Review of robotic-assisted surgery: what the future looks like through a spine oncology lens
Introduction
Rapid technological advancements have allowed for new and improved devices within the medical field. Specifically, the development of FDA-approved robotic-assisted surgical devices has helped create a push for improved patient care and outcomes (1). Conceptually, most robotic technology seeks to assist the human operator in reducing variability and improving accuracy of surgical maneuvers.
Historically, fields such as surgical oncology, urology, and gynecology/oncology have led the way in developing robotic assistance. In these settings, surgical robots have allowed improved optical visualization and improved surgical maneuvering for retraction, exposure, and resection of tissue (2-5). However, robotics in spine surgery is a comparatively immature technology, and the use of robotic assistance mainly surrounds assistance with instrumentation. Examples of demonstrated benefits include improved accuracy, decreased radiation exposure, and reduced early postoperative complications and adverse events (6-14). Robotic-assisted spinal surgery requires limited exposure and has therefore been associated with quicker recovery and a reduced length of stay (10,12-14). Advancements have also led to success of robotic surgical systems in spinal tumors such as paravertebral schwannomas (15-17) and presacral tumors (18), and can also offer advantages in vertebral augmentation (18,19), biopsies (18-20), and osteotomies (21). However, robotics in spine surgery often results in longer operative times due to device set-up, not to mention the obvious downside of capital equipment cost (9,13). Although the scope of robotic maneuvers in spine surgery is currently limited, the inherent nature of the technology unlocks myriad other applications and roles. The purpose of this manuscript is to define and explore what some of these future applications may look like by understanding both the history and capabilities of the systems.
Robotics in non-orthopedic oncology
Pelvic and intraabdominal surgery
Robotic technology is commonly implemented in gynecological oncology, specifically in the treatment of endometrial and cervical cancer (4). The most heavily studied platform is the da Vinci Surgical System. Analyses of the literature in this setting has suggested that robotic surgery is associated with less blood loss, shorter hospital stay, fewer complications, and fewer transfusions than laparoscopy for patients with endometrial cancer and complex myomectomies, but with the caveat of increased operative time and increased cost (22-24).
Similarly, robotics in urology offers a useful niche and solution for challenging open or laparoscopic procedures. Robotic arms can morselize samples and extract them through their respective ports, and literature has shown that robot-assisted partial nephrectomy is a safe, minimally invasive operation with excellent functional outcomes and few perioperative complications when performed by an experienced surgeon (3,25,26). Pediatric urology has also gained traction in adopting robotic assistance for removal of suspicious or cancerous genitourinary tract lesions, despite the cost burden of obtaining and maintaining the capital equipment (5).
Other cases of the da Vinci system in general surgery include assistance in liver resections for benign and malignant tumors (2,5), where the robot provides a safe, minimally invasive technique option. Robotics have also been documented in the successful resection of other challenging tumors including hilar cholangiocarcinomas (27), malignant and benign pancreatic tumors (28), and adrenal tumors (29).
Otolaryngology
The da Vinci surgical system also offers utility in the treatment of tumors of the ears, nose, and throat. A recent case-study reports that the da Vinci robot assisted in the first case of robotic-assisted excision of a cystic hygroma in a patient younger than 2 years old (30). The robot provided greater range of motion and reduced tremor in a small area as well as an improved cosmetic outcome due to its minimally invasive nature. Similarly, robotic-assisted transaxillary thyroidectomy has shown to be a feasible choice for the treatment of benign nodules (31,32). In addition to smoother motion with use of robotic arms, robotic assisted surgery has improved otolaryngic surgical approaches such as the transoral approach, making once difficult-to-access tumors more accessible and safer to remove (33,34).
Cardiothoracic surgery
While video-assisted thoracoscopic surgery (VATS) has been the mainstay minimally invasive method to diagnose and treat thoracic medical conditions, the da Vinci surgical system has since allowed for the introduction of robot-assisted thoracoscopic surgery (RATS). RATS has been demonstrated to be a safe and effective treatment option with better short-term outcomes than VATS for the treatment of non-small cell lung cancer (35). Furthermore, robotic pulmonary lobectomy has shown to have excellent lymph node removal rates with minimal morbidity (35-37). The da Vinci system has also demonstrated efficacy in the removal of benign primary cardiac tumors, such as a right ventricle intracardiac lipoma (38) and papillary fibroelastoma of mitral chordae (38,39), and even facilitates sternal-sparing access to the heart (40). Though cost is still a burden, robotics continues to be indicated in a breadth of thoracic surgeries and we can expect the applications to broaden with more experience and published data.
