Role of reactive oxygen species in high concentration glucose-induced growth inhibition of human peritoneal mesothelial cells
Introduction
Peritoneal dialysis (PD) is an effective life-sustaining treatment for patients with end-stage renal disease. The peritoneum may become dysfunctional as a dialysis organ over time because of progressive membrane injury. As a result of prolonged exposure to bioincompatible PD fluid (PDF) that contains high levels of glucose and degradation products of glucose, low pH, and high osmolality, peritonitis develops (1). Human peritoneal mesothelial cells (HPMCs) play an important role in peritoneal membrane injury (2). Hypertrophy and arrest of the cell cycle induced by high concentration glucose (HG) in renal mesangial cells in the early stage of diabetic nephropathy, which might be one of the factors of the initiation or progression of the disease (3). A recent study demonstrated that HG inhibits the growth of HPMCs (4). Another study showed that HG causes hypertrophy, senescence, cell cycle arrest, apoptosis, and necrosis, epithelial-to-mesenchymal transition (EMT), peritoneal angiogenesis, which are detrimental to continuous ambulatory PD (CAPD) patients (5,6). Regarding the mechanism of HG cytotoxicity, the oxidative stress state induced by reactive oxygen species (ROS) has been the subject of increasing interest for CAPD patients (7). There is not only increased generation of ROS by mesothelial cells but also by peritoneal phagocytes. ROS can damage HPMCs, but the mechanism of damage remains unclear. ROS can inhibit cell growth and induce cell cycle arrest in other types of cells, such as mesangial and proximal tubular cells (3). Belonging to the CIP/KIP family of cyclin-dependent kinase inhibitors (CKIs), p21Waf1 and p27kip1 are negative regulators of the cell cycle. Some effects of HG have been associated with increased expression of p21Waf1 (8). Additionally, mammalian cells produce hydrogen peroxide (H2O2), in low concentrations, H2O2 plays an important role in cell proliferation, immunity, and metabolism. However, higher non-physiologic concentrations of H2O2 can cause oxidative stress. Stress can lead to cell death if it is not alleviated (9). H2O2 is involved in various processes including inducing hypertrophic and senescent phenotypes in some cell types, arresting the cell cycle, and increasing the expression of p21Waf1 and p27Kip1 (10-12). Presently, there is no report on the reciprocal effects of these factors and the role of ROS in HG-induced changes in the cell cycle and growth of HPMCs. Therefore, we designed this preliminary study to explore these correlations. We present the following article in accordance with the MDAR reporting checklist (available at https://atm.amegroups.com/article/view/10.21037/atm-22-4352/rc).
Methods
Cell culture
A HPMC line, HMrSV5 (kindly provided by Prof. Pierre Ronco, Hospital Tenon, Paris, France), was cultured in Dulbecco’s modified Eagle’s medium (DMEM; Gibco, NY, USA) supplemented with 10% fetal calf serum (FCS; Gibco, NY, USA), 20 mmol/L hydroxyethyl piperazine ethane sulfonic acid (HEPES; Gibco, NY, USA), and 2 mmol/L L-glutamine at 37 ℃ in a humidified atmosphere with 5% CO2.
Research design
The study was divided into 2 parts—part A and part B—to observe the effects of HG and H2O2 purchased from Nanjing Chemical Reagent (Nanjing, China) on HPMCs, respectively. All the test solutions used DMEM for all stimulations.
Part A comprised 8 groups: group a1 with 0.1% glucose in DMEM (G0) as the control group, group a2 with 1.35% glucose (1.35G), group a3 with 3.86% glucose (3.86G), group a4 with 3.86% mannitol (MCE, Shanghai, China) (3.86M), group a5 with pyruvate 35 mmol/L in DMEM (G0 + P), group a6 with 3.86% glucose and pyruvate (MCE) 35 mmol/L (3.86G + P), group a7 with catalase 500 U/L (G0 + C), and group a8 with 3.86% glucose and catalase 500 U/L in DMEM (3.86G + C). Part B consisted of 6 groups: group b1 with H2O2 0.5 mmol/L in DMEM (G0 + 0.5H), group b2 with H2O2 0.1 mmol/L (G0 + 0.1H), group b3 with H2O2 0.5 mmol/L and 3.86% glucose (3.86G + 0.5H), group b4 with H2O2 0.1 mmol/L and 3.86% glucose (3.86G + 0.1H), group b5 with H2O2 0.5 mmol/L, 3.86% glucose, and pyruvate 35 mmol/L (3.86G + 0.5H + P), group b6 with 0.1% glucose (G0, control group). Unless noted otherwise, the stimulation time was 24 and 12 h in part A and B, respectively. Each experiment was performed with 3 independent replicates.
