Amentoflavone inhibits colorectal cancer epithelial-mesenchymal transition via the miR-16-5p/HMGA2/β-catenin pathway
Introduction
Colorectal cancer (CRC) is one of the most common malignant tumors in humans and is the main cause of cancer-related deaths worldwide (1). The Wnt/β-catenin signaling pathway plays a role in the invasion and metastasis of CRC (2-5). Epithelial to mesenchymal transition (EMT) is the key mechanism that drives most cancers, including CRC invasion and metastasis (6). Dysregulation of the Wnt/β-catenin signaling pathway has been shown to play an important role in the EMT required for colorectal tumor metastasis (7,8).
Amentoflavone is a natural biflavone compound with many biological properties, including anti-inflammatory, anti-oxidative, and anti-apoptotic effects (9,10). Previous studies have also found that amentoflavone exerts antitumor effects in solid tumors (11-13). However, the underlying mechanisms and functions of amentoflavone on CRC EMT have not been fully explored.
MicroRNAs (miRNAs) are a large class of small non-coding RNAs (14). The miR-16 family comprises six mature miRNAs (miR15a/b, miR-16-5p, miR-195, miR-424, and miR-497) widely explored in various cancers and considered tumor suppressors that inhibit tumor progression (15-20). MiR-16-5p has been reported to inhibit CRC progression (21). However, the potential role of miR-16-5p in amentoflavone-suppressed CRC tumor progression and its mediation of EMT in CRC remains unknown. In the present study, we demonstrated both in vitro and in vivo that amentoflavone increases miR-16-5p expression to inhibit CRC EMT and has an antitumor effect by blocking the HMGA2/Wnt/β-catenin pathway. We present the following article in accordance with the ARRIVE reporting checklist (available at https://atm.amegroups.com/article/view/10.21037/atm-22-3035/rc).
Methods
Tissue specimens
Ninety-six CRC tissues and paired adjacent normal tissues were obtained from patients diagnosed with CRC who received surgery at the First Affiliated Hospital of Guangxi University of Chinese Medicine. The study was conducted in accordance with the Declaration of Helsinki (as revised in 2013). The study was approved by the Medical Research Ethics Committee of the First Affiliated Hospital of Guangxi University of Chinese Medicine (No. UEXP00001325). Informed consent was taken from all the patients.
Cell culture and transient transfection
Human CRC cell lines (HCT116 and SW480) from the Chinese Type Culture Collection (Shanghai, China) were cultured in an Roswell Park Memorial Institute (RPMI) 1640 medium (RPMI 1640; Gibco, USA) supplemented with 10% fetal bovine serum (FBS; Gibco, USA) and 2% penicillin/streptomycin (HyClone, Shanghai, China). All cell lines were authenticated by short tandem repeat (STR) profiling and all experiments were performed with mycoplasma-free cells. The cells were treated with amentoflavone (50 µM) followed by Lipopolysaccharide (1 µg/mL). The miRNA inhibitors and the miRNA negative control (NC) were synthesized by the Shanghai Gene Pharma Co., Ltd. (Shanghai, China) and transiently transfected as previously described (22). The concentration of amentoflavone in the study was reference as previous (11-13).
Quantitative reverse transcription polymerase chain reaction (qRT-PCR)
TRIzol reagent (Invitrogen, Carlsbad, CA, USA) was used to extract total RNA from the CRC tissues and CRC cells. qRT-PCR was performed as previously described (22). The primers involved in this study were designed and provided by Sangon Biotech (Shanghai) Co. Ltd. The specific miRNA and mRNA expression levels were quantified using the 2−∆∆CT method. U6 and ACTIN genes were used as normalization controls.
Cell invasion and migration assays
A 24-well chamber with an 8-µm pore size (cat. no. 3422; Corning, Inc.) was used to detect the cell migratory and invasive abilities according to the manufacturer’s protocol and performed as described previously (22). Use hole diameter of 8 µM Transwell chamber (Corning Inc., Corning, NY, USA) to evaluate cell migration and invasion. 48 hours after transfection, 5× the transfected cells were put into 300 µL medium without FBS and placed in the upper chamber, and 500 µL medium with 20% FBS was placed in the lower chamber. For matrix invasion test, Transwell chamber is coated with matrix (BD Biosciences, San Jose, CA, USA). Inoculate 5 with 300 µL culture medium without FBS × 104 transfected cells were transferred to Transwell upper chamber, and 500 µL culture medium with 20% FBS was placed in the lower chamber. The cells were incubated at 37 ℃ for 24 hours for migration and 48 hours for invasion. In the two tests, the cells were fixed with 100% methanol for 5 min (Baotai Biotechnology Research Institute, Haimen, China), and then stained with 0.5% crystal violet (Baotai Biotechnology Research Institute) for 5 min. Subsequently, the cells remaining on the upper surface of the membrane were carefully removed with a cotton swab. Then, in five randomly selected fields of view, an inverted microscope (magnification, ×200) count migrating and invading cells. Each experiment was repeated 3 times.