Summary of robotics in visceral organ tumor surgery
Whereas traditional laparoscopic techniques only offer 2D optics and lack depth perception, robotics allows enhanced optics, magnification, and a variety of arms for retraction and manipulation of tissues. Another benefit is that scalability of motion allows large hand movements to translate to smoother and finer surgical maneuvers such as in suturing and tumor resection (40,41). It follows that more motion control, improved optics and multi-arm retraction should lead to better control of surgical margins and outcomes in tumor surgery such as local recurrence. However, there is a lack of detailed evidence comparing robotic-assisted general surgery to more traditional procedures in this regard.
Robotics in spine surgery thus far
Robotics offers significant utility in spine surgery, predominantly as an assist to placing pedicle screw instrumentation (13). Posterior pedicle screw instrumentation is a common and important component of many spine procedures, and inaccuracy of placement despite use of conventional fluoroscopy can result in neurovascular injury or construct failure (42). Therefore, a demand for application of surgical robots has led to the recent surge in their use.
Surgical robotics have been categorized into three categories by Nathoo et al., and can be applied to robotic-assisted spine surgery (43). The first involves supervisory systems that involve pre-operative planning by the surgeon and input into a synchronized system that communicates and directs the robot to perform in a fully automated fashion. The surgeon is still required to be present for the entirety of the procedure and can supervise the robot in case of malfunction or misdirection requiring fine-tuning. The second category describes a robot-surgeon interaction such that a controller is manipulated directly by the surgeon to control the surgical instruments held by the robot. This system is exemplified by the da Vinci System. The third category is a shared system relationship. Preoperative planning by the surgeon and templating imaging allows the robot to locate itself to a preset location, but requires the surgeon to insert and manipulate the surgical instruments. This latter method is the most common system used in spinal pedicle screw placement and is exemplified by the Mazor (Medtronic, Minneapolis, MN, USA), ROSA (MedTech Surgical, Newark, NJ, USA), and Excelsius GPS (Globus Medical, Audubon, PA, USA) systems (43,44) (Table 1).
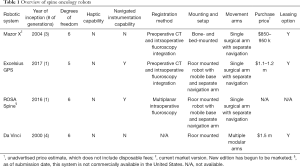
Full table
Early reviews comparing freehand to fluoroscopic-guided or robot-assisted pedicle screw placement lacked randomized controlled trials (RCT), leaving insufficient evidence to recommend one over the other (45,46). Recently, a meta-analysis of 6 RCTs demonstrated improved Grade A accuracy as described by the Gerztbein-Robbins classification, as well as fewer proximal facet joint violations with robot assistance (47). Another recent systematic review including a mix of study types also suggested superior accuracy with robotic assistance, although no specific analysis was performed (13). Other studies have demonstrated robot-assisted screw accuracies of 84–100% (12,14,48).
Radiation exposure is of utmost concern to spine surgeons attempting to minimize exposure to both patients and the surgical staff, and robotic-assisted instrumentation has been linked to reduced radiation exposure. A prospective RCT demonstrated 13.3 seconds of radiation per screw in the fluoroscopic-assisted group versus only 3.5 seconds per screw in the robot-assisted group, a nearly four-fold reduction in radiation exposure, and similar findings have been demonstrated in other studies (14,49,50). Freehand techniques offer similarly low rates of radiation, although operative times have almost unanimously been longer using robotic assistance (9,13). Unlike robotic-assisted instrumentation, fluoroscopic confirmation of correct freehand screw placement is recommended, which increases radiation to the patient without a significant increase in overall radiation exposure to the surgical staff. Overall, use of preoperative and postoperative imaging minimizing necessity for intraoperative fluoroscopy, either with freehand or robotic-assisted techniques, can reduce radiation exposure to the surgical staff (9).
Other applications
For nearly three decades, spine surgery has employed the intraoperative assistance of robots and several now offer more unique applications. As opposed to traditional CT/fluoroscopy based platforms for preoperative planning, the SPINEBOT, Cooperative Robotic Assistant (CoRA), and Innomotion allow for a multi-view approach for trajectory planning using MRI-guided imaging (20,51). Similarly, the Neuroglide and robot spinal surgical system (RSSS) were created with an infrared optical tracker navigation system (52,53).
