MyD88 deficiency aggravates the severity of acute pancreatitis by promoting MyD88-independent TRIF pathway-mediated necrosis
Introduction
Acute pancreatitis (AP) is an inflammatory disease with an increasing incidence rate worldwide (1). Severe acute pancreatitis (SAP) has a high mortality rate of up to 40% (2). During AP, a key initiating event is premature activation of zymogens, resulting in pancreatic acinar cells damage. These damaged pancreatic acinar cells release pro-inflammatory mediators and recruit inflammatory cells (3). In the progression of AP, inflammation and parenchymal cell death are 2 pivotal pathologic responses that determine the severity of the AP (3). Necrosis and apoptosis are the main forms of parenchymal cell death, and the severity of AP has been shown to be directly correlated with necrosis and inversely correlated with apoptosis (4-7).
Apoptosis is a highly coordinated process that may be activated by intrinsic and extrinsic pathways. It is executed by the activation of caspases (8). Conversely, necrosis is dependent on the activities of receptor-interacting protein kinase 1 (RIP1) and RIP3, which form a pronecrotic complex with a homotypic interaction motif upon the activation of the tumor necrosis factor (TNF) receptor and related receptors (9). It has been reported that necrosis/necroptosis plays a prominent role in the pathological progress of AP. The mechanisms by which pancreatitis are initiated are not clear; however, it is thought that the disease originates from injury to the acinar cells, which leads to uncontrolled inflammation, which in turn, and more importantly, contributes to parenchymal necrosis. At present, there is no specific medical therapy targeting the inflammatory storm of AP that has been proven to have any clinical benefit (2). The discovery of new therapeutic targets requires a better understanding of the regulatory inflammatory pathways in AP.
Toll-like receptor 4 (TLR4) is one of the best-known toll-like receptors (TLRs) that respond to both pathogen-associated molecular patterns and tissue damage-related signals (10,11). TLR4 activation can also drive inflammation. Recent research on AP has shown that a lack of TLR4 ameliorates pancreatic inflammation in mice (12,13). TLR4 activates 2 distinct intracellular signaling pathways via the adaptor molecules myeloid differentiation primary response protein 88 pathway (the MyD88-dependent pathway) and the Toll-interleukin receptor domain-containing adaptor-inducing interferon-β (TRIF; the MyD88-independent pathway) (14). MyD88-dependent signaling triggers the classical inflammatory cascade, leading to the activation of nuclear factor-κB (NF-κB) and mitogen-activated protein kinases (MAPKs), which in turn lead to the production of pro-inflammatory and anti-inflammatory cytokines. MyD88 can also mediate apoptosis, and mostly does so indirectly through the production of pro-apoptotic intermediates, such as TNF-α (15). Conversely, the MyD88-independent TRIF signaling pathway leads to the activation of interferon regulatory factor 3 (IRF3) and interferon β (IFN-β) production, can mediate delayed NF-κB and MAPK activation (16), and has also been implicated in the RIP1/RIP3-mediated necrosis signaling pathways (9,17).
Based on these findings, we postulated that the Myd88-dependent and Myd88-independent signaling pathways play a role in the pathogenesis of AP. We found that the deletion of MyD88 inhibits the MyD88-dependent pathway-mediated protective immune defense response and enhances the MyD88-independent TRIF pathway, which, surprisingly, increases the severity of experimental AP mainly by promoting pancreatic necrosis via the MyD88-independent TRIF-mediated necrotic signaling pathway. Our findings improve understandings of the complex role of MyD88 in the TLR4-mediated immune defense response and cell death in AP, and may lead to innovative therapies in the treatment of SAP. We present the following article in accordance with the ARRIVE reporting checklist (available at https://atm.amegroups.com/article/view/10.21037/atm-22-5134/rc).
Methods
Animals and reagents
Wild-type C57BL/6 mice and MyD88-deficient mice were obtained from the Model Animal Research Center of Nanjing University (Nanjing, China). All the mice were maintained on a 12-h light/dark cycle with free access to water and food and acclimatized for at least 1 week. Simple random sampling was used to allocate the mice to the control and experimental groups. The mice were fasted for 18 h and only provided water ad libitum before the experiment. The genotype of MyD88-deficient mice was validated before each experiment.
Experiments were performed under a project license (2021737A) granted by Ethics Committee of West China Hospital Sichuan University, in compliance with the institutional guidelines for the care and use of animals. The protocol was prepared before the study, and it was not registered anywhere.