Morphological observation
The HPMCs were harvested from culture flasks by trypsin digestion and centrifugation as described previously,and inoculated into a 24-well culture plate at 6.0×104 cells/well and grown at 37 ℃ in a 5% CO2 incubator. When the cells reached to logarithmic phase, incubated with 0.1% glucose, 3.86% glucose, and 0.5 mmol/L H2O2. Then, the morphological changes were observed under a phase-contrast microscope 24 h post-stimulation.
Flow cytometric analysis
Cells of groups a1–a6 and b1–b6 were assessed by flow cytometry 12–24 h post-incubation. Subsequently, the cells were washed with phosphate-buffered saline (PBS), trypsinized, fixed with 70% ethanol at 4 ℃ overnight, and stained with propidium iodide (PI) for 30 min. Then, 106 cells/mL in each group were harvested and analyzed on a FACS Epics XL Flow Cytometer (Coulter) to elucidate cell cycle distribution and calculate the proportion of cells in the G1 phase.
Cell proliferation studies with 3H-thymidine incorporation
The proliferation of the HPMCs was estimated indirectly based on the incorporation of 3H-thymidine into growing cells that were seeded in a 96-well plate at a density of 103 cells/well. After 48 h, the cells were exposed to the test solutions (groups a1–a8 and b1–b6, i.e., 14 groups) for 24 h. Subsequently, 3H-thymidine (1 Ci/well, procured from Chinese Science Academy, Shanghai Institute of Nuclear Research) was added for an additional 6-h incubation period. Then, the test solutions were discharged, and the cells were trypsinized and collected on a filter paper using a semi-automatic cell collector (type of DYQIII). The filter papers were placed into the tube, and the scintillation reagent was added to count the samples in a beta counter (Pharmacia).
Reverse-transcription polymerase chain reaction (RT-PCR)
Total RNA was extracted from HPMCs in the a1–a6 and b1–b6 groups using TRIzol as stated by the protocol. An equivalent of 2 µg of RNA was reversed transcribed into complementary DNA (cDNA) using M-MLV reverse transcriptase (Gibco, NY, USA). PCR amplification was performed in a total volume of 50 µL using the following protocol: 94 ℃ for 5 min, 25 cycles of 94 ℃ for 1 min, 65 ℃ (p21) for 30 s, 58 ℃ (GAPDH) for 30 s, 72 ℃ for 1 min, and finally, 72 ℃ for 7 min. The PCR products were separated by 2% agarose gel electrophoresis with 0.5 µg/mL ethidium bromide and photographed. Bands were scanned using a densitometer (Gensnap), and band density was compared to that of the housekeeping gene. The sequences of the amplification primers were as follows: p21Waf1 (sense: 5'-CAGGGGACAGCAGAGGAAGA-3', antisense 5'-GGGCGGCCAGGGTATGTAC-3'), p27Kip1 (sense: 5'-ATGTCAAACGTGCGAGTGTC-3', antisense: 5'-TCTGTAGTAGAACTCGGGCAA-3'), GAPDH (sense: 5'-CCACCCATGGCAAATTCCATGGCA-3', antisense: 5'-TCTAGAGGGCAGGTCAGGTCCACC-3').
Immunocytochemistry (ICC)
HPMCs were grown on 1×1 cm2 slides and stimulated with various test solutions (groups a1–a8 and b1–b6) for 12–24 h. Then, the stimulations were removed, and the cell layer was rinsed with PBS. Subsequently, the cells were fixed with freshly prepared 4% paraformaldehyde at room temperature for 20 min. Then, the cells were rehydrated with PBS and incubated with a primary antibody (mouse anti-human p21Waf1 or rabbit anti-human p27Kip1) for 4 ℃ overnight (1:100 dilution, Santa Cruz, CA, USA). After washing with PBS, the cells were incubated with horseradish peroxidase (HRP)-conjugated secondary antibody (37 ℃ for 2 h), and the immune reaction was detected by 3,3'-diaminobenzidine (DAB). The nuclei were counterstained with hematine. Computer software was used for semiquantitative analysis to calculate the proportion of positive cells.