Furthermore, robotic-assisted radiation delivery systems offer relevant applications to neural-axis tumor surgery. The Cyberknife (Accuray, Inc., Sunnyvale, CA, USA) is a fully robotic radiation delivery system for tumor ablation offering a six-axis robotic arm and custom molds to immobilize the spine, while maintaining 1 mm precision (54,55). There are no current offerings that implement instruments for tumor excision or spine instrumentation. However, the Cyberknife can deliver radiation while minimizing effects to adjacent tissue, and offers intriguing technology to identify tumor tissue that may be implemented in future systems. Novalis (BrainLAB, Heimstetten, Germany) also offers single-fraction radiosurgery to improve radiation delivery and uses stereoscopic imaging technology to maintain high-precision (56).
Drawbacks of robotic systems
Implementation of innovative robotic systems encompasses various challenges. Facility concerns involve capital cost and the financial burden of maintenance. Although each robotic system involves various costs, the three FDA-approved systems each cost more than $850,000 and necessitate more than $2,000 in annual disposable fees (13). Some of the fees are offset by leasing options or no-cost placement of a robot in centers performing high case volumes, although the long-term true cost of these arrangements is unknown. An additional complexity is that having a robotic system offers a marketing avenue to increase overall case volume and many centers use these systems as a loss leader.
Much debate surrounding the efficacy of robot-assisted surgery surrounds operative time and the inherent “loss of full control” by the surgeon. The idea of “loss of control” instills concerns in patients who rely on surgeon experience and confidence with established techniques and tools. However, patient-, floor-, or table-mounted robots such as the ROSA, Mazor, and Excelsius allow for a shared-control model with low likelihood of failure and multiple safeguards and emergency stops. And while longer operative times are generally linked to worse patient outcomes and increased facility costs, much of the available literature is based on data from surgeons with comparatively limited experience with robotic systems. Operative time can be rapidly reduced with streamlined steps and improved operator experience by the surgical team (20). The steep learning curve places further limitations on fully implementing robotic systems, and can take more than 30 cases, even by experienced surgeons (57). Finally, much like other novel literature, current studies of robotic-assisted spine surgery should be closely criticized for any conflicts of interest.
Application of robotics in spine tumor surgery
After the lung and liver, bone is the most common site for metastatic spread of cancer (58,59), with prostate and breast cancer making up more than two-thirds of these metastases (60). The axial skeleton is the most common bony site, and metastases in this location may cause significant neurologic or structural morbidity for a significant proportion of our aging population (60,61). While less common, primary bone tumors of the spine, malignant or benign, bring their own unique issues and challenges with regard to localization, visualization, and obtaining surgical margins. Often, surgical care for patients with neoplastic lesions of the spine is complex, individualized, and multidisciplinary. Robotics may be an important and useful tool in accomplishing surgical goals in this setting.
Robotics have been increasingly useful in the excision of spine tumors at varied sites. In one case report, a paraspinal left-sided neurogenic tumor was resected using the da Vinci Xi system where one arm was used to retract the lung and allow for posterior mediastinal visualization (15). Similarly, another patient with a larger paraspinal mass spanning T9 to T11 underwent successful robot-assisted resection using this device’s angulated arms and excellent 3D imaging. A third case of a paraspinal schwannoma at L4 was also successfully resected, requiring displacement of the ureter and psoas muscle, demonstrating the impact of the da Vinci’s dexterous arms, increased optical magnification (up to 10×), and 3D resolution for accurate dissections at otherwise unachievable angles and spaces (16,62).
Preoperative planning
Preoperative planning is a crucial step in the robotic workflow before implementing such a system in spine tumor surgery (Figure 1). While plain radiographs only provide two-dimensional information, thin-cut CT imaging facilitates high-quality image registration for successful robotic implementation (19), and MRI-based modalities may continue to improve the precision of planning and executing surgery around soft tissues and neurovascular elements. Conceptually, improved imaging techniques allow for the planning of custom patient-specific trajectories for implants, biopsies, or other percutaneous interventions such as cryoablation (63,64). Additionally, rapid interpretation of data by surgical robots could theoretically improve the likelihood of successful tumor resection. Addition of classification systems into the software packages of these robots could offer meaningful information to the surgeon. For example, the Weinstein, Boriani, Biagini (WBB) classification system describes vertebral tumor involvement and divides vertebrae into 12 zones circumferentially and 6 various zones of depth (radially) from extraosseous to intradural (65). Preoperative or intraoperative processing of the WBB classification and application to spine tumors by robotic systems could allow for more accurate resections during vertebrectomies, sagittal resections, or posterior arch resections depending on the radiating zones of tumor (66). Finally, surgical teams can also use 3D modeling and printing techniques to plan extent of resections of spine tumors, which when implemented with robotic systems may offer improved precision and accuracy (67).
