CJ. Bone as an endocrine organ. Endocr Pract 2012;18:758-62. [Crossref] [PubMed]
- Everson CA, Szabo A. Recurrent restriction of sleep and inadequate recuperation induce both adaptive changes and pathological outcomes. Am J Physiol Regul Integr Comp Physiol 2009;297:R1430-40. [Crossref] [PubMed]
- Jürimäe J, Rämson R, Mäestu J, et al. Interactions between adipose, bone, and muscle tissue markers during acute negative energy balance in male rowers. J Sports Med Phys Fitness 2011;51:347-54. [PubMed]
- Yamamoto M, Markatos A, Seedor J, et al. The effects of the aminobisphosphonate alendronate on thyroid hormone-induced osteopenia in rats. Calcif Tissue Int 1993;53:278-82. [Crossref] [PubMed]
- Irwin MR. Why sleep is important for health: a psychoneuroimmunology perspective. Annu Rev Psychol 2015;66:143-72. [Crossref] [PubMed]
- Smith BJ, Lerner MR, Bu SY, et al. Systemic bone loss and induction of coronary vessel disease in a rat model of chronic inflammation. Bone 2006;38:378-86. [Crossref] [PubMed]
- Bedford JL, Barr SI. The relationship between 24-h urinary cortisol and bone in healthy young women. Int J Behav Med 2010;17:207-15. [Crossref] [PubMed]
- Gertz ER, Silverman NE, Wise KS, et al. Contribution of serum inflammatory markers to changes in bone mineral content and density in postmenopausal women: a 1-year investigation. J Clin Densitom 2010;13:277-82. [Crossref] [PubMed]
- Minutoli L, Altavilla D, Bitto A, et al. Trehalose: a biophysics approach to modulate the inflammatory response during endotoxic shock. Eur J Pharmacol 2008;589:272-80. [Crossref] [PubMed]
- Harris RA. Spatial, Temporal, and Functional Aspects of Macrophages during "The Good, the Bad, and the Ugly" Phases of Inflammation. Front Immunol 2014;5:612. [Crossref] [PubMed]
- Schlundt C, El Khassawna T, Serra A, et al. Macrophages in bone fracture healing: Their essential role in endochondral ossification. Bone 2018;106:78-89. [Crossref] [PubMed]