Western blot
Groups a1, a3, a6, and b3 were stimulated in a 90-mm dish for 24 h. Then total cellular proteins extracted from the HPMCs, quantified, separated by sodium dodecyl sulfate (SDS)-polyacrylamide gel electrophoresis (PAGE). After electrophoresis and transfer (nitrocellulose membrane, Bio-Rad, Amresco), the polyvinylidene fluoride (PVDF) membranes were blocked in 5% milk prior and probed with mouse monoclonal antibody to human p21Waf1 and rabbit anti-human p27Kip1 (Santa Cruz, CA, USA). This was followed by incubation with the secondary antibody, namely HRP-conjugated goat anti-mouse IgG (Yeasen, Shanghai, China). Finally, the membranes were developed using enhanced chemiluminescence (ECL) blotting substrate (Amersham, USA) and exposed to X-ray film. Glyceraldehyde 3-phosphate dehydrogenase (GAPDH) was used as a control. The immunoreactive band intensity was measured on an image analysis system (Genesnap).
Statistical analysis
Data are shown as the mean ± standard error of the mean (SEM). Each experiment was repeated at least 3 times. Student’s t-test or one-way analysis of variance (ANOVA) were used for statistical analysis using SPSS 10 software (IBM Corporation, Armonk, NY, USA). P<0.05 was considered statistically significant.
Results
Morphological observations
Morphological changes were observed after 24-h stimulation. The HPMCs in group G0 (control group) showed a typical polygonal and cobblestone monolayer appearance when grown to confluency. In the 3.86G group, the nucleolus was obvious, with the appearance of poly-nuclei and hypertrophy of the cell. In the 0.5 mmol/L H2O2 group, the changes were similar to that in the HG group.
Effects of various stimulations on cell growth (proliferation)
No significant difference in cell growth was observed among group a1 (G0), a5 (G0 + P), and a7 (G0 + C), and the count per minute (CPM) was higher than that in group a2 (1.35G) or a3 (3.86G). No difference was detected between the cell numbers in group a6 (3.86G + P) and a8 (3.86G + C), but both had significantly higher cell numbers than group a3 (Figure 1A).
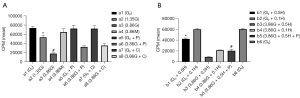
A high concentration of H2O2 (0.5 mmol/L) inhibited the proliferation of HPMCs, which was not demonstrated with the 0.1 mmol/L dose (b2 vs. b6, P>0.05). This effect was accentuated by HG conditions (b1 vs. b3, P<0.05), while antioxidant treatment attenuated this effect (b3 vs. b5, P<0.05) (Figure 1B).
Effects of various stimulations on the cell cycle
HG conditions elevated the proportion of G1 phase cells (shown as percent in parentheses) in a dose-dependent manner. Compared to the a2 (77.1±7.40) or a3 (82.3±6.20) groups, the a1 group (68.2±42.5) (control) differed significantly (P<0.05). However, no significant difference was detected between the proportion in high osmolality a4 (70.1±5.21) and a1 (control) (P>0.05). Without HG, pyruvate did not change the proportion of G1 phase significantly [a5 (72.2±6.22) vs. a1] (P>0.05). After adding the antioxidant, the proportion in the a6 group (72.2±5.32) was decreased compared to the a3 group (82.3±6.20) (P<0.05).
Moreover, the proportion of G1 phase cells was increased, and HG enhanced this effect. [comparing group b1 (75.2±5.35) with b6 (65.3±7.21), b3 (86.2±7.10) with b1, and b4 (80.2±8.12) with b2 (71.1±4.02), P<0.05]. However, after adding the antioxidants, the proportion was decreased when comparing the b5 group (72.2±4.22) to the b3 group (P<0.05).
Effects of various stimulations on p21Waf1 expression
The expression of p21Waf1 mRNA in group a3 was maximal. Following stimulation of HPMCs with HG and H2O2 (Figure 2A-2D), p21Waf1 mRNA expression was elevated compared with the control in a dose-dependent manner (a3 vs. a2, a2 vs. a1; b2 vs. b6, P<0.05). After adding the antioxidant in the HG- (a3 vs. a6) or H2O2-containing medium (b3 vs. b5), the expression was significantly reduced in both. Moreover, no statistically significant difference was detected in p21Waf1 gene expression among groups a4, a5, and control (Figure 2C). On the other hand, HG enhanced the effect of H2O2 (b4 vs. b2), as shown in Figure 2D (P<0.05).
