FGF10 mediates protective anti-oxidative effects in particulate matter-induced lung injury through Nrf2 and NF-κB signaling
Introduction
Industrialization and urbanization directly impact atmospheric pollution, which is recognized as a global environmental threat to human health. Regarded as the fundamental constituent of atmospheric pollutants, particulate matter (PM) poses a considerable health risk (1). As a chemical mixture of solid particles and liquid droplets, PM mainly consists of exhaust particles from vehicles and those released as a result of the incomplete combustion of coal. Due to their aerodynamics characteristics in the context of a functional lung, PM deposits in the bronchial and alveolar epithelium (2). The deposition of PM in the pulmonary epithelium inevitably leads to premature epithelial senescence and secretion of pro-inflammatory cytokines, which closely relates to the oxidative stress and lays the foundation for the pathological characteristics associated with PM-induced lung injury (3).
As documented in both epidemiological investigations and fundamental research, short- or long-term PM exposure is closely related to the morbidity and mortality of individuals suffering from various respiratory diseases, such as bronchitis and alveolitis (4). However, the molecular mechanism underlying the toxic effects of PM has not yet been fully elucidated. Besides symptomatic management such as inhalation of budesonide, there are only partially effective clinical interventions for protecting the lung from the harmful effects of PM. Considering the continuous exposure to PM in our daily lives and the heavy socio-economic burden of respiratory diseases, further investigation of the molecular mechanism mediating the deleterious effects of PM is urgently required to identify novel preventive and therapeutic strategies.
Oxidative stress, which is characteristic of an imbalance between the intrinsic oxidation and anti-oxidation systems, is a typical cellular response to PM and is reported as a pivotal pathophysiological event in PM-induced lung injury (5). As the first mechanical and immunological surface proximal to PM exposure, the pulmonary epithelium is the primary source of the overproduction of reactive oxygen species (ROS). ROS excess not only drives lipid peroxidation and protein denaturation, which mediates the cytotoxic effects of PM leading to premature epithelial senescence, but also functions as an essential regulator of redox-sensitive signaling, contributing to secretion of pro-inflammatory cytokines (6).
Nuclear factor-κB (NF-κB) signaling and nuclear factor erythroid 2 related factor 2 (Nrf2) signaling are the two well-known redox-sensitive signaling pathways (7). NF-κB initiates and also sustains the inflammatory response. Wang et al. reported that oxidative stress-induced NF-κB signaling was essential for the elevated secretion of the pro-inflammatory cytokines, interleukin (IL)-6 and IL-8, from bronchial epithelial cells exposed to PM (8). In contrast to the pro-inflammatory role of NF-κB signaling, Nrf2 signaling plays an intrinsic cytoprotective role against oxidative stress; it regulates the expression of numerous antioxidant factors, including hemeoxygenase-1 (HO-1) and quinine oxidoreductase 1 (NQO1), through the antioxidant response element (ARE) to eliminate the excess ROS (9).
It has been reported that herbal extracts from plants such as Djulis and curcumin exert a regulatory effect on Nrf2 signaling to protect pulmonary epithelial cells from oxidative injury induced by PM exposure (10,11). Moreover, the activation of Nrf2 signaling is essential for the inhibitory effect of sythiaside on NF-κB signaling, which exerts an anti-inflammatory effect against cigarette smoke (12). Considering the protective anti-oxidative role of Nrf2 signaling during oxidative stress, a potential endogenous modulator of Nrf2 signaling might preserve the redox state, thereby providing a promising therapeutic strategy for the treatment of PM-induced lung injury.
Fibroblast growth factor 10 (FGF10), which acts as a canonic member of the FGF superfamily, is a typical paracrine FGF secreted by mesenchymal cells (13). FGF10 specifically interacts with FGF receptor 2b (FGFR2b), and FGFR2b is exclusively expressed by epithelial cells, enabling FGF10-mediated mesenchymal-to-epithelial transition during development, differentiation, and regeneration (14). The pleiotropic role of FGF10 and the associated molecular mechanism involved in the epithelial repair process of PM-induced lung injury is a significant focus of current research. Our previous studies have identified the protective role of FGF10 in PM-induced lung injury. In particular, we reported that blockade of high-mobility group box 1 (HMGB1)-toll-like receptor 4 (TLR4) inflammatory signaling and dysregulation of endoplasmic reticulum stress constituted the underlying molecular mechanisms (15,16). Given the vital role of oxidative stress in mediating the toxic effects of PM, it is critical to evaluate whether FGF10 impacts oxidative stress during PM-induced lung injury. It is known for the first time to explore whether FGF10 exerts an anti-oxidative effect in PM-induced lung injury and potential molecular mechanism, which might be a therapeutic candidate for environmentally-induced lung injury.
Recently, the anti-oxidative effect of FGF10 in different organs, except for the lung, has been reported. For example, Dong et al. demonstrated that FGF10 activated the Phosphatidylinositide 3-kinases/protein kinase B (PI3K/Akt) signaling-induced antioxidant response to promote peripheral nerve regeneration (17). Moreover, FGF10 was reported to activate the Akt-dependent Nrf2 signaling axis to enable a protective effect against hepatic ischemia-reperfusion injury (18). Based on these findings, FGF10 may exert an anti-oxidative effect against PM exposure. The current study tested the hypothesis that FGF10 is protective against PM-induced lung injury via Nrf2-dependent suppression of NF-κB signaling. To test this hypothesis, C57BL/6 mice were subjected to PM exposure with or without FGF10 administration. Human bronchial epithelial cells were utilized to investigate the mechanism associated with FGF10-mediated regulation of the redox-sensitive NF-κB and Nrf2 signaling. We present the following article in accordance with the ARRIVE reporting checklist (available at https://atm.amegroups.com/article/view/10.21037/atm-22-4389/rc).