Cerulein and L-arginine hydrochloride were purchased from Sigma Chemical (Sigma, St. Louis, Missouri, USA). Antibodies against RIP1, RIP3, and β-actin were purchased from Cell Signaling Technology (CST, Danvers, Massachusetts, USA). Other items were purchased from standard suppliers or as indicated in the text.
Induction of AP
A cerulein pancreatitis model was induced in the mice (weight: 20–25 g) by 7-hourly intraperitoneal (IP) injections of 50 µg/kg of cerulein (Sigma) in saline, while the control mice were given saline as previously described (18). In addition, 1.65 mg/kg of RIP1 inhibitor necrostatin-1 (Nec-1; Santa Cruz Biotechnology, California, USA) was injected intraperitoneally 10 min before the cerulein injection, every 2 h later for 6 h in total; one-fourth of the above-mentioned concentration was applied to the MyD88−/− mice. The mice were killed 0, 8, and 24 h after the first injection of cerulein. For L-arginine induced pancreatitis, L-arginine hydrochloride (8%; Sigma) was prepared (pH: 7.0). The mice (weight: 20–25 g) received IP injections of L-arginine (4.0 g/kg) every 2 h, and the control mice received IP injections of saline as previously describe (19). The mice were killed at 0, 48, and 72 h after the first injection of L-arginine. The blood samples were centrifuged at 2,000 ×g for 20 min, and the serum was stored at –80 ℃. The pancreas was removed, frozen in liquid nitrogen, and stored at –80 ℃. There were 10 mice in each group, and the experiments were repeated 3 times to confirm the results. Pathological evaluation was done by two pathologists.
Biochemical assays
The levels of serum amylase and lipase were measured by means of a commercially available kit (R&D System, MN, USA). The extent of neutrophil infiltration in the pancreas was quantitated by measuring tissue myeloperoxidase (MPO) activity (20). The enzyme activity was measured by a MPO kit (Nanjing Jiancheng Bioengineering Institute, Nanjing, China), and calculated as a percentage of the control.
Morphology assays
For the light microscopy, the pancreas was fixed in 4% paraformaldehyde, embedded in paraffin, and 5-mm sections were processed for hematoxylin and eosin (H&E) staining. The extent of necrosis was scored by 2 experienced morphologists.
Terminal deoxynucleotidyl transferase-mediated dUTP-biotin nick-end labelling (TUNEL) assays
TUNEL assays were used to quantify the apoptosis of the pancreatic tissue sections. The sections were stained using TUNEL (Merck & Co., Inc., USA). Dark brown staining of the nucleus was observed in TUNEL-positive cells [containing labelled deoxyribonucleic acid (DNA) fragments]. The sections were counterstained with 0.3% methyl green. The numbers of positive apoptotic cells were counted in 10 high-power fields (×400 magnification) (21).
Quantitative real-time reverse transcription-polymerase chain reaction (RT-PCR)
The ribonucleic acids (RNAs) from the pancreas were prepared according to method described in Griffin’s study (22). Each tissue was lysed with TRIzol (Invitrogen, California, USA) for total RNA isolation. Next, a DNA reverse-transcription system (Invitrogen) was used for the reverse transcription. Polymerase chain reaction (PCR) was performed in the presence of specific primers. The following primers were used: TLR4, forward: 5'-CAGAACTTCAGTGGCTGGA-3', reverse: 5'-Tagggtttcctgtcagtatc-3'; interleukin (IL)-1 receptor-associated kinase 4 (IRAK4), forward: 5'-CGGCGACGACAGATACAAT’-3', reverse: 5'-CTTTTGACGGTTTGGGGAA-3'; TRIF, forward: 5'-CAGGGACCGGGAGATCTA-3', reverse: 5'-CATGTTCCGAACCGACAGC-3'; TNF-α, forward: 5'-AGACCCTCACACTCAGATCAT-3', reverse: 5'-AGTCACAGAAGGAGTGGCTAA-3'; IL-6, forward: 5'-CACTAGGTTTGCCGAGTAGA-3', reverse: 5'-CGTGAAAAGATGACCCAGAT-3'; IL-10, forward: 5'-CAACATACTGCTAACCGACTC-3', reverse: 5'-cctggggcatcacttctac-3'; β-actin, forward: 5'-CGTGAAAAGATGACCCAGAT-3', reverse: 5'-ACCCTCATAGATGGGCACA-3'. The 40-cycle program (94 ℃ for 20 s, 53 ℃ for 30 s, and 72 ℃ for 30 s) conditions were used for all PCRs on an iCycler IQ (Bio-Rad) system. β-actin was used as the internal control in each reaction, and relative quantitative gene expression was calculated using the 2−ΔΔCt method (23). Each sample was analyzed in triplicate.