Western blotting (WB)
Total protein from the CRC cell lines and tissues was isolated, and the protein concentrations were measured using a bicinchoninic acid (BCA) protein assay kit (Pierce, IL, USA). The follow-up experiments were performed as described previously (22). E-Cadherin as the epithelial marker, and N-Cadherin and Vimentin as the mesenchymal marker were selected. The primary antibodies are listed in Table S1.
Luciferase assay
The pmirGLO-HMGA2 3'-UTR (WT) or pmirGLO-mutant HMGA2 3'-UTR (MUT) were co-transfected into cells with miR-16-5p or miR-NC by Lipofectamine 2000. The follow-up experiments were performed as described previously (22).
TOP/FOP flash assay
A TOP/FOP flash assay was performed as previously described (23). Luciferase activities were measured using the Dual-Glo Luciferase Assay System (Promega, Madison, Wisconsin, USA).
The patient-derived xenograft (PDX) model
To evaluate the therapeutic effect and molecular mechanism of Amentoflavone against CRC, the murine CRC model was used in this study. The female C57BL/6 mice were purchased from Changsha Tianqin BioTech Ltd. (China). All the mice were housed and bred under SPF condition, a 12-hour light/12-hour dark cycle was set under the animal facility, temperature was set 18–23 ℃ with 40–60% humidity.
For the PDX model, primary tumor samples were obtained for xenograft establishment as described previously (24). The mice were given oral doses of amentoflavone (50 mg/kg body weight) or vehicle control daily for 45 days (n=5 for each group). In this study, roughly 120 mice were used. The animals were euthanized when the diameter of the tumor exceeded 200 mm, to reduce any unnecessary pain, suffering and distress. No unexpected adverse events had been observed throughout this study. In this study, the endpoint refers the end stage of experimental period, and/or the tumor exceeded 200 mm. The mice with a CRC tumor were randomized into different groups.
Animal experiments were performed under a project license (No. ANIEXP00003106) granted by the Ethical Committee of the First Affiliated Hospital of Guangxi University of Chinese Medicine, in compliance with national guidelines for the care and use of animals. A protocol was prepared before the study without registration.
Statistical analyses
To maintain the reliability of the conducted experiments, the performers were blinded, only the experiment designer(s) were aware of the group allocations. The data were analyzed using Prism 5 (GraphPad Software, San Diego, CA, USA) and SPSS 17.0 software (SPSS Inc., Cary, NC, USA). The values are presented as the means ± structural equation modeling (SEM). The details of the statistical methods are described in the Figure legends. All experiments were performed at least three times in duplicate.
Results
Amentoflavone inhibited CRC cell migration, invasion, and EMT
The Transwell assay indicated that compared with the control group, the migrative and invasive ability of HCT116 and SW480 cells treated with amentoflavone (50 µM) was significantly decreased (Figure 1A,1B). Therefore, migration and invasion of HCT116 and SW480 cells may be inhibited in response to amentoflavone treatment. The qRT-PCR and WB analyses indicated that the relative mRNA and protein expression of E-cadherin in HCT116 and SW480 treated with amentoflavone (50 µM) were significantly increased, while N-cadherin and vimentin were significantly decreased (Figure 1C,1D). The aforementioned results suggest that amentoflavone may inhibit CRC cell migration and invasion by EMT.
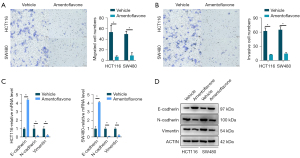
Amentoflavone increased miR-16-5p levels to inhibit EMT in CRC cells
The miR-16 family (miR-16-5p/miR-195-5p/miR-424-5p/miR-497-5p) exhibits a tumor suppressive potential, reducing EMT in many tumors. As shown in Figure 2A and Figure S1A, we found an increased level of miR-16-5p in HCT116 and SW480 cells treated with amentoflavone, while no changes were found in other family members. Additionally, we found that inhibited miR-16-5p enhanced EMT and reinstated the EMT ability in HCT116 and SW480 cells treated with amentoflavone (Figure 2B,2C and Figure S1B). Meanwhile, amentoflavone treatment inhibited the migration and invasion of HCT116 and SW480 cells, whereas inhibited miR-16-5p expression enhanced HCT116 and SW480 cell migration and invasion (Figure 2D,2E). Moreover, inhibited miR-16-5p reversed the migration and invasion ability in HCT116 and SW480 cells treated with amentoflavone (Figure 2D,2E). The above results indicated that amentoflavone inhibited EMT, migration, and invasion ability in CRC cells by increasing miR-16-5p levels.