Methods
Reagents and antibodies
The standard reference airborne (1649b) was purchased from NIST (Gaithersburg, MD, USA). For in vivo animal experiments, exogenous recombinant FGF10 was obtained from the School of Pharmaceutical Science affiliated with Wenzhou Medical University. For in vitro cell experiments, exogenous recombinant FGF10 was purchased from PeproTech (Shanghai, China). Antibodies against FGF10, phospho-p65, p65, phospho-IκBα, IκBα, Nrf2, Kelch-like ECH-associated protein 1 (KEAP1), heme oxygenase 1 (HO-1), and Quinone Oxidoreductase 1 (NQO1) were purchased from Cell Signaling Technology (Beverly, MA, USA). The enzyme linked immunosorbent assay (ELISA) kits for mouse FGF10, mouse IL-6, mouse IL-8, human IL-6, and human IL-8 were purchased from Boyun Biotechnology (Shanghai, China). The compound BAY11-7082 was purchased from Selleck (Houston, Texas, USA). N-acetylcysteine (NAC) and ML385 were purchased from Sigma-Aldrich (Shanghai, China).
Cells and animals
Human bronchial epithelial cells (HBECs) were purchased from the Chinese Academy of Sciences (Shanghai, China) and cultured at 37 ℃ inside the cell culture incubator with 5% carbon dioxide (CO2) in Roswell Park Memorial Institute (RPMI)-1640 medium (GIBCO, Weihao, MA, USA) supplemented with 10% fetal bovine serum (FBS, GIBCO), 50 U/mL penicillin, and streptomycin (GIBCO). Male C57BL/6J mice (6–8 weeks, 20–25 g) were purchased from Beijing Vital River Laboratory Animal Technology Company (Beijing, China) and housed in a specific pathogen-free facility at The First Affiliated Hospital of Wenzhou Medical University. Animal experiments were performed under a project license (No. 2021-0037) granted by the ethics committee for animal welfare at The First Affiliated Hospital of Wenzhou Medical University, in compliance with national guidelines for the care and use of animals. A protocol was prepared before the study without registration.
Experimental design
For in vivo animal experiments, the mice were randomly grouped (Vehicle group, FGF10 group, PM group, PM + FGF10 group; n=12 mice per group). The mouse model of PM-induced lung injury was established through intratracheal instillation of 4 mg/kg PM for 2 consecutive days. To explore the potential effect of FGF10, 5 mg/kg FGF10 was intratracheally instilled 1 h before PM exposure. The mice were euthanized with an intraperitoneal injection of pentobarbital sodium 24 h after the second PM exposure, and their bronchoalveolar lavage (BAL) and lung tissues were collected.
For in vitro cell culture experiments, PM was initially re-suspended in phosphate buffer saline (PBS) at a stock concentration of 4 mg/mL, and then HBECs were incubated with 200 µg/mL PM for the indicated time. FGF10 (10 ng/mL), NAC (2.5 mM), BAY 11-7082 (5 µM), or ML385 (10 µM) were added to the pretreated HBECs at 1 h before PM exposure for the indicated purpose.
Hematoxylin and eosin (H&E) and histological analysis
Firstly, the harvested lung tissues were fixed in 4% paraformaldehyde for 24 h. Next, the lung tissues were embedded in paraffin and precisely sectioned into 5 µm sections. Finally, the slides were de-paraffinized and separately stained with H&E for histological analysis. Three independent experienced investigators carried out the histological analysis. The histological analysis criteria were as follows: 0 meant that no apparent inflammation was observed; 1 denoted occasional cuffing with inflammatory cells; 2 represented that the majority of bronchi or vessels were surrounded by a thin layer (1 to 5) of inflammatory cells; and 3 indicated that the majority of bronchi or vessels were surrounded by a thick layer (>5) of inflammatory cells (19).
Redox state measurement
For in vitro experiments, the HBECs were plated in 24-well plates and exposed to PM after pre-treatment with FGF10. Subsequently, the HBECs were incubated with 2,7-Dichlorodihydrofluorescein diacetate (DCFH-DA) (10 µM) in serum-free 1,640 media for 20 min at 37 ℃ in a 5% CO2 culture chamber. Following this, the HBECs were washed thrice with PBS. The fluorescence intensity was measured with an Operetta High Content Screening System (PerkinElmer, USA), and Operetta Harmony 4.8 (PerkinElmer) analysis was conducted.
For the in vivo animal experiments, fresh lung tissues embedded in optimal cutting temperature compound (OCT) were precisely sliced and separately stained with dihydroethidium (DHE). DHE is naturally oxidized by superoxide, which emits red fluorescence and can be visualized through a fluorescence microscope. According to the manufacturer’s instructions, the levels of malondialdehyde (MDA) and hydrogen peroxide (H2O2) in the lung tissues were measured using a lipid peroxidation MDA kit and H2O2 kit, respectively.