Western blot analysis
The total protein of the pancreas tissues was extracted. First, portions of the frozen pancreas tissue were rapidly homogenized in liquid nitrogen, and then reconstituted in ice-cold radioimmunoprecipitation assay buffer containing 1 mmol/L of phenylmethanesulfonylfluoride and a cocktail of protease inhibitors (1:100 dilution) (Sigma, St. Louis, Missouri, USA). The tissue lysates were rotated for 40 min at 4 ℃ and centrifuged at 4 ℃ for 15 min at 16,000 ×g. The supernatants were recovered, and total amounts of protein were measured using the bicinchoninic acid assay method (Pierce, Rockford, Illinois, USA). Twenty micrograms of protein was subjected to 12% sodium dodecyl sulfate-polyacrylamide gel electrophoresis. Then, transferred onto polyvinylidene difluoride membranes (Millipore, Massachusetts, USA). The membranes blocked with 5% milk powder solution for 1 h at room temperature and incubated at 4 ℃ overnight with rabbit monoclonal anti-RIP1 antibody (1:1,000 dilution), anti-RIP3 antibody (1:1,000 dilution) and anti-β-actin antibody (1:1,000 dilution). Membranes were washed for 10 min 3 times. Then, it incubated with goat polyclonal anti-rabbit immunoglobulin G secondary antibody (Cell Signaling) conjugated to horseradish peroxidase (1:5,000 dilution) for 1 h at room temperature. Finally, the antibody binding was visualized using the enhanced chemiluminescence system (Pierce, Rockford, USA).
Statistical analysis
The highest and lowest values of each group’s results were removed, and the rest of the values were averaged. The results are expressed as the mean ± standard error of the mean (SEM). The data were analyzed using a one-way analysis of variance with the Tukey-Kramer post-hoc test. A P value <0.05 was considered statistically significant.
Results
Effect of MyD88 deletion on cerulein-induced AP
We assessed the severity of AP in the cerulein-administered wild-type and MyD88−/− mice. The morphological changes observed in pancreatitis included acinar cell vacuolization, edema, and necrosis, all of which were significantly more severe in the MyD88−/− mice than the wild-type mice (Figure 1). The levels of serum amylase (Figure 2A) and lipase (Figure 2B) of the MyD88−/− mice were significantly higher than those of the wild-type mice. Compared to the wild-type mice, the MyD88−/− mice had increased pancreatic tissue MPO activity (Figure 2C).
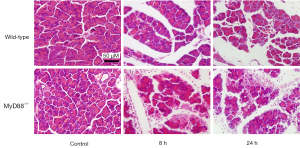
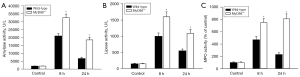
Effect of MyD88 deletion on the TLR4-mediated MyD88-dependent and MyD88-independent pathways in cerulein-induced AP
We assessed TLR4 expression in the cerulein-induced wild-type and MyD88−/−mice. The results revealed an increase in TLR4 messenger RNA (mRNA) expression in the cerulein-treated wild-type mice compared to the control animals, and this increase was significantly more enhanced in the MyD88−/− mice than the wild-type mice (Figure 3A). We then assessed the expression of interleukin-1 receptor-associated kinase 4 (IRAK4), which is the downstream molecule of MyD88, and found that IRAK4 expression in the MyD88−/− mice did not change after cerulein treatment, but its expression in the wild-type mice increased 24 h after cerulein treatment compared to that of the control mice (Figure 3B). We also measured the expression of TRIF, which represents the MyD88-independent pathway, and noted a slight increase in TRIF mRNA expression in the cerulein-treated wild-type mice. The MyD88−/− mice were significantly more enhanced than the wild-type mice (Figure 3C). These findings indicate that the activation of IRAK4 was dependent on MyD88, and the MyD88-dependent pathway was blocked in the cerulein-induced MyD88−/− mice. Conversely, the TLR4-mediated MyD88-independent TRIF pathway was significantly enhanced in the cerulein-induced MyD88−/− mice.