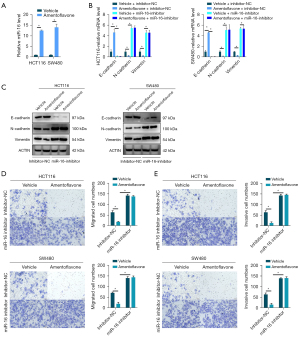
miR-16-5p targets HMGA2 in CRC
To explore the EMT function of miR-16-5p in CRC, we used the TargetScan database (http://www.targetscan.org/vert_71/) to identify the target genes of miR-16. Among the predicted targets, we focused on eight genes that had cumulative weighted context++ scores >0.50 and an aggregate PCT (percentage) >0.80 (Table S2). As shown in Figure 3A, HMGA2, reported to be involved in inducing EMT in CRC (25), was increased in HCT116 and SW480 cells treated with miR-16-5p inhibitor, while there was no change in the other 21 genes. Therefore, we selected HMGA2 for further validation in CRC. According to the prediction by the seed complementarity, a potential miR-16-5p-binding site was found at nt1302–1309 of HMGA2 3'-UTR (Figure 3B). Additionally, the results showed that co-transfection of miR-16-5p inhibitor significantly increased the firefly luciferase activity of the reporter with WT 3'-UTR of HMGA2 but not that of the mutant reporter (Figure 3C), which indicates that miR-16-5p directly targets the 3'-UTR of HMGA2.
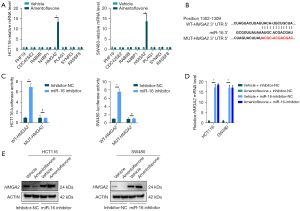
Amentoflavone decreased HMGA2 via miR-16-5p
As shown in Figure 3D,3E, amentoflavone treatment suppressed the mRNA and protein of HMGA2 in HCT116 and SW480 cells, whereas inhibited miR-16-5p expression increased the mRNA and protein of HMGA2 in HCT116 and SW480 cells. Moreover, inhibited miR-16-5p replenished the mRNA and protein of HMGA2 in HCT116 and SW480 cells treated with amentoflavone (Figure 3D,3E). All the above results indicated that amentoflavone decreased HMGA2 via miR-16.
Amentoflavone inactivated the Wnt/β-catenin pathway via the miR-16/HMGA2 axis
As HMGA2 is a key regulator activating the Wnt/β-catenin pathway and miR-16-5p targets HMGA2 in CRC, we wondered whether amentoflavone inactivates the Wnt/β-catenin pathway via miR-16. As shown in Figure 4A, amentoflavone treatment suppressed the protein level of β-catenin in HCT116 and SW480 cells, whereas inhibited miR-16-5p expression increased the protein level of β-catenin in HCT116 and SW480 cells. Moreover, inhibited miR-16-5p replenished the protein level of β-catenin in HCT116 and SW480 cells treated with amentoflavone (Figure 4B). Additionally, amentoflavone treatment suppressed the Wnt/TCF luciferase reporter activity in HCT116 and SW480 cells, whereas inhibited miR-16-5p expression increased the Wnt/TCF luciferase reporter activity in HCT116 and SW480 cells. Moreover, inhibited miR-16-5p replenished the Wnt/TCF luciferase reporter activity in HCT116 and SW480 cells treated with amentoflavone (Figure 4B). As shown in Figure 4C, amentoflavone treatment suppressed the mRNA expression of Wnt targets in HCT116 and SW480 cells, whereas inhibited miR-16-5p expression increased the mRNA expression of Wnt targets in HCT116 and SW480 cells. Moreover, inhibited miR-16-5p replenished the mRNA expression of Wnt targets in HCT116 and SW480 cells treated with amentoflavone (Figure 4C). Meanwhile, amentoflavone treatment suppressed the protein level of β-catenin in HCT116 and SW480 cells, whereas overexpression of HMGA2 expression increased the protein level of β-catenin in HCT116 and SW480 cells. Moreover, overexpression of HMGA2 expression replenished the protein level of β-catenin in HCT116 and SW480 cells treated with amentoflavone (Figure 4D). Additionally, amentoflavone treatment suppressed the Wnt/TCF luciferase reporter activity in HCT116 and SW480 cells, whereas overexpression of HMGB2 expression increased the Wnt/TCF luciferase reporter activity in HCT116 and SW480 cells. Moreover, overexpression of HMGA2 expression replenished the Wnt/TCF luciferase reporter activity in HCT116 and SW480 cells treated with amentoflavone (Figure 4E). As shown in Figure 4F, amentoflavone treatment suppressed the mRNA expression of Wnt targets in HCT116 and SW480 cells, whereas overexpression of HMGA2 expression increased the mRNA expression of Wnt targets in HCT116 and SW480 cells. Moreover, overexpression of HMGB2 expression replenished the mRNA expression of Wnt targets in HCT116 and SW480 cells treated with amentoflavone (Figure 4F). All the above results indicated that amentoflavone inactivated the Wnt/β-catenin pathway via the miR-16/HMGA2 axis.