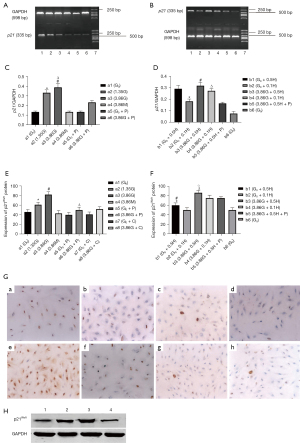
The expression of p21Waf1 protein in the HG group (a3) was maximal, followed by the a2, a6, a8, a1, a4, a5, and a7 groups (Figure 2E). As a response to HG stimulation, p21Waf1 protein expression was higher in HPMCs than in controls in a dose-dependent manner (a3 vs. a2, a2 vs. a1, P<0.05). This effect was reduced significantly by the antioxidant (a6 vs. a3, P<0.05). No difference was observed between p21 expression in the high osmolality (a4) group and the control group (Figure 2E). The expression of p21Waf1 protein in the HG group (b3) was the strongest, followed by the b4, b5, b1, b2, and b6 groups. We found that exogenous H2O2 elevated the expression of p21 (b1 vs. b6, b1 vs. b2, P<0.05), which was enhanced by HG (b3 vs. b1), and the antioxidant counteracted the effect of exogenous H2O2 (b3 vs. b5, P<0.05) (Figure 2F). The representative ICC images for p21 are shown in Figure 2G.
The results of Western blot were similar to that of ICC. The expression between the HG group (a3) and the HG plus H2O2 group (b3) did not differ significantly, and both groups had stronger expression than the control group (a1). The expression (a6) was reduced after the addition of antioxidants but remained higher than the control (P<0.05) (Figure 2H).
Effects of various stimulations on p27Kip1 expression
The representative results of RT-PCR for p27 are shown in Figure 3A,3B. Following stimulation of HPMCs with HG (Figure 3A) and H2O2 (Figure 3B), no significant differences were observed in p27 gene expression (Figure 3A,3B) (P>0.05).
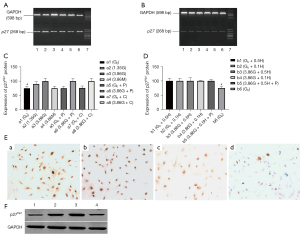
Following the stimulation of HPMCs with HG, the expression of p27Kip1 protein in the a3, a6, a8, and a2 groups was similar, with approximately 100% positive expression and no significant differences. Moreover, p27 expression in the a1, a4, a5, and a7 groups was also similar, approximately 75% positive, which was significantly different when compared to that of the a3, a6, a8, a2 groups (P<0.05). This indicated that HG increased p27 expression. However, a significant change was observed in p27 expression after pyruvate addition. Also, no difference was observed in p27 expression between the high osmolality group (a4) and the control group (Figure 3C). Both exogenous H2O2 and H2O2 plus glucose elevated the expression of p27. The positive cell percent of all 5 groups with HG or H2O2 was nearly 100% compared to that of the b6 group (75%), which reached statistical significance (Figure 3D). The representative ICC images for p27 are shown in Figure 3E. The results of Western blot were similar to p21Waf1 (Figures 2H,3F).
Discussion
In our study, we preliminarily demonstrated: (I) the effect of ROS and HG on the growth (proliferation) of HPMCs and the role of p21 and p27 in this process; (II) the role of ROS in HG-induced changes in cell growth in HPMCs.
Effects of HG and exogenous H2O2 on the growth and cell cycle of HPMCs
This study suggested that HG inhibits cell proliferation, as described previously (4). We also found that exogenous H2O2 inhibits the proliferation of HPMCs in a dose-dependent manner. The low concentration of H2O2 did not have an obvious effect, which is supported by similar study on cultured human tracheal epithelium and renal proximal tubule cells (3). On the other hand, we also observed that the addition of H2O2 in HG medium enhanced the cytotoxicity of H2O2. These results suggest that peritonitis or long-term dialysis might cause injury to CAPD patients from the increased generation of H2O2, which might be harmful in the presence of HG.