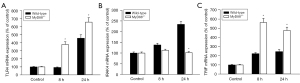
Effect of MyD88 deletion on the inflammatory response in cerulein-induced AP
To assess the pancreatic inflammatory response, TNF-α, IL-6, and IL-10 levels were examined by real-time RT-PCR. Both pro-inflammatory (TNF-α and IL-6) and anti-inflammatory (IL-10) cytokines were increased in the wild-type mice after cerulein treatment. TNF-α and IL-10 mRNA levels were more decreased in the MyD88−/− mice than the wild-type mice (Figure 4A,4B). However, at the late stage (24 h), the IL-6 mRNA level of the MyD88−/− mice was significantly more increased than that of the wild-type mice (Figure 4C).
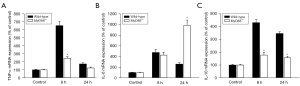
Effect of MyD88 deletion on cell death in cerulein-induced AP
We also investigated apoptosis during cerulein-induced AP (Figure 5A). The in-situ TUNEL assays showed that apoptosis was more increased in the wild-type mice treated with cerulein at the early stage (8 h) than the control mice. Additionally, few apoptosis positive cells were detected at the late stage (24 h) of the disease. However, the increase of apoptosis in the MyD88−/− mice was less than that in the wild-type mice at the early stage (Figure 5B). A quantitative analysis of acinar cell necrosis was undertaken by standard histological examination. The results revealed that there was significantly more necrosis in the MyD88−/− mice than the wild-type mice (Figure 5C). We then assessed the necrotic mediators of RIP1 and RIP3 using Western blot assays (Figure 6A). RIP1 revealed a marked degradation at the early stage (8 h), and RIP3 was slightly more increased in the cerulein-induced wild-type mice than the control mice (Figure 6B,6C). Conversely, the expression of RIP1 and RIP3 was significantly more enhanced in the MyD88−/− mice than the wild-type mice (Figure 6B,6C).
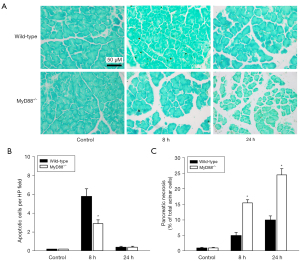
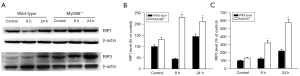
Effect of Nec-1 on cerulein-induced necrosis in MyD88−/− mice
To examine the role of the RIP1 inhibitor Nec-1 on necrosis in AP in the MyD88−/− mice, we investigated the necrosis in the cerulein-induced MyD88−/− mice with or without Nec-1. The extent of necrosis was obtained by histological examination. Lactate dehydrogenase (LDH) was also tested to examine the severity of necrosis. The results showed that cerulein-induced acinar cell necrosis was significantly reduced by the additional Nec-1 treatment in the MyD88−/− mice (Figure 7).
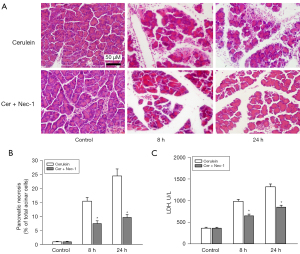
Effect of MyD88 deletion on L-arginine induced AP
To confirm that the enhanced effects of the MyD88−/− mice were not cerulein-induced AP model-specific, a L-arginine induced AP model was examined. The morphological changes were more severe in the MyD88−/− mice than the wild-type mice (Figure 8). Further, there were significantly higher levels of serum amylase (Figure 9A) and lipase (Figure 9B), more pancreatic acinar cell necrosis (Figure 9C), and higher levels of LDH (Figure 9D) in the MyD88−/− mice than the wild-type mice.
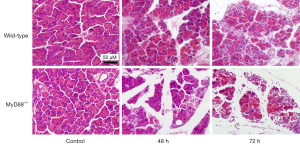
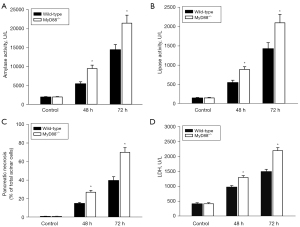
Discussion
Inflammation and parenchymal cell death are characteristics of AP development (3). Once the premature intra-acinar digestive zymogens are activated, the injured acinar cells produce and release inflammatory mediators, and inflammatory cells are then recruited into the pancreas, which leads to systemic inflammation (24,25). TLRs are thought to be important receptors of the innate immune response. A previous study showed that TLR4, a typical TLR, plays a pro-inflammatory role in AP (12). MyD88 is the first and most essential utilized adaptor of TLR4 (26). The expression of Myd88 increases in AP pancreatic tissue (27). Previous studies have shown that Myd88 could affect the severity of AP. And Myd88 could mediate AP inflammation and SAP-related intestinal injury regulation (28,29). In addition, autophagy and oxidative stress play important roles in the pathogenesis of AP. MyD88 deficiency ameliorates oxidative injury in an autophagy-dependent mechanism (30). However, its role in AP remains unclear.