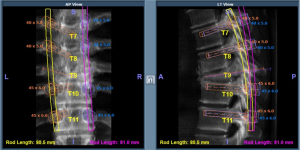
Resection guidance
Robot-assisted spine surgery has been used to guide resection for primary bone tumors in the spine. Bederman et al. reported a case of an en bloc sacrectomy for a sacral osteosarcoma (21). Using the Renaissance System in conjunction with preoperative CT, the robotic system assisted in performing iliac osteotomy and sacroiliac joint disarticulation via drilling of planned pilot holes. Additionally, Oh et al. reported on a series of robotically-assisted resections of presacral tumors involving sacral bone, demonstrating the feasibility of such robotic systems in cases of local tumor that has expanded to adjacent structures (18). Finally, robotics in spine tumor surgery has been well-demonstrated in thoracic paravertebral tumors, demonstrating the usefulness of robotics in surgical techniques that require a multidisciplinary approach (16). These reports demonstrate the ability of robotic systems to assist in en bloc procedures about the spine in settings where resection precision and preservation of normal tissues are critical to oncologic and patient outcomes.
Reconstruction
In addition to the benefits in tumor resection, robotics may offer some unique advantages in reconstruction. Typical reconstruction following spinal tumor surgery may involve posterior segmental instrumentation, spanning synthetic cages, and biologic bone grafting, host-related challenges due to complex anatomy, tumor extent, and poor bone quality are hurdles which must be overcome. Additionally, prior surgery, chemotherapy, and/or radiotherapy treatment may create distorted or fibrosed local anatomy (68). These challenges may be best addressed by a less invasive, precise instrumentation modality such as robotics. This is especially germane in the modern era, where less-invasive surgical options and prognosis-shifting improvements in medical therapies have opened the door to surgical options for patients who otherwise would have not been considered operative candidates (69).
As previously mentioned, precise placement of pedicle screws is an important function of robotics in spine surgery procedures where capturing ideal bone corridors is important. Solomiichuk et al. assessed the accuracy of the SpineAssist system (Mazor Robotics, Caesarea, Israel) in thoracolumbar metastases (69,70). Retrospectively, the authors evaluated 70 patients with metastatic spinal disease requiring spine surgery, and used a matched-cohort to compare traditional anatomic placement of pedicle screws. Their results suggested no statistical difference in accuracy or radiation time between the two groups, although one misplaced screw in the traditional method using fluoroscopy required a revision surgery. Similarly, Hu et al. published their experience with robotics in spine tumor surgery, reporting on 9 consecutive patients with thoracolumbar spinal tumor burden with concurrent or impending neural compression (19). Using pre-operative thin-slice CT imaging, pedicle screw and vertebral augmentation trajectories were mapped out and used to accurately perform indicated procedures. There were no documented peri-operative complications. Blood loss and operative time were within typical ranges for the surgical team. Finally, spinal robotics may also be useful in hypervascular primary bone tumors as well as metastatic renal cell and thyroid carcinomas since robotic-assisted systems may limit exposures during screw placement and therefore minimize blood loss (71).
Intradural applications
Robotics has been described for intradural applications, namely for intracranial pathology (72). The ROSA system has been tool for various neurosurgical procedures, as described by Lefranc et al. (73) in adults, and De Benedictis (74) in children. However, as our knowledge of intradural spine tumors increases and its relationship to the surrounding anatomy becomes more apparent, we can expect other robotic systems to allow surgeons to adequately address this niche. To date, there is no published literature describing the appropriate application of these newly modeled robotic systems for intradural spine tumors, but increasing research in this field may expand the indications for surgery on patients who may have been deemed poor candidates in the past.
Future directions
Enhanced haptic robotic feedback is potentially valuable in allowing the surgeon to execute the preoperative plan. Currently, most applications in spine involve haptic feedback preventing deviation during pedicle screw placement. For example, the Mazor X screen will flash red and provide a “beep” when it senses too much pressure onto its arm, necessitating the user to re-register the system and causing a surgical delay. In contrast, robots in the arthroplasty arena provide a more rigid environment with consistent beeping, vibrations, and screen changes to alert and maintain the surgeon within the planned trajectory of bony cuts without necessitating re-registration (75). Implementing such systems into robotic spine systems would be beneficial with handheld tools such as a burr in achieving wide margins during tumor resections or during challenging spinal decompressions. Theoretically any surgical instrument can be linked to haptic robotics, and with increasingly complex software algorithms we can expect feedback systems to similarly become even more tactile and realistic. The ultimate limit of this technology is far removed from the current state, and one can imagine futuristic applications such as remote telesurgery as the systems become more reliable and precise.