We speculated that these effects are related to the changes in HG- or exogenous H2O2-induced cell cycle. In this study, we found that both HG and exogenous H2O2 can induce cell cycle arrest of HPMCs at the G1 phase, but not with HG-induced high osmolality. This finding was supported by Zhang et al. (10) who demonstrated that H2O2 induced the premature senescence of human diploid fibroblasts by increasing p21 gene expression and arresting the cell cycle at the G1 phase. Therefore, based on the current results, we can deduce that HG inhibits the growth of HPMCs by arresting cell cycle progression.
Effects of HG and exogenous H2O2 on the expression of p21Waf1 and p27Kip1 in HPMCs
In diabetes research, p21 and p27 have been associated with the cytotoxicity of HG. We found that both HG and exogenous H2O2 increased the expression of p21Waf1, which was supported by Lin et al. (8) in a diabetic nephropathy study and Kim et al. (11) in human fibroblast cells. For p27Kip1, protein expression was increased by either HG or exogenous H2O2, but neither of them influenced the gene expression of p27Kip1. Elson et al. (13) demonstrated that in most situations, the gene expression of p27 is consistent in the cell cycle, indicating that the alteration of p27 occurs at the protein level, corresponding to our results. In a study on diabetic nephropathy, Aitken et al. (14) demonstrated that HG inhibits cell proliferation (cell growth) in a dose-dependent manner, which is associated with the increased expression of p27Kip1. Thus, we inferred that both HG and exogenous H2O2 can inhibit the growth of HPMCs by arresting cell cycle progression via increased expression of p21 and p27.
Effects of endogenous ROS on HG-induced growth inhibition and the expression of p21Waf1 and p27Kip1 in HPMCs
HG-induced growth inhibition and cell cycle arrest in HPMCs are attributed to a complex mechanism. Recently, the role of ROS in PD has been under intense focus. ROS are now being accepted as signaling molecules which activate protein kinases, transcription factors, and gene expression (15). Horiuchi et al. (16) found that cultured HPMCs can produce H2O2. Moreover, mesothelial cells in culture significantly increased the generation of H2O2 when exposed to HG. Recently, a study measured ROS generation by HPMCs cultured under HG using a ROS-sensitive fluorescence probe and confocal microscope (15). On the other hand, peritoneal infection or long-term, continuous stimulation by the biologically incompatible peritoneal dialysate is likely to produce ROS in the peritoneal cavity, which might harm HPMCs and CAPD patients.
In this study, HG-induced p21Waf1 expression was downregulated at both the gene and protein level after the addition of antioxidants to the HG media. Moreover, G1 phase arrest and inhibited cell growth were attenuated, indicating that the antioxidants (pyruvate and catalase) counteracted the effect of endogenous ROS. Shostak et al. reported that pyruvate could improve the peritoneal dialysate-induced inhibition of proliferation in HPMCs (17). These results were supported by other similar study (18). We also found a specific scavenger for H2O2, namely catalase, which can improve the inhibition of cell growth. This finding suggests endogenous H2O2 is a vital part of ROS, which is linked to HG-induced cell growth inhibition. Although a study has reported the ROS-increased expression of p27Kip1 and HG-increased expression of p27Kip1, the effect of endogenous ROS is not certain. At least, in our preliminary study, we could not demonstrate any significant influence of endogenous ROS on the expression of p27, as shown by ICC results in our previous study (19). Thus, we infer that in our methods, the incubation time of HG with HPMCs is limited, which might restrict the effect of ROS. Therefore, we could not detect the effect of endogenous H2O2 on p27 expression with ICC, which is different from CAPD in vivo. Also, the HPMCs were in contact with HG for a prolonged period. Western blotting presented a different positive result, indicating a certain effect of endogenous ROS on p27 expression. These discrepancies in the results may be due to the high sensitivity of Western blots compared to ICC in quantitative analysis. Thus, it may be inferred that HG-dependent cell damage is a function of both the reduced production of scavengers and the increased generation of ROS by mesothelial cells.
Interestingly, one study suggested that HG increases the expression of p21 and p27, mediated by TGF-β1 (20). These results indicate that chronic exposure to elevated glucose might result in TGF-β1-mediated accelerated senescence of HPMCs in vitro. Jin et al. demonstrated that the role of HG is related to ROS (21), but to the best of our knowledge, the role of ROS in p21 and p27 expression in HPMCs has not yet been studied. Our preliminary results suggested that HG inhibits cell growth by increasing ROS-mediated p21 and p27 expression.