In the present study, MyD88-deficient mice were used to establish a cerulein-induced AP model. The results showed that the severity of AP was increased in the MyD88−/− mice, which had increased levels of serum amylase and lipase, pancreatic MPO activity, and increased pancreatic acinar cell necrosis compared to the wild-type mice. Our findings that the MyD88−/− mice were likely to develop more severe pancreatitis may be surprising, as previous studies have reported that (12,13). As a downstream molecule of the TLR4 pathway, MyD88 should act concurrently with its upper stream regulator. To explain this unexpected finding, we measured the expression of TLR4, and found that the expression of TLR4 was significantly more enhanced in the MyD88−/− mice than the wild-type mice. Thus, we hypothesized that MyD88 was not the only pathway that acts with TLR4, and the other pathway may exert the opposite function in the progression of pancreatitis.
We then focused on both the MyD88-dependent and MyD88-independent TRIF signaling pathways. The MyD88-dependent signaling pathway was inhibited, which resulted in the inhibition of IL-10 in the MyD88−/− mice. IL-10 is the most potent anti-inflammatory cytokine, and research has shown that IL-10 protects the pancreas by stopping monocytes and macrophages from releasing pro-inflammatory factors and regulating systemic immune reactions and inflammatory reactions (31). Thus, the induction of IL-10 mediated by the MyD88-dependent signaling pathway is a protective immune defense mechanism that occurs in response to pancreatic tissue damage. Conversely, TRIF expression was significantly more increased in the MyD88−/− mice than the wild-type mice, indicating that the TRIF signaling pathway was primarily activated in the absence of MyD88. TRIF can mediate NF-κB and MAPK activation (16), resulting in the release of inflammatory cytokines at the late stage of this disease. In our study, the level of the pro-inflammatory cytokine, IL-6, was significantly increased at the late stage in the MyD88−/− mice. In addition, increased IL-6 levels have been shown to be proportional to the increased severity of AP (32).
TRIF plays an important role in many diseases. It could mediate production protective natural tumor-reactive IgM by B1 cells (33). In addition, preventive effect of lycopene in ulcerative colitis mice also through the TLR4-TRIF Pathway (34). Microglia necroptosis was regulated by TLR4-TRIF Pathway (35). In AP, loss of TRIF accelerates AP. And the TRIF depletion favors acinar cell necrosis to apoptosis (36). Thus, TRIF could affect the severity of AP. The TRIF signaling pathway also has been known to be involved in cell death signaling pathways, as TRIF has a RIP homotypic interaction motif that can physically interact with death receptors RIP1 and RIP3 (9,17). Apoptosis is considered predominantly protective (4), while necrosis can lead to organ damage and death (37). Apoptosis is mediated by caspase activation. Caspase also protects the pancreas from necrosis (4,38). The MyD88-dependent pathway has been reported to induce apoptosis through the TNF-α mediated caspase-8 pathway (39,40). Thus, the deletion of MyD88 led to less NF-κB activation and TNF-α production, all of which resulted in the reduced induction of caspase 8-mediated apoptosis.
Consistent with previous research, we found that apoptosis was lower in the MyD88−/− mice than the wild-type mice during cerulein-induced AP. Similarly, the level of TNF-α was more reduced in the MyD88−/− mice than the wild-type mice. The uncontrollable process was previously thought to be a characteristic of necrosis. However, recent research has shown that necrosis may occur as a controlled behavior (41). This so-called necroptosis is mediated by the 2 death receptors, RIP1 and RIP3 (9). Several recent studies have reported that TLRs activate programmed necrosis through the TRIF-RIP3 pathway or RIP1-RIP3 necrotic pathway (9,42).