Current robotic arms are rudimentary, but modularity and optionality of sensors and tools is expected to expand. For example, identifying tumor margins often require microscopic evaluation. Evolution of robotic systems would benefit spine tumor surgery by including robotic arms that can accommodate modular ends with special sensors to detect tumor tissue, adjacent bone, dura, nerves, and vessels. Alternatively, intravenous radio-markers can allow for detection of residual tumor using optical or fluorescent tracers that can further amplify haptic feedback to allow for an efficient and safe surgical environment. Such fluorescent-guided surgery using activatable probes to identify cancerous tissue and tumor margins has been successful in other oncologic fields, and its application to robot-assisted spine tumor surgery would be beneficial (76).
Since the human arm has 7 degrees of freedom and three joints, the future of robotic surgical systems also involves expansion of capabilities in complex motions which manipulate the human host. For example, by linking to segmental instrumentation, robots may one day gain the ability to precisely manipulate individual vertebral segments, allowing for controlled osteotomy closure or deformity correction.
Conclusions
Robotic-assisted surgical systems are in their infancy compared to their potential, but have demonstrated clear benefits when compared to traditional open techniques. In caring for spine tumors, the ultimate use of robotics surrounds the ability of the system to identify and understand distorted spinal anatomy and lend detailed information that the operating surgeon can use to provide personalized care to each patient. As technology continues to evolve, we can expect more streamlined, interactive, and realistic robotic systems.
Acknowledgments
None.
Footnote
Conflicts of Interest: MW Colman has relationships with the following: K2M: educational speaking; Alphatec: Consulting/design; Spinal Elements: Consulting/design; Orthofix: educational speaking; Medicrea: Consulting; AO Spine: fellowship support. The other authors have no conflicts of interest to declare.
References
- Website [Internet]. [cited 2019 Jan 12]. Available online: www.fda.gov/MedicalDevices/ProductsandMedicalProcedures/SurgeryandLifeSupport/ComputerAssistedSurgicalSystems/default.htm
- Zou H, Luo L, Xue H, et al. Preliminary experience in laparoscopic resection of hepatic hydatidectocyst with the Da Vinci Surgical System (DVSS): a case report. BMC Surg 2017;17:98. [Crossref] [PubMed]
- Novara G, La Falce S, Kungulli A, et al. Robot-assisted partial nephrectomy. Int J Surg 2016;36:554-9. [Crossref] [PubMed]
- Sinno AK, Fader AN. Robotic-assisted surgery in gynecologic oncology. Fertil Steril 2014;102:922-32. [Crossref] [PubMed]
- Croner R, Perrakis A, Grützmann R, et al. Robotic-Assisted Liver Surgery. Zentralbl Chir 2016;141:154-9. [PubMed]
- Onen MR, Naderi S. Using robotic systems in spine surgery. Turk Neurosurg [Internet]. 2013. Available online: http://dx.doi.org/ [Crossref]
- Devito DP, Kaplan L, Dietl R, et al. Clinical acceptance and accuracy assessment of spinal implants guided with SpineAssist surgical robot: retrospective study. Spine (Phila Pa 1976) 2010;35:2109-15. [Crossref] [PubMed]
- Fan Y, Du JP, Liu JJ, et al. Accuracy of pedicle screw placement comparing robot-assisted technology and the free-hand with fluoroscopy-guided method in spine surgery: An updated meta-analysis. Medicine 2018;97:e10970. [Crossref] [PubMed]
- Yu L, Chen X, Margalit A, et al. Robot-assisted vs freehand pedicle screw fixation in spine surgery - a systematic review and a meta-analysis of comparative studies. Int J Med Robot 2018;14:e1892. [Crossref] [PubMed]
- Fan Y, Du J, Zhang J, et al. Comparison of Accuracy of Pedicle Screw Insertion Among 4 Guided Technologies in Spine Surgery. Med Sci Monit 2017;23:5960-8. [Crossref] [PubMed]
- Ringel F, Stüer C, Reinke A, et al. Accuracy of robot-assisted placement of lumbar and sacral pedicle screws: a prospective randomized comparison to conventional freehand screw implantation. Spine 2012;37:E496-501. [Crossref] [PubMed]
- Kantelhardt SR, Martinez R, Baerwinkel S, et al. Perioperative course and accuracy of screw positioning in conventional, open robotic-guided and percutaneous robotic-guided, pedicle screw placement. Eur Spine J 2011;20:860-8. [Crossref] [PubMed]