Western blot
The harvested lung tissues and the cultured HBECs were lysed with Radio Immunoprecipitation Assay (RIPA) lysis buffer containing a chemical mixture including phenylmethanesulfonyl fluoride (PMSF), protease inhibitors, and a phosphatase inhibitor. An equal concentration of total protein was loaded onto the different lanes. The loaded proteins were separated based on their molecular weight by sodium dodecyl sulfate-polyacrylamide gel electrophoresis (SDS-PAGE). The separated proteins in the SDS-PAGE gel were then transferred to polyvinylidene difluoride (PVDF) membranes and incubated with primary antibodies at a specific diluted concentration. The transferred PVDF membranes were kept at 4 ℃ overnight, followed by incubation for 1 h at room temperature with the secondary antibody at a specific dilution. The immune-reactive bands were measured with an Enhanced chemiluminescence (ECL) reagent on a Bio-Rad gel imaging system (Bio-Rad, USA).
Enzyme-linked immunosorbent assay (ELISA)
For ELISA, the BAL was carefully collected through a tracheal cannula, with 1 ml of PBS instilled into the lung. The collected BAL was then centrifuged at 12,000 rpm for 15 min at 4 ℃. The levels of IL-6 and IL-8 in the BAL supernatants and cell culture supernatants were quantified using the indicated ELISA kits, according to the manufacturer’s instructions.
Statistical analysis
All of the data were expressed as mean ± SEM (Standard Error of Mean). In vitro experiments were conducted with n≥3 independent replicates, and the in vivo experiments were conducted with n=12 mice per group. Statistical analyses were performed using the GraphPad Prism program (GraphPad, San Diego, CA, USA). The Student’s t-test was employed for statistical analysis of significance. One-way ANOVA (Analysis of Variance) followed by Tukey’s post-hoc analysis was used to evaluate the statistical difference between multiple groups. A P value less than 0.05 was considered to be statistically significant.
Results
FGF10 is significantly upregulated upon PM exposure in vivo
To clarify the possible role of FGF10 in PM-induced lung injury, changes in FGF10 protein expression were evaluated after PM exposure in vivo. The schematic diagram of the in vivo experimental design is displayed in Figure 1A. We generated the mouse model of PM-induced lung injury. Western blot of the mouse lung tissues revealed upregulated expression of the FGF10 protein upon PM exposure (Figure 1B,1C). As FGF10 is typically a paracrine growth factor, the protein expression of FGF10 was consistently upregulated in BAL (Figure 1D). These findings suggested that the upregulated expression of the endogenous FGF10 protein represented a potential self-protection response against PM exposure. To confirm the potential role of FGF10 in PM-induced lung injury, intratracheal instillation of exogenous FGF10 was performed. A schematic diagram of the in vivo experimental design with intratracheal instillation of FGF10 is shown in Figure 1E.
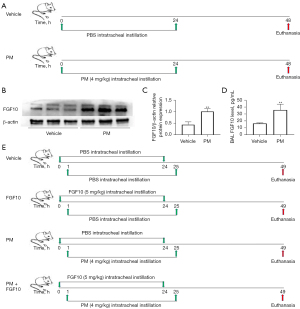
FGF10 attenuates PM-induced lung injury and suppresses NF-κB signaling
Exogenous FGF10 undoubtedly supplements the effect of endogenous FGF10. The in vivo experimental plan with intratracheal administration of FGF10 was carried out. The histopathological analyses of lung sections stained with H&E revealed that PM exposure led to a marked infiltration of inflammatory cells clustered around the PM deposits, mainly restricted to the peripheral bronchi. However, the intratracheal instillation of FGF10 exhibited a pronounced reduction in inflammatory cells around the PM deposits (Figure 2A).
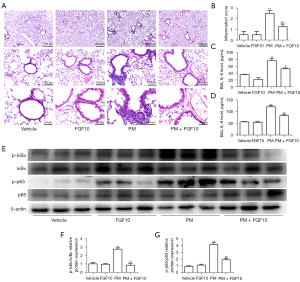
Next, the semi-quantified inflammation score for the lung sections stained with H&E was evaluated by three independent investigators. Consistent with the histopathological analyses, the intratracheal instillation of FGF10 reversed the inflammation score in the group exposed to PM. Also, no significant difference was observed between the vehicle and FGF10 groups (Figure 2B).
The protein expression of inflammatory cytokines, IL-6 and IL-8, from the BAL were quantified by ELISA. Compared to the vehicle group, PM exposure notably upregulated the protein expression of IL-6 and IL-8, whereas FGF10 pre-treatment significantly reduced the protein expression of IL-6 and IL-8 (Figure 2C,2D). The redox-sensitive NF-κB signaling is also known to participate in PM-induced lung injury, which is closely related to the production of inflammatory cytokines such as IL-6 and IL-8. Western blot of lung tissues showed that PM exposure resulted in the phosphorylation of IκBαof lung tissues showed pre-treatment significantly inhibited NF-κo activation (Figure 2E-2G). These findings suggested that FGF10 played a suppressive role in oxidative stress and NF-κB signaling. Collectively, these results indicated that FGF10 played a protective role in PM-induced lung injury.
FGF10 suppresses oxidative stress and activates Nrf2 signaling in vivo
Oxidative stress is the critical regulator of PM-induced lung injury. However, the antioxidant role of FGF10 remains to be demonstrated. The DHE staining of lung sections illustrated that ROS production was markedly higher in the PM group than in the vehicle group. However, FGF10 pre-treatment effectively blocked ROS production (Figure 3A,3B). Simultaneously, the levels of MDA and H2O2, which are sensitive biomarkers that reflect the cellular redox status, were reversed with FGF10 pre-treatment (Figure 3C,3D), highlighting the antioxidant effect of FGF10 in PM-induced lung injury.