Nevertheless, the present study has some limitations. We could not directly measure H2O2 concentration in HG medium. Furthermore, the significance of direct measurement is with little meaning since the effect of H2O2 could be influenced by other factors, such as cell types and the microenvironment. Our previous study found that high glucose increased the expression of monocyte chemoattractant protein-l (MCP-1) and interlukin-8 (IL-8), and endogenous ROS could induce the expression of IL-8 (22). Many researches have confirmed that the classical signaling pathway involved about p21Waf1 is p21WAF1/CIP1, and the classical signaling pathway involved in p27Kip1 is mitogen-activated extracellular signal-regulated kinase-extracellular regulated protein kinases (MEK-ERK) signaling (23,24). But the mechanisms of HG-induced changes in the cell cycle and cell proliferation in HPMCs have not yet been clarified, although ROS may play a role. Thus, the signal transduction pathways through which ROS affects the cell cycle and proliferation need to be investigated further.
Acknowledgments
We thank Prof. Yijie Wu, Department of Endocrinology, Shanghai General Hospital, for revising the English manuscript; Prof. Shanlin Liu, Department of Biochemistry, Shanghai Medical College, Fudan University, China, for his kind suggestions in this study; and Dr. Kaitlyn Reasoner for her kind help in revising and polishing the English writing of this article.
Funding: This study was supported by the National Natural Science Foundation of China (No. 81800676).
Footnote
Reporting Checklist: The authors have completed the MDAR reporting checklist. Available at https://atm.amegroups.com/article/view/10.21037/atm-22-4352/rc
Data Sharing Statement: Available at https://atm.amegroups.com/article/view/10.21037/atm-22-4352/dss
Conflicts of Interest: All authors have completed the ICMJE uniform disclosure form (available at https://atm.amegroups.com/article/view/10.21037/atm-22-4352/coif). The authors have no conflicts of interest to declare.
Ethical Statement: The authors are accountable for all aspects of the work in ensuring that questions related to the accuracy or integrity of any part of the work are appropriately investigated and resolved.
Open Access Statement: This is an Open Access article distributed in accordance with the Creative Commons Attribution-NonCommercial-NoDerivs 4.0 International License (CC BY-NC-ND 4.0), which permits the non-commercial replication and distribution of the article with the strict proviso that no changes or edits are made and the original work is properly cited (including links to both the formal publication through the relevant DOI and the license). See: https://creativecommons.org/licenses/by-nc-nd/4.0/.
References
- Zhu N, Gu L, Jia J, et al. Endothelin-1 triggers human peritoneal mesothelial cells' proliferation via ERK1/2-Ets-1 signaling pathway and contributes to endothelial cell angiogenesis. J Cell Biochem 2019;120:3539-46. [Crossref] [PubMed]
- Margetts PJ. Twist: a new player in the epithelial-mesenchymal transition of the peritoneal mesothelial cells. Nephrol Dial Transplant 2012;27:3978-81. [Crossref] [PubMed]
- Shen S, Ji C, Wei K. Cellular Senescence and Regulated Cell Death of Tubular Epithelial Cells in Diabetic Kidney Disease. Front Endocrinol (Lausanne) 2022;13:924299. [Crossref] [PubMed]
- Zhang Y, Xiao WH, Huang YX, et al. MiR-128-3p inhibits high-glucose-induced peritoneal mesothelial cells fibrosis via PAK2/SyK/TGF-β1 axis. Ther Apher Dial 2022; Epub ahead of print. [Crossref] [PubMed]
- Krediet RT. Acquired Decline in Ultrafiltration in Peritoneal Dialysis: The Role of Glucose. J Am Soc Nephrol 2021;32:2408-15. [Crossref] [PubMed]
- Zhu N, Wang L, Guo H, et al. Thalidomide Suppresses Angiogenesis Through the Signal Transducer and Activator of Transcription 3/SP4 Signaling Pathway in the Peritoneal Membrane. Front Physiol 2021;12:712147. [Crossref] [PubMed]