- Ghasem A, Sharma A, Greif DN, et al. The Arrival of Robotics in Spine Surgery: A Review of the Literature. Spine 2018;43:1670-7. [PubMed]
- Hyun SJ, Kim KJ, Jahng TA, et al. Minimally Invasive Robotic Versus Open Fluoroscopic-guided Spinal Instrumented Fusions: A Randomized Controlled Trial. Spine 2017;42:353-8. [Crossref] [PubMed]
- Pacchiarotti G, Wang MY, Kolcun JP, et al. Robotic paravertebral schwannoma resection at extreme locations of the thoracic cavity. Neurosurg Focus 2017;42:E17. [Crossref] [PubMed]
- Yang MS, Kim KN, Yoon DH, et al. Robot-assisted resection of paraspinal Schwannoma. J Korean Med Sci 2011;26:150-3. [Crossref] [PubMed]
- Kufeld M, Wowra B, Muacevic A, et al. Radiosurgery of Spinal Meningiomas and Schwannomas. Technol Cancer Res Treat 2012;11:27-34. [Crossref] [PubMed]
- Oh JK, Yang MS, Yoon DH, et al. Robotic resection of huge presacral tumors: case series and comparison with an open resection. J Spinal Disord Tech 2014;27:E151-4. [Crossref] [PubMed]
- Hu X, Scharschmidt TJ, Ohnmeiss DD, et al. Robotic assisted surgeries for the treatment of spine tumors. Int J Spine Surg 2015. [Crossref] [PubMed]
- Bertelsen A, Melo J, Sánchez E, et al. A review of surgical robots for spinal interventions. Int J Med Robot 2013;9:407-22. [Crossref] [PubMed]
- Bederman SS, Lopez G, Ji T, et al. Robotic guidance for en bloc sacrectomy: a case report. Spine 2014;39:E1398-401. [Crossref] [PubMed]
- Ran L, Jin J, Xu Y, et al. Comparison of robotic surgery with laparoscopy and laparotomy for treatment of endometrial cancer: a meta-analysis. PLoS One 2014;9:e108361. [Crossref] [PubMed]
- Corrado G, Vizza E, Legge F, et al. Comparison of Different Surgical Approaches for Stage IB1 Cervical Cancer Patients: A Multi-institution Study and a Review of the Literature. Int J Gynecol Cancer 2018;28:1020-8. [Crossref] [PubMed]
- Kim S, Luu TH, Llarena N, et al. Role of robotic surgery in treating fibroids and benign uterine mass. Best Pract Res Clin Obstet Gynaecol 2017;45:48-59. [Crossref] [PubMed]
- Ge GJ, Ding GQ, Zhao WP, et al. Robot-assisted partial nephrectomy for treating renal hilar tumors: a clinical study of 22 cases. Zhonghua Yi Xue Za Zhi 2018;98:2438-40. [PubMed]
- Prince M, Khanna RV. Robotic-Assisted Laparoscopic Partial Nephrectomy. In: Khanna RV, Bratslavsky G, Stein RJ, editors. Surgical Techniques for Kidney Cancer. New York, NY: Springer New York, 2018;41-8.
- Hu HJ, Wu ZR, Jin YW, et al. Minimally invasive surgery for hilar cholangiocarcinoma: state of art and future perspectives. ANZ J Surg 2019;89:476-80. [Crossref] [PubMed]
- Guerra F, Checcacci P, Vegni A, et al. Surgical and oncological outcomes of our first 59 cases of robotic pancreaticoduodenectomy. J Visc Surg 2018. [Epub ahead of print]. [Crossref] [PubMed]
- Morelli L, Tartaglia D, Bronzoni J, et al. Robotic assisted versus pure laparoscopic surgery of the adrenal glands: a case-control study comparing surgical techniques. Langenbecks Arch Surg 2016;401:999-1006. [Crossref] [PubMed]
- Lin FC, Yang TL, Tung MC, et al. Robot-assisted excision of cervical cystic hygroma through a retroauricular hairline approach: a case report. J Med Case Rep 2016;10:154. [Crossref] [PubMed]
- Vauterin T, Verdonck J, Despeghel AS, et al. Transaxillary robotic thyroidectomy: Belgian team learning from the first 50 cases. B-ENT 2015.Suppl 24:61-8. [PubMed]
- Shen H, Shan C, Qiu M. Systematic review and meta-analysis of transaxillary robotic thyroidectomy versus open thyroidectomy. Surg Laparosc Endosc Percutan Tech 2014;24:199-206. [Crossref] [PubMed]
- Sayin I, Fakhoury R, Prasad VM, et al. Transoral robotic surgery for base of tongue neoplasms. B-ENT 2015.Suppl 24:45-50. [PubMed]
- Hamilton D, Paleri V. Role of transoral robotic surgery in current head & neck practice. Surgeon 2017;15:147-54. [Crossref] [PubMed]
- Dai F, Xu S, Xu W, et al. A Paired Case Controlled Study Comparing the Short-term Outcomes of Da Vinci RATS and VATS Approach for Non-small Cell Lung Cancer. Zhongguo Fei Ai Za Zhi 2018;21:206-11. [PubMed]
- Nasir BS, Bryant AS, Minnich DJ, et al. Performing robotic lobectomy and segmentectomy: cost, profitability, and outcomes. Ann Thorac Surg 2014;98:203-8; discussion 208-9. [Crossref] [PubMed]
- Wilson JL, Louie BE, Cerfolio RJ, et al. The prevalence of nodal upstaging during robotic lung resection in early stage non-small cell lung cancer. Ann Thorac Surg 2014;97:1901-6; discussion 1906-7.