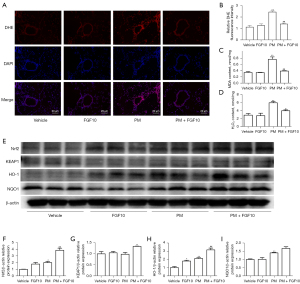
Nrf2 signaling represents an intrinsic cytoprotective mechanism against oxidative stress. Western blot of the lung tissues showed that FGF10 pre-treatment significantly activated Nrf2 signaling, as evidenced by the upregulation of the downstream mediators of Nrf2 signaling, namely, HO-1 and NQO1 (Figure 3E-3I). These findings suggested that FGF10 suppressed oxidative stress, which was reflected by the activation of Nrf2 signaling.
FGF10 exerts anti-inflammatory effects via modulation of NF-κB signaling in HBECs
To further validate the cytoprotective role of FGF10, the HBECs were subjected to 200 µg/mL PM alone or in combination with 10 ng/mL FGF10. The ELISA results for cell culture supernatants showed elevated protein expression of IL-6 and IL-8 with PM exposure, which was reversed by FGF10 pre-treatment (Figure 4A,4B). It is known that NF-κB signaling is the central inflammatory signaling responsible for the expression and secretion of IL-6 and IL-8 in the PM-induced inflammatory response. Therefore, to determine the effect of FGF10 on NF-κB signaling in the PM-induced inflammatory response, BAY11-7082, a synthetic chemical inhibitor of NF-κB, was selected for further experiments. This NF-κB inhibitor was found to exert similar effects as FGF10 (Figure 4C,4D). Moreover, pre-treatment with FGF10 reversed the effects on NF-κB signaling induced by PM exposure (Figure 4E-4G). Collectively, these results suggested that PM exposure promoted the NF-κB signaling-dependent inflammatory response; meanwhile, FGF10 reversed the inflammatory effects of PM exposure on NF-κB signaling.
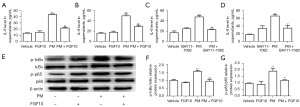
The anti-oxidative effect of FGF10 on ROS production and the modulation of NF-κB signaling by the antioxidant NAC exert anti-inflammatory effects in HBECs
The regulatory mechanism of FGF10 on NF-κB signaling remains to be elucidated. ROS, as the representative biomarker of oxidative stress, promotes various cellular processes including the activation of NF-κB signaling. Fluorescence staining of ROS in the HBECs showed that PM exposure caused an elevation in ROS levels, while FGF10 pre-treatment reduced ROS production upon PM exposure (Figure 5A,5B).
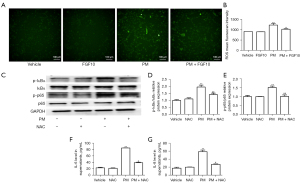
To outline the direct phosphorylation of proteins involved in NF-κB signaling by ROS, the antioxidant NAC was utilized as an intervention upon PM exposure. Western blot analysis pointed out that the phosphorylation of p65 and IκBα was reduced after NAC pre-treatment compared to the PM group (Figure 5C-5E). PM exposure led to significant upregulation of p-p65 and p-IκBα and NAC effectively reversed upregulated p-p65 and p-IκBα. Consistent with the suppression of NF-κB signaling, NAC pre-treatment also suppressed the expression of IL-6 and IL-8 (Figure 5F,5G). These findings implied that ROS partially mediated the activation of NF-κB signaling in the PM-induced inflammatory response, and FGF10 potentially functioned as a scavenger of ROS to mediate its anti-inflammatory effects.
Effect of FGF10 on the activation and expression of Nrf2 signaling in HBECs
Considering that FGF10 is not a direct antioxidant like NAC, the anti-oxidative effect of FGF10 needs to be further explored. The anti-oxidative effect of Nrf2 signaling is reportedly the critical pathway protecting against oxidative stress. Therefore, we explored the relationship between FGF10 and Nrf2 signaling. As illustrated in Figure 6A-6E, PM exposure resulted in elevated expression of Nrf2, reflecting a self-protecting cellular mechanism. However, the protein expression of Nrf2 was highest upon PM exposure when combined with FGF10 pre-treatment. Similarly, the expression of HO-1 and NQO1 were also highest when PM exposure was combined with FGF10 pre-treatment.
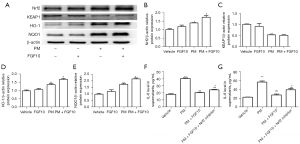
We utilized the specific Nrf2 inhibitor, ML385, to further validate the regulatory effects of FGF10 on Nrf2 signaling. It was found that ML385 pre-treatment abolished the rescue of IL-6 and IL-8 expression by FGF10 upon PM exposure (Figure 6F,6G). These findings demonstrated that Nrf2 signaling was critical for the protective effect of FGF10 in the PM-induced inflammatory response.
Discussion
As a consequence of the widespread health-related concerns associated with PM, numerous efforts have been deployed to determine the molecular mechanism of PM-induced lung injury to identify potential therapeutic targets. PM exposure leads to lung injury primarily through the inflammatory mechanism mediated by oxidative stress. It is recognized that oxidative stress, owing to the excess production of ROS and insufficient synthesis of the antioxidant enzymes, is a pivotal pathophysiological characteristic of PM-induced lung injury (20). NF-κB signaling is a well-known redox-sensitive signaling that mediates inflammation. Under oxidative stress conditions, elevated levels of ROS stimulate the redox-sensitive NF-κB signaling, which promotes irreversible cellular injury and the secretion of inflammatory mediators (21,22). In contrast to the inflammatory NF-κB signaling, the redox-sensitive Nrf2 signaling represents a finitely self-protective mechanism (23).