- Roumeliotis S, Dounousi E, Salmas M, et al. Unfavorable Effects of Peritoneal Dialysis Solutions on the Peritoneal Membrane: The Role of Oxidative Stress. Biomolecules 2020;10:768. [Crossref] [PubMed]
- Lin SH, Ho WT, Wang YT, et al. Histone methyltransferase Suv39h1 attenuates high glucose-induced fibronectin and p21(WAF1) in mesangial cells. Int J Biochem Cell Biol 2016;78:96-105. [Crossref] [PubMed]
- Chidawanyika T, Supattapone S. Hydrogen Peroxide-induced Cell Death in Mammalian Cells. J Cell Signal 2021;2:206-11. [PubMed]
- Zhang SL, Li BL, Li W, et al. Retracted: The Effects of Ludartin on Cell Proliferation, Cell Migration, Cell Cycle Arrest and Apoptosis Are Associated with Upregulation of p21WAF1 in Saos-2 Osteosarcoma Cells In Vitro. Med Sci Monit 2021;27:e931590. [Crossref] [PubMed]
- Kim W, Kwon HJ, Jung HY, et al. P27 Protects Neurons from Ischemic Damage by Suppressing Oxidative Stress and Increasing Autophagy in the Hippocampus. Int J Mol Sci 2020;21:9496. [Crossref] [PubMed]
- Zhang J, Buranjiang G, Mutalifu Z, et al. KIF14 affects cell cycle arrest and cell viability in cervical cancer by regulating the p27Kip1 pathway. World J Surg Oncol 2022;20:125. [Crossref] [PubMed]
- Elson DJ, Nguyen BD, Wood R, et al. The cyclin-dependent kinase inhibitor p27Kip1 interacts with the aryl hydrocarbon receptor and negatively regulates its transcriptional activity. FEBS Lett 2022;596:2056-71. [Crossref] [PubMed]
- Aitken TJ, Crabtree JE, Jensen DM, et al. Decreased proliferation of aged rat beta cells corresponds with enhanced expression of the cell cycle inhibitor p27KIP1. Biol Cell 2021;113:507-21. [Crossref] [PubMed]
- Jia M, Qiu H, Lin L, et al. Inhibition of PI3K/AKT/mTOR Signalling Pathway Activates Autophagy and Suppresses Peritoneal Fibrosis in the Process of Peritoneal Dialysis. Front Physiol 2022;13:778479. [Crossref] [PubMed]
- Horiuchi T, Matsunaga K, Banno M, et al. HPMCs induce greater intercellular delocalization of tight junction-associated proteins due to a higher susceptibility to H2O2 compared with HUVECs. Perit Dial Int 2009;29:217-26. [Crossref] [PubMed]
- Shostak A, Gotloib L, Kushnier R, et al. Protective effect of pyruvate upon cultured mesothelial cells exposed to 2 mM hydrogen peroxide. Nephron 2000;84:362-6. [Crossref] [PubMed]
- Zhang JJ, Shen HQ, Deng JT, et al. Effect of peritoneal dialysis solution with different pyruvate concentrations on intestinal injury. Exp Biol Med (Maywood) 2020;245:644-53. [Crossref] [PubMed]
- Tang ZH, Yao J, Liu J, et al. Reactive oxygen species and high glucose induce growth inhibition of human peritoneal mesothelial cells through arresting cell cycles progression. Chinese Journal of Nephrology 2003;19:223-7.
- Ksiazek K, Korybalska K, Jörres A, et al. Accelerated senescence of human peritoneal mesothelial cells exposed to high glucose: the role of TGF-beta1. Lab Invest 2007;87:345-56. [Crossref] [PubMed]
- Jin QH, Hu XJ, Zhao HY. Curcumin activates autophagy and attenuates high glucose-induced apoptosis in HUVECs through the ROS/NF-κB signaling pathway. Exp Ther Med 2022;24:596. [Crossref] [PubMed]
- Tang ZH, Zhang Z, Liu J, et al. Effect of high glucose on the expression of IL-8 and MCP-1 in human peritoneal mesothelial cells and the role of reactive oxygen species in this course. Chinese Journal of Blood Purification 2002;1:12-5.
- Cheng SY, Seo J, Huang BT, et al. Mitomycin C and decarbamoyl mitomycin C induce p53-independent p21WAF1/CIP1 activation. Int J Oncol 2016;49:1815-24. [Crossref] [PubMed]
- Bhatt KV, Spofford LS, Aram G, et al. Adhesion control of cyclin D1 and p27Kip1 levels is deregulated in melanoma cells through BRAF-MEK-ERK signaling. Oncogene 2005;24:3459-71. [Crossref] [PubMed]
(English Language Editor: C. Betlazar-Maseh)