- Seguchi R, Yashiki N, Kato H, et al. Robot assisted tumor resection of an asymptomatic right atrial intracardiac lipoma; report of a case. Kyobu Geka 2011;64:503-5. [PubMed]
- Arsalan M, Smith RL, Squiers JJ, et al. Robotic Excision of a Papillary Fibroelastoma of the Mitral Chordae. Ann Thorac Surg 2016;101:e187-8. [Crossref] [PubMed]
- Woo YJ. Robotic cardiac surgery. Int J Med Robot 2006;2:225-32. [Crossref] [PubMed]
- Treatment Choices for Endometrial Cancer, by Stage [Internet]. [cited 2019 Jan 12]. Available online: https://www.cancer.org/cancer/endometrial-cancer/treating/by-stage.html
- Joseph JR, Smith BW, Liu X, et al. Current applications of robotics in spine surgery: a systematic review of the literature. Neurosurg Focus 2017;42:E2. [Crossref] [PubMed]
- Nathoo N, Cavuşoğlu MC, Vogelbaum MA, et al. In touch with robotics: neurosurgery for the future. Neurosurgery 2005;56:421-33; discussion 421-33. [Crossref] [PubMed]
- Booher G, Vardiman A. Navigated robotic assisted thoracic pedicle screw placement for metastatic renal cell carcinoma. Neurosurg Focus 2018;45:V5. [Crossref] [PubMed]
- Marcus HJ, Cundy TP, Nandi D, et al. Robot-assisted and fluoroscopy-guided pedicle screw placement: a systematic review. Eur Spine J 2014;23:291-7. [Crossref] [PubMed]
- Liu H, Chen W, Wang Z, et al. Comparison of the accuracy between robot-assisted and conventional freehand pedicle screw placement: a systematic review and meta-analysis. Int J Comput Assist Radiol Surg 2016;11:2273-81. [Crossref] [PubMed]
- Gao S, Lv Z, Fang H. Robot-assisted and conventional freehand pedicle screw placement: a systematic review and meta-analysis of randomized controlled trials. Eur Spine J 2018;27:921-30. [Crossref] [PubMed]
- Kim HJ, Jung WI, Chang BS, et al. A prospective, randomized, controlled trial of robot-assisted vs freehand pedicle screw fixation in spine surgery. Int J Med Robot 2017;13. [Crossref] [PubMed]
- Keric N, Doenitz C, Haj A, et al. Evaluation of robot-guided minimally invasive implantation of 2067 pedicle screws. Neurosurg Focus 2017;42:E11. [Crossref] [PubMed]
- Roser F, Tatagiba M, Maier G. Spinal robotics: current applications and future perspectives. Neurosurgery 2013;72 Suppl 1:12-8. [Crossref] [PubMed]
- Hempel E, Fischer H, Gumb L, et al. An MRI-compatible surgical robot for precise radiological interventions. Comput Aided Surg 2003;8:180-91. [Crossref] [PubMed]
- Kostrzewski S, Duff JM, Baur C, et al. Robotic system for cervical spine surgery. Int J Med Robot 2012;8:184-90. [Crossref] [PubMed]
- Jin H, Wang L, Hu Y, et al. Design and control strategy of robotic spinal surgical system. In: The 2011 IEEE/ICME International Conference on Complex Medical Engineering [Internet]. 2011. Available online: http://dx.doi.org/ [Crossref]
- Sahgal A, Chou D, Ames C, et al. Image-Guided Robotic Stereotactic Body Radiotherapy for Benign Spinal Tumors: The University of California San Francisco Preliminary Experience. Technol Cancer Res Treat 2007;6:595-604. [Crossref] [PubMed]
- Dodd RL, Ryu MR, Kamnerdsupaphon P, et al. CyberKnife radiosurgery for benign intradural extramedullary spinal tumors. Neurosurgery 2006;58:674-85; discussion 674-85. [Crossref] [PubMed]
- De Salles AA, Pedroso AG, Medin P, et al. Spinal lesions treated with Novalis shaped beam intensity-modulated radiosurgery and stereotactic radiotherapy. J Neurosurg 2004;101 Suppl 3:435-40. [Crossref] [PubMed]