As for the significant role of oxidative stress, efforts toward the reversal of oxidative stress potentially provide an effective strategy against PM-induced lung injury. Although the ROS scavenger NAC is a promising therapeutic agent, the elevation in mesenchyme-derived FGF10 upon PM exposure is more intriguing. FGF10 plays a pivotal role in organogenesis, repair after lung injury and regarded as a therapy to repair impaired epithelium. Considering the multifunctional effects of FGF10 in the repair process associated with lung injury (24,25), the elevated levels of FGF10 are consistent with the body’s self-repair mechanism against PM exposure. As a complement to the reported regulatory effect on HMGB1-TLR4 signaling and reversal of endoplasmic reticulum stress (15,16), further investigation of the anti-oxidative effects of FGF10 and the underlying molecular mechanism would certainly broaden our current understanding of the multifunctional effects of FGF10 and validate FGF10 as a novel therapeutic target for treating PM-induced lung injury.
For the in vivo studies, intratracheal instillation of PM was performed for 2 consecutive days to establish a model of short-term PM exposure, as previously reported (26,27). As PM is a chemical mixture and the composition varies with the geographical area and season, commercial PM with its settled constituents was purchased to guarantee experimental reproducibility. Consistent with previous findings, intratracheal instillation of PM promoted bronchitis but not alveolitis, with significant elevation of endogenous FGF10. This result contrasts with the observation in humans that PM exposure promotes both bronchitis and alveolitis. We speculated that the currently used method for the intratracheal instillation of PM might impair the intrinsic aerodynamics of PM. We also speculated that the levels of available FGF10 in the lung positively impact the outcome of PM-induced lung injury (28).
To further clarify this, recombinant FGF10 was employed as an exogenous supplementation. As expected, the exogenous FGF10 markedly relieved the degree of lung injury, as evidenced by the histopathological examinations and secreted inflammatory mediators. Simultaneously, the production of oxygen-free radicals and ROS as well as the synthesis of antioxidant entities were investigated to measure the redox state. Oxidative stress is considered one of the most prevalent mechanisms in the pathogenesis of lung injury, and PM is the primary inducer of excessive ROS, promoting mitochondrial dysfunction (29). It was shown that the exogenous FGF10 reversed ROS generation upon PM exposure, together with the downregulation of oxidative stress markers, such as MDA and H2O2.
NF-κB signaling is related to the secretion of inflammatory mediators such as IL-6 and IL-8 and contributes to the development of lung injury (8). As for the regulation of NF-κB signaling by FGF10, it has been reported that FGF10 derived from the neuron and microglia/macrophages inhibits the NF-κB-dependent neuroinflammation through FGFR2/PI3K signaling, which accelerates the recovery from spinal cord injury (30). Furthermore, neuro-derived FGF10 inhibits NF-κB-dependent neuro-inflammation by activating the PI3K survival signaling, which was reported to improve cerebral ischemia injury (31). A similar inhibitory effect of FGF10 on NF-κB signaling was observed in the current study.
Wang et al. (8) discovered the role of ROS-dependent NF-κB signaling in lung inflammation upon PM exposure. Consistent with the findings of Wang et al. (8), the inhibition of NF-κB signaling was related to the anti-oxidative effects of FGF10 on the activation of Nrf2 signaling. These findings synergistically revealed that FGF10 exerted protective anti-oxidative activity in response to PM exposure and anti-inflammatory effects through the inhibition of NF-κB signaling. However, it should be noted that the anti-oxidative effect of FGF10 is likely different from that elicited by the direct antioxidant, NAC. The molecular mechanism mediating the protective anti-oxidative property of FGF10 remains to be elucidated.
Nrf2 signaling is a crucial regulator of redox homeostasis, which counterbalances excessive ROS production. Nrf2 is physically located in the cytoplasm through binding to KEAP1. Upon stimulation of Nrf2 signaling and with the degradation of KEAP1, Nrf2 spontaneously translocates into the nucleus to further bind to anti-oxidative response elements, thereby regulating the expression of genes encoding antioxidant enzymes such as HO-1 and NQO1 (32,33). The regulatory mechanism of Nrf2 signaling is complex, as redox-sensitive signaling is potently induced by oxidative stress.
Also, the regulatory effect of FGF10 on Nrf2 signaling has been discovered both in peripheral nerve regeneration and hepatic ischemia-reperfusion injury (17,18). It has been shown that the protein expressions of Nrf2, along with that of its downstream mediators, HO-1 and NQO1, were slightly upregulated with PM exposure, highlighting the regulatory effect of oxidative stress on Nrf2 signaling. Others have reported conflicting findings, namely, that PM exposure suppresses Nrf2 signaling and downregulates the expression of downstream anti-oxidative genes, thus leading to excessive intracellular ROS (34,35). The causal relationship between oxidative stress and Nrf2 signaling in the context of PM is not direct, and the contradiction might be dosage-dependent. The activated Nrf2 signaling is regarded as a compensatory mechanism. However, it was not sufficient to counteract the oxidative lung injury. Exogenous FGF10 further amplified Nrf2 signaling to the degree that led to reduced oxidative lung injury. It should be noted that the upregulated expression of KEAP1 was controversial to the activated Nrf2 signaling in vivo. The downstream HO-1 and NQO1 are more sensitive indicators of the state of Nrf2 signaling. These results highlighted that the lungs of PM-exposed mice were in a state of oxidative stress and that the protective role of FGF10 was at least partially attributed to the amplified Nrf2-mediated antioxidant effect.