In this study, both RIP1 and RIP3 expressions were more enhanced in the MyD88−/− mice than the wild-type mice. As a result, necrosis was significantly increased in the pancreas of the MyD88−/− mice. In addition, the special inhibitor of RIP1 Nec-1 reduced cerulein-induced necrosis in the MyD88−/− mice, but some necrosis was still observed. This finding indicates that the special inhibition of RIP1 decreased necrosis during cerulein-induced AP in MyD88−/− mice. The residue of necrosis might be due to the RIP1-independent RIP3-mediated necrotic pathway. A previous study also observed that RIP3 knockout mice showed less tissue damage in cerulein-induced AP than wild-type mice (43). Overall, these findings suggest that the deletion of MyD88 promotes necrosis and aggravates AP by enhancing the TRIF-mediated RIP1/RIP3 necrotic pathway.
A L-arginine induced AP model was adopted to confirm that the findings of the present study were not unique to the cerulean-induced AP model. The L-arginine-induced AP model was relatively more severe than the hyperstimulation cerulein model. It was also associated with a longer period (peak injury occurred in 72 h) than the cerulein model (44). In the present study, the necrosis of L-arginine-induced AP mice was significantly greater than that of the cerulein-induced AP mice. Deleting MyD88 also significantly enhanced necrosis in the pancreas and aggravated the severity of L-arginine induced AP. These results proved that MyD88 is a significant determinant of the severity of AP, and its effect is not model-specific but common to all models tested.
Conclusions
The present study showed that MyD88 acts as a key mediator of the TLR4-mediated immune defense response and cell death in AP through the MyD88-dependent/MyD88-independent pathway. The deletion of MyD88 in AP decreased the induction of IL-10 and TNF-α mediated apoptosis, and enhanced MyD88-independent TRIF pathway-mediated necrosis via the activation of RIP1/RIP3, all of which led to increased necrosis and inflammation in the pancreas, which in turn aggravated the severity of AP. Thus, a lack of MyD88 inhibited the TLR4-mediated protective immune defense response and enhanced the MyD88-independent TRIF pathway, surprisingly accelerating the severity of AP largely by promoting pancreatic necrosis via the TRIF-mediated necrotic signaling pathway. The critical role of MyD88 in the immune defense response and cell death indicates that MyD88 represents a potential therapeutic target in the management of AP.
Acknowledgments
Funding: This study was supported by the 1.3.5 Project for Disciplines of Excellence, West China Hospital, Sichuan University (Nos. ZYGD20006 and ZYJC18027).
Footnote
Reporting Checklist: The authors have completed the ARRIVE reporting checklist. Available at https://atm.amegroups.com/article/view/10.21037/atm-22-5134/rc
Data Sharing Statement: Available at https://atm.amegroups.com/article/view/10.21037/atm-22-5134/dss
Conflicts of Interest: All authors have completed the ICMJE uniform disclosure form (available at https://atm.amegroups.com/article/view/10.21037/atm-22-5134/coif). The authors have no conflicts of interest to declare.
Ethical Statement: The authors are accountable for all aspects of the work in ensuring that questions related to the accuracy or integrity of any part of the work are appropriately investigated and resolved. Experiments were performed under a project license (No. 2021737A) granted by Ethics Committee of West China Hospital Sichuan University, in compliance with the rules of institutional guidelines for the care and use of animals.
Open Access Statement: This is an Open Access article distributed in accordance with the Creative Commons Attribution-NonCommercial-NoDerivs 4.0 International License (CC BY-NC-ND 4.0), which permits the non-commercial replication and distribution of the article with the strict proviso that no changes or edits are made and the original work is properly cited (including links to both the formal publication through the relevant DOI and the license). See: https://creativecommons.org/licenses/by-nc-nd/4.0/.