- Hu X, Lieberman IH. What is the learning curve for robotic-assisted pedicle screw placement in spine surgery? Clin Orthop Relat Res 2014;472:1839-44. [Crossref] [PubMed]
- Hosono N, Yonenobu K, Fuji T, et al. Orthopaedic management of spinal metastases. Clin Orthop Relat Res 1995.148-59. [PubMed]
- Coleman RE. Metastatic bone disease: clinical features, pathophysiology and treatment strategies. Cancer Treat Rev 2001;27:165-76. [Crossref] [PubMed]
- Macedo F, Ladeira K, Pinho F, et al. Bone Metastases: An Overview. Oncol Rev 2017;11:321. [Crossref] [PubMed]
- Morgan JW, Adcock KA, Donohue RE. Distribution of skeletal metastases in prostatic and lung cancer. Mechanisms of skeletal metastases. Urology 1990;36:31-4. [Crossref] [PubMed]
- da Vinci Surgery - Minimally Invasive Robotic Surgery with the da Vinci Surgical System [Internet]. [cited 2019 Jan 12]. Available online: www.davincisurgery.com/da-vinci-surgery/da-vinci-surgical-system/
- Stoianovici D, Cleary K, Patriciu A, et al. Acubot: a robot for radiological interventions. IEEE Trans Rob Autom 2003;19:927-30. [Crossref]
- Melzer A, Gutmann B, Remmele T, et al. INNOMOTION for percutaneous image-guided interventions: principles and evaluation of this MR- and CT-compatible robotic system. IEEE Eng Med Biol Mag 2008;27:66-73. [Crossref] [PubMed]
- Boriani S, Weinstein JN, Biagini R. Primary bone tumors of the spine. Terminology and surgical staging. Spine 1997;22:1036-44. [Crossref] [PubMed]
- Chan P, Boriani S, Fourney DR, et al. An Assessment of the Reliability of the Enneking and Weinstein-Boriani-Biagini Classifications for Staging of Primary Spinal Tumors by the Spine Oncology Study Group. Spine 2009;34:384-91. [Crossref] [PubMed]
- Xiao JR, Huang WD, Yang XH, et al. En Bloc Resection of Primary Malignant Bone Tumor in the Cervical Spine Based on 3-Dimensional Printing Technology. Orthop Surg 2016;8:171-8. [Crossref] [PubMed]
- Singh K, Samartzis D, Vaccaro AR, et al. Current concepts in the management of metastatic spinal disease. The role of minimally-invasive approaches. J Bone Joint Surg Br 2006;88:434-42. [Crossref] [PubMed]
- Choi D, Bilsky M, Fehlings M, et al. Spine Oncology-Metastatic Spine Tumors. Neurosurgery 2017;80:S131-7. [Crossref] [PubMed]
- Solomiichuk V, Fleischhammer J, Molliqaj G, et al. Robotic versus fluoroscopy-guided pedicle screw insertion for metastatic spinal disease: a matched-cohort comparison. Neurosurg Focus 2017;42:E13. [Crossref] [PubMed]
- Gupta P, Gamanagatti S. Preoperative transarterial Embolisation in bone tumors. World J Radiol 2012;4:186-92. [Crossref] [PubMed]
- McBeth PB, Louw DF, Rizun PR, et al. Robotics in neurosurgery. Am J Surg 2004;188:68S-75S. [Crossref] [PubMed]
- Lefranc M, Capel C, Pruvot-Occean AS, et al. Frameless robotic stereotactic biopsies: a consecutive series of 100 cases. J Neurosurg 2015;122:342-52. [Crossref] [PubMed]
- De Benedictis A, Trezza A, Carai A, et al. Robot-assisted procedures in pediatric neurosurgery. Neurosurg Focus 2017;42:E7. [Crossref] [PubMed]
- Jacofsky DJ, Allen M. Robotics in Arthroplasty: A Comprehensive Review. J Arthroplasty 2016;31:2353-63. [Crossref] [PubMed]
- Nagaya T, Nakamura YA, Choyke PL, et al. Fluorescence-Guided Surgery. Front Oncol 2017;7:314. [Crossref] [PubMed]