In the pathophysiology of PM-induced lung injury, notably bronchitis, the bronchial epithelial cells act as both the first line of defense against chemical and biological pathogens and also as the initiators of secondary inflammation (36). FGF10 is pivotal in regenerative medicine, and it binds to the specific epithelial receptor FGFR2b (37). As bronchial epithelial cells are the primary cells regulating FGF10 expression and also represent its principal cellular target, it is speculated that the protective effect of FGF10 is mediated through the regulation of bronchial epithelial cells (38). Based on the anti-oxidative effects of FGF10 in vivo, immortalized human bronchial epithelial cells were selected to validate the hypothesis in vitro. For the in vitro experiments, pre-treatment of bronchial epithelial cells with exogenous FGF10 was performed to mimic the in vivo mesenchymal-epithelial FGF10 signaling. In contrast to the in vivo setting, the ROS scavenger NAC and Nrf2 inhibitor were employed. Both FGF10 and NAC have an inhibitory effect on the PM-induced phosphorylation of NF-κB signaling and the subsequent secretion of Il-6 and IL-8. It has been reported that IL-6 and IL-8 are the target genes regulated by NF-κB signaling. NAC neutralizes excessive ROS to exert anti-inflammatory effects, while FGF10 is not a first-line antioxidant.
To validate the Nrf2-mediated anti-oxidative impact of FGF10 on NF-κB signaling, it was found that the anti-inflammatory effect of FGF10 was abolished when FGF10 and the Nrf2 inhibitor were combined. However, the mediators linking FGF10-FGFR2b and Nrf2 signaling require further exploration. It was reported that FGF10/Akt/Nrf2 signaling is critical in oxidative stress-mediated myocardial ischemia-reperfusion injury (39). Moreover, the protective effect of FGF10 in hepatic ischemia-reperfusion injury relies on PI3K/Akt-dependent Nrf2 activation (18). Therefore, it is speculated that PI3K/Akt signaling might be the mediator between FGF10 and Nrf2 signaling.
Although the current study identified the protective role of FGF10 and the anti-oxidative mechanism it promoted, there were several limitations that should be noted. Firstly, the PM-induced lung injury was confined to bronchitis. In the future, PM aerosolization will be performed instead of intratracheal instillation, as such a delivery route will likely also induce alveolitis. Secondly, while exogenous FGF10 was intratracheally instilled to magnify the effect of endogenous FGF10, the contribution of endogenous FGF10 signaling to the repair process remains unclear. Research on the regulation of FGF10 by lncRNA or miRNA would make the whole study more complete.
Further loss of function experiments to delete Fgfr2b expression in the bronchial epithelium or abrogate Fgf10 mesenchymal expression will be conducted. Simultaneously, more oxidative stress and inflammatory indicators should be adopted to reveal the pathogenesis of PM-induced lung injury and pleiotropic effect of FGF10. Thirdly, this study lacks clinical specimens such as primary bronchial epithelial cells, which could be collected for cell-based experiments. Therefore, further studies must be carried out to determine the anti-oxidative mechanism associated with FGF10, and in particular to understand its complex regulatory network.
In conclusion, the current study demonstrated the protective effect of FGF10 in PM-induced lung injury. The Nrf2-mediated anti-oxidative effects of FGF10 were responsible for the inhibition of NF-κB signaling and contributed to confining PM-induced lung injury (Figure 7). The current study broadens our understanding of the multifunctional effects of FGF10 and identified FGF10 as a novel antioxidant with therapeutic potential against PM-induced lung injury.
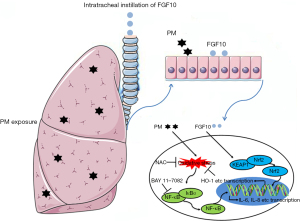
Acknowledgments
Funding: The work was supported by the Zhejiang Provincial Science Technology Department Foundation (Nos. 2020RC080 and 2022RC203, to Nian Dong) and Wenzhou Technology Foundation (No. Y20220035, to Nian Dong).
Footnote
Reporting Checklist: The authors have completed the ARRIVE reporting checklist. Available at https://atm.amegroups.com/article/view/10.21037/atm-22-4389/rc
Data Sharing Statement: Available at https://atm.amegroups.com/article/view/10.21037/atm-22-4389/dss
Conflicts of Interest: All authors have completed the ICMJE uniform disclosure form (available at https://atm.amegroups.com/article/view/10.21037/atm-22-4389/coif). ND reports that the work was supported by the Zhejiang Provincial Science Technology Department Foundation (Nos. 2020RC080 and 2022RC203) and Wenzhou Technology Foundation (No. Y20220035). The other authors have no conflicts of interest to declare.
Ethical Statement: The authors are accountable for all aspects of the work in ensuring that questions related to the accuracy or integrity of any part of the work are appropriately investigated and resolved. Animal experiments were performed under a project license (No. 2021-0037) granted by the ethics committee for animal welfare at The First Affiliated Hospital of Wenzhou Medical University, in compliance with national guidelines for the care and use of animals.