References
- Iannuzzi JP, King JA, Leong JH, et al. Global Incidence of Acute Pancreatitis Is Increasing Over Time: A Systematic Review and Meta-Analysis. Gastroenterology 2022;162:122-34. [Crossref] [PubMed]
- Boxhoorn L, Voermans RP, Bouwense SA, et al. Acute pancreatitis. Lancet 2020;396:726-34. [Crossref] [PubMed]
- Lee PJ, Papachristou GI. New insights into acute pancreatitis. Nat Rev Gastroenterol Hepatol 2019;16:479-96. [Crossref] [PubMed]
- Mareninova OA, Sung KF, Hong P, et al. Cell death in pancreatitis: caspases protect from necrotizing pancreatitis. J Biol Chem 2006;281:3370-81. [Crossref] [PubMed]
- Bhatia M. Apoptosis versus necrosis in acute pancreatitis. Am J Physiol Gastrointest Liver Physiol 2004;286:G189-96. [Crossref] [PubMed]
- Bhatia M. Apoptosis of pancreatic acinar cells in acute pancreatitis: is it good or bad? J Cell Mol Med 2004;8:402-9. [Crossref] [PubMed]
- Louhimo J, Steer ML, Perides G. Necroptosis Is an Important Severity Determinant and Potential Therapeutic Target in Experimental Severe Pancreatitis. Cell Mol Gastroenterol Hepatol 2016;2:519-35. [Crossref] [PubMed]
- Gaman L, Dragos D, Vlad A, et al. Phytoceuticals in Acute Pancreatitis: Targeting the Balance between Apoptosis and Necrosis. Evid Based Complement Alternat Med 2018;2018:5264592. [Crossref] [PubMed]
- He S, Liang Y, Shao F, et al. Toll-like receptors activate programmed necrosis in macrophages through a receptor-interacting kinase-3-mediated pathway. Proc Natl Acad Sci U S A 2011;108:20054-9. [Crossref] [PubMed]
- Takeda K, Akira S. Toll receptors and pathogen resistance. Cell Microbiol 2003;5:143-53. [Crossref] [PubMed]
- Takeda K, Akira S. Toll-like receptors in innate immunity. Int Immunol 2005;17:1-14. [Crossref] [PubMed]
- Sharif R, Dawra R, Wasiluk K, et al. Impact of toll-like receptor 4 on the severity of acute pancreatitis and pancreatitis-associated lung injury in mice. Gut 2009;58:813-9. [Crossref] [PubMed]
- Wu J, Ma X, Chen W, et al. Protective effects of HTD4010, a Reg3α/PAP-derived peptide, in mouse model of acute pancreatitis via toll-like receptor 4 pathway. Biochem Biophys Res Commun 2019;512:670-7. [Crossref] [PubMed]
- Lin C, Wang H, Zhang M, et al. TLR4 biased small molecule modulators. Pharmacol Ther 2021;228:107918. [Crossref] [PubMed]
- Wang J, Kobayashi M, Han M, et al. MyD88 is involved in the signalling pathway for Taxol-induced apoptosis and TNF-alpha expression in human myelomonocytic cells. Br J Haematol 2002;118:638-45. [Crossref] [PubMed]
- Brown J, Wang H, Hajishengallis GN, et al. TLR-signaling networks: an integration of adaptor molecules, kinases, and cross-talk. J Dent Res 2011;90:417-27. [Crossref] [PubMed]
- Schilling JD, Machkovech HM, He L, et al. TLR4 activation under lipotoxic conditions leads to synergistic macrophage cell death through a TRIF-dependent pathway. J Immunol 2013;190:1285-96. [Crossref] [PubMed]
- Gironella M, Folch-Puy E, LeGoffic A, et al. Experimental acute pancreatitis in PAP/HIP knock-out mice. Gut 2007;56:1091-7. [Crossref] [PubMed]
- Yang X, Yao L, Fu X, et al. Experimental Acute Pancreatitis Models: History, Current Status, and Role in Translational Research. Front Physiol 2020;11:614591. [Crossref] [PubMed]
- Linders J, Madhi R, Mörgelin M, et al. Complement Component 3 Is Required for Tissue Damage, Neutrophil Infiltration, and Ensuring NET Formation in Acute Pancreatitis. Eur Surg Res 2020;61:163-76. [Crossref] [PubMed]
- Chao KC, Chao KF, Chuang CC, et al. Blockade of interleukin 6 accelerates acinar cell apoptosis and attenuates experimental acute pancreatitis in vivo. Br J Surg 2006;93:332-8. [Crossref] [PubMed]
- Griffin M, Abu-El-Haija M, Abu-El-Haija M, et al. Simplified and versatile method for isolation of high-quality RNA from pancreas. Biotechniques 2012;52:332-4. [Crossref] [PubMed]
- Schmittgen TD, Livak KJ. Analyzing real-time PCR data by the comparative C(T) method. Nat Protoc 2008;3:1101-8. [Crossref] [PubMed]