Open Access Statement: This is an Open Access article distributed in accordance with the Creative Commons Attribution-NonCommercial-NoDerivs 4.0 International License (CC BY-NC-ND 4.0), which permits the non-commercial replication and distribution of the article with the strict proviso that no changes or edits are made and the original work is properly cited (including links to both the formal publication through the relevant DOI and the license). See: https://creativecommons.org/licenses/by-nc-nd/4.0/.
References
- Guo C, Lv S, Liu Y, et al. Biomarkers for the adverse effects on respiratory system health associated with atmospheric particulate matter exposure. J Hazard Mater 2022;421:126760. [Crossref] [PubMed]
- Han B, Xu J, Zhang Y, et al. Associations of Exposure to Fine Particulate Matter Mass and Constituents with Systemic Inflammation: A Cross-Sectional Study of Urban Older Adults in China. Environ Sci Technol 2022;56:7244-55. [Crossref] [PubMed]
- Song C, Liu L, Chen J, et al. Evidence for the critical role of the PI3K signaling pathway in particulate matter-induced dysregulation of the inflammatory mediators COX-2/PGE2 and the associated epithelial barrier protein Filaggrin in the bronchial epithelium. Cell Biol Toxicol 2020;36:301-13. [Crossref] [PubMed]
- Nishida C, Yatera K. The Impact of Ambient Environmental and Occupational Pollution on Respiratory Diseases. Int J Environ Res Public Health 2022;19:2788. [Crossref] [PubMed]
- Michael S, Montag M, Dott W. Pro-inflammatory effects and oxidative stress in lung macrophages and epithelial cells induced by ambient particulate matter. Environ Pollut 2013;183:19-29. [Crossref] [PubMed]
- Nozza E, Valentini S, Melzi G, et al. Advances on the immunotoxicity of outdoor particulate matter: A focus on physical and chemical properties and respiratory defence mechanisms. Sci Total Environ 2021;780:146391. [Crossref] [PubMed]
- Piao CH, Fan Y, Nguyen TV, et al. PM2.5 Exacerbates Oxidative Stress and Inflammatory Response through the Nrf2/NF-κB Signaling Pathway in OVA-Induced Allergic Rhinitis Mouse Model. Int J Mol Sci 2021;22:8173. [Crossref] [PubMed]
- Wang J, Huang J, Wang L, et al. Urban particulate matter triggers lung inflammation via the ROS-MAPK-NF-κB signaling pathway. J Thorac Dis 2017;9:4398-412. [Crossref] [PubMed]
- Ge C, Hu L, Lou D, et al. Nrf2 deficiency aggravates PM2.5-induced cardiomyopathy by enhancing oxidative stress, fibrosis and inflammation via RIPK3-regulated mitochondrial disorder. Aging (Albany NY) 2020;12:4836-65. [Crossref] [PubMed]
- Chu CC, Chen SY, Chyau CC, et al. Djulis (Chenopodium formosanum) and Its Bioactive Compounds Protect Human Lung Epithelial A549 Cells from Oxidative Injury Induced by Particulate Matter via Nrf2 Signaling Pathway. Molecules 2021;27:253. [Crossref] [PubMed]
- Yang S, Huang XL, Chen J, et al. Curcumin protects BEAS-2B cells from PM2.5-induced oxidative stress and inflammation by activating NRF2/antioxidant response element pathways. Int J Mol Med 2021;47:45. [Crossref] [PubMed]
- Cheng L, Li F, Ma R, et al. Forsythiaside inhibits cigarette smoke-induced lung inflammation by activation of Nrf2 and inhibition of NF-κB. Int Immunopharmacol 2015;28:494-9. [Crossref] [PubMed]
- Wu J, Chu X, Chen C, et al. Role of Fibroblast Growth Factor 10 in Mesenchymal Cell Differentiation During Lung Development and Disease. Front Genet 2018;9:545. [Crossref] [PubMed]
- Yuan T, Volckaert T, Redente EF, et al. FGF10-FGFR2B Signaling Generates Basal Cells and Drives Alveolar Epithelial Regeneration by Bronchial Epithelial Stem Cells after Lung Injury. Stem Cell Reports 2019;12:1041-55. [Crossref] [PubMed]
- Shi Q, Wang Q, Liu L, et al. FGF10 protects against particulate matter (PM)-induced lung injury via regulation of endoplasmic reticulum stress. Int Immunopharmacol 2022;105:108552. [Crossref] [PubMed]
- Liu L, Song C, Li J, et al. Fibroblast growth factor 10 alleviates particulate matter-induced lung injury by inhibiting the HMGB1-TLR4 pathway. Aging (Albany NY) 2020;12:1186-200. [Crossref] [PubMed]
- Dong L, Li R, Li D, et al. FGF10 Enhances Peripheral Nerve Regeneration via the Preactivation of the PI3K/Akt Signaling-Mediated Antioxidant Response. Front Pharmacol 2019;10:1224. [Crossref] [PubMed]
- Li S, Zhu Z, Xue M, et al. The protective effects of fibroblast growth factor 10 against hepatic ischemia-reperfusion injury in mice. Redox Biol 2021;40:101859. [Crossref] [PubMed]
- Lee KS, Lee HK, Hayflick JS, et al. Inhibition of phosphoinositide 3-kinase delta attenuates allergic airway inflammation and hyperresponsiveness in murine asthma model. FASEB J 2006;20:455-65. [Crossref] [PubMed]
- Quezada-Maldonado EM, Sánchez-Pérez Y, Chirino YI, et al. Airborne particulate matter induces oxidative damage, DNA adduct formation and alterations in DNA repair pathways. Environ Pollut 2021;287:117313. [Crossref] [PubMed]
- Lee H, Park C, Kwon DH, et al. Schisandrae Fructus ethanol extract attenuates particulate matter 2.5-induced inflammatory and oxidative responses by blocking the activation of the ROS-dependent NF-κB signaling pathway. Nutr Res Pract 2021;15:686-702. [Crossref] [PubMed]
- Ziegler K, Kunert AT, Reinmuth-Selzle K, et al. Chemical modification of pro-inflammatory proteins by peroxynitrite increases activation of TLR4 and NF-κB: Implications for the health effects of air pollution and oxidative stress. Redox Biol 2020;37:101581. [Crossref] [PubMed]
- Deng X, Rui W, Zhang F, et al. PM2.5 induces Nrf2-mediated defense mechanisms against oxidative stress by activating PIK3/AKT signaling pathway in human lung alveolar epithelial A549 cells. Cell Biol Toxicol 2013;29:143-57. [Crossref] [PubMed]
- Upadhyay D, Panduri V, Kamp DW. Fibroblast growth factor-10 prevents asbestos-induced alveolar epithelial cell apoptosis by a mitogen-activated protein kinase-dependent mechanism. Am J Respir Cell Mol Biol 2005;32:232-8. [Crossref] [PubMed]
- Braun S, auf dem Keller U, Steiling H, et al. Fibroblast growth factors in epithelial repair and cytoprotection. Philos Trans R Soc Lond B Biol Sci 2004;359:753-7. [Crossref] [PubMed]
- Xu F, Cao J, Luo M, et al. Early growth response gene 1 is essential for urban particulate matter-induced inflammation and mucus hyperproduction in airway epithelium. Toxicol Lett 2018;294:145-55. [Crossref] [PubMed]
- Wu YF, Li ZY, Dong LL, et al. Inactivation of MTOR promotes autophagy-mediated epithelial injury in particulate matter-induced airway inflammation. Autophagy 2020;16:435-50. [Crossref] [PubMed]
- Marega M, Chen C, Bellusci S. Cross-Talk Between Inflammation and Fibroblast Growth Factor 10 During Organogenesis and Pathogenesis: Lessons Learnt From the Lung and Other Organs. Front Cell Dev Biol 2021;9:656883. [Crossref] [PubMed]
- Tao S, Zhang H, Xue L, et al. Vitamin D protects against particles-caused lung injury through induction of autophagy in an Nrf2-dependent manner. Environ Toxicol 2019;34:594-609. [Crossref] [PubMed]
- Chen J, Wang Z, Zheng Z, et al. Neuron and microglia/macrophage-derived FGF10 activate neuronal FGFR2/PI3K/Akt signaling and inhibit microglia/macrophages TLR4/NF-κB-dependent neuroinflammation to improve functional recovery after spinal cord injury. Cell Death Dis 2017;8:e3090. [Crossref] [PubMed]
- Li YH, Fu HL, Tian ML, et al. Neuron-derived FGF10 ameliorates cerebral ischemia injury via inhibiting NF-κB-dependent neuroinflammation and activating PI3K/Akt survival signaling pathway in mice. Sci Rep 2016;6:19869. [Crossref] [PubMed]
- Pardo M, Qiu X, Zimmermann R, et al. Particulate Matter Toxicity Is Nrf2 and Mitochondria Dependent: The Roles of Metals and Polycyclic Aromatic Hydrocarbons. Chem Res Toxicol 2020;33:1110-20. [Crossref] [PubMed]
- Zhang H, Xue L, Li B, et al. Therapeutic potential of bixin in PM2.5 particles-induced lung injury in an Nrf2-dependent manner. Free Radic Biol Med 2018;126:166-76. [Crossref] [PubMed]
- Jia G, Yu S, Sun W, et al. Hydrogen Sulfide Attenuates Particulate Matter-Induced Emphysema and Airway Inflammation Through Nrf2-Dependent Manner. Front Pharmacol 2020;11:29. [Crossref] [PubMed]
- Guohua F, Tieyuan Z, Xinping M, et al. Melatonin protects against PM2.5-induced lung injury by inhibiting ferroptosis of lung epithelial cells in a Nrf2-dependent manner. Ecotoxicol Environ Saf 2021;223:112588. [Crossref] [PubMed]
- Becker S, Mundandhara S, Devlin RB, et al. Regulation of cytokine production in human alveolar macrophages and airway epithelial cells in response to ambient air pollution particles: further mechanistic studies. Toxicol Appl Pharmacol 2005;207:269-75. [Crossref] [PubMed]
- Itoh N. FGF10: A multifunctional mesenchymal-epithelial signaling growth factor in development, health, and disease. Cytokine Growth Factor Rev 2016;28:63-9. [Crossref] [PubMed]
- Yuan T, Volckaert T, Chanda D, et al. Fgf10 Signaling in Lung Development, Homeostasis, Disease, and Repair After Injury. Front Genet 2018;9:418. [Crossref] [PubMed]
- Zheng T, Yang J, Zhang J, et al. Downregulated MicroRNA-327 Attenuates Oxidative Stress-Mediated Myocardial Ischemia Reperfusion Injury Through Regulating the FGF10/Akt/Nrf2 Signaling Pathway. Front Pharmacol 2021;12:669146. [Crossref] [PubMed]
(English Language Editor: A. Kassem)