- Grady T, Liang P, Ernst SA, et al. Chemokine gene expression in rat pancreatic acinar cells is an early event associated with acute pancreatitis. Gastroenterology 1997;113:1966-75. [Crossref] [PubMed]
- Bhatia M, Brady M, Shokuhi S, et al. Inflammatory mediators in acute pancreatitis. J Pathol 2000;190:117-25. [Crossref] [PubMed]
- Warner N, Núñez G. MyD88: a critical adaptor protein in innate immunity signal transduction. J Immunol 2013;190:3-4. [Crossref] [PubMed]
- Fan JJ, Mei QX, Deng GY, et al. Porous SiO2 -coated ultrasmall selenium particles nanospheres attenuate cerulein-induce acute pancreatitis in mice by downregulating oxidative stress. J Dig Dis 2021;22:363-72. [Crossref] [PubMed]
- Li H, Xie J, Guo X, et al. Bifidobacterium spp. and their metabolite lactate protect against acute pancreatitis via inhibition of pancreatic and systemic inflammatory responses. Gut Microbes 2022;14:2127456. [Crossref] [PubMed]
- Yang X, Wan J, Li N, et al. MiR155 Disrupts the Intestinal Barrier by Inducing Intestinal Inflammation and Altering the Intestinal Microecology in Severe Acute Pancreatitis. Dig Dis Sci 2022;67:2209-19. [Crossref] [PubMed]
- Qi M, Liao S, Wang J, et al. MyD88 deficiency ameliorates weight loss caused by intestinal oxidative injury in an autophagy-dependent mechanism. J Cachexia Sarcopenia Muscle 2022;13:677-95. [Crossref] [PubMed]
- Zhang XP, Lin Q, Zhou YF. Progress of study on the relationship between mediators of inflammation and apoptosis in acute pancreatitis. Dig Dis Sci 2007;52:1199-205. [Crossref] [PubMed]
- Ćeranić DB, Zorman M, Skok P. Interleukins and inflammatory markers are useful in predicting the severity of acute pancreatitis. Bosn J Basic Med Sci 2020;20:99-105. [PubMed]
- Dyevoich AM, Disher NS, Haro MA, et al. A TLR4-TRIF-dependent signaling pathway is required for protective natural tumor-reactive IgM production by B1 cells. Cancer Immunol Immunother 2020;69:2113-24. [Crossref] [PubMed]
- Li Y, Pan X, Yin M, et al. Preventive Effect of Lycopene in Dextran Sulfate Sodium-Induced Ulcerative Colitis Mice through the Regulation of TLR4/TRIF/NF-κB Signaling Pathway and Tight Junctions. J Agric Food Chem 2021;69:13500-9. [Crossref] [PubMed]
- Tu Y, Yang Y, Wang Y, et al. Developmental exposure to chlorpyrifos causes neuroinflammation via necroptosis in mouse hippocampus and human microglial cell line. Environ Pollut 2022;314:120217. [Crossref] [PubMed]
- Regel I, Raulefs S, Benitz S, et al. Loss of TLR3 and its downstream signaling accelerates acinar cell damage in the acute phase of pancreatitis. Pancreatology 2019;19:149-57. [Crossref] [PubMed]
- Garg PK, Madan K, Pande GK, et al. Association of extent and infection of pancreatic necrosis with organ failure and death in acute necrotizing pancreatitis. Clin Gastroenterol Hepatol 2005;3:159-66. [Crossref] [PubMed]
- Gukovskaya AS, Pandol SJ. Cell death pathways in pancreatitis and pancreatic cancer. Pancreatology 2004;4:567-86. [Crossref] [PubMed]
- Salaun B, Romero P, Lebecque S. Toll-like receptors' two-edged sword: when immunity meets apoptosis. Eur J Immunol 2007;37:3311-8. [Crossref] [PubMed]
- Weng D, Marty-Roix R, Ganesan S, et al. Caspase-8 and RIP kinases regulate bacteria-induced innate immune responses and cell death. Proc Natl Acad Sci U S A 2014;111:7391-6. [Crossref] [PubMed]
- Edinger AL, Thompson CB. Death by design: apoptosis, necrosis and autophagy. Curr Opin Cell Biol 2004;16:663-9. [Crossref] [PubMed]
- Kaiser WJ, Sridharan H, Huang C, et al. Toll-like receptor 3-mediated necrosis via TRIF, RIP3, and MLKL. J Biol Chem 2013;288:31268-79. [Crossref] [PubMed]
- He S, Wang L, Miao L, et al. Receptor interacting protein kinase-3 determines cellular necrotic response to TNF-alpha. Cell 2009;137:1100-11. [Crossref] [PubMed]
- Ren Y, Liu W, Zhang L, et al. Milk fat globule EGF factor 8 restores mitochondrial function via integrin-medicated activation of the FAK-STAT3 signaling pathway in acute pancreatitis. Clin Transl Med 2021;11:e295. [Crossref] [PubMed]
(English Language Editor: L. Huleatt)