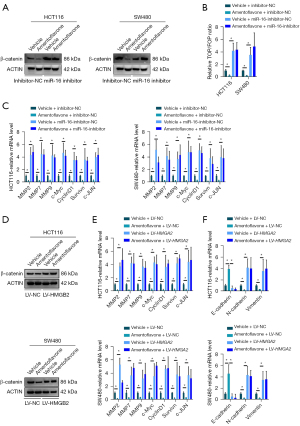
The combination of miR-16-5p and HMGA2 predicts prognosis in CRC patients
To assess the clinical significance of the miR-16-5p/HMGA2 axis in CRC, we examined the expression of miR-16 and HMGA2 mRNA in 96 CRC patients. As shown in Figure 5A,5B, miR-16-5p was decreased in CRC tumors compared to adjacent normal tissues, while HMGA2 mRNA was increased in CRC tumors compared to adjacent normal tissues. The expression of miR-16-5p was negatively correlated with the expression of HMGA2 mRNA in the 96 patients with CRC (Figure 5C and Table S3). Kaplan-Meier analysis revealed the survival benefits in CRC patients with low miR-16-5p or high HMGA2 mRNA levels (Figure 5D,5E). Of note, the combination of low miR-16-5p and high HMGA2 mRNA predicted a better overall survival of CRC patients (Figure 5F). Collectively, these results suggest the prognostic value of the combination of miR-16-5p and HMGA2 in predicting the outcome of CRC patients.
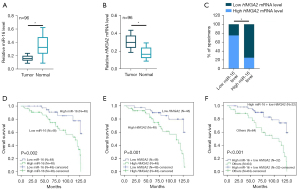
Amentoflavone exhibited antitumor effects in the PDX model
Next, by virtue of PDXs from CRC tumors, we found that, compared with the vehicle control, amentoflavone partially blocked the growth of PDXs derived from CRC tumors (Figure 6A,6B). Additionally, as shown in Figure 6C,6D, amentoflavone treatment suppressed the mRNA of HMGA2, whereas it increased miR-16-5p expression in PDXs. Meanwhile, amentoflavone treatment suppressed HMGA2 and β-catenin protein in the PDXs (Figure 6E,6F), which demonstrates that amentoflavone inhibited CRC tumor growth in vivo via the miR-16-5p/HMGA2/β-catenin pathway.
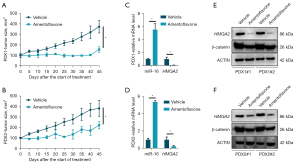
Discussion
In this study, we revealed that amentoflavone reduced the migration, invasion, and EMT in CRC cell lines by increasing miR-16-5p levels. Mechanistically, amentoflavone treatment inactivated the Wnt/β-catenin pathway via miR-16-5p, directly targeting 3'-UTR of HMGA2 to suppress HMGA2 expression in CRC. In addition, our clinical investigations revealed a close correlation between miR-16-5p and HMGA2 levels and CRC progression and patient prognosis. Moreover, the in vivo PDX model suggested that amentoflavone exhibited antitumor effects in vivo via the miR-16-5p/HMGA2/β-catenin pathway. Hence, these findings indicate that amentoflavone’s ability to inhibit migration, invasion, and EMT and exert antitumor effects might be attributable to the inhibition of the HMGA2/Wnt/β-catenin pathway activation by the increase in miR-16-5p expression (Figure 7).
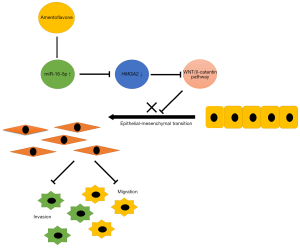
In our previous study, amentoflavone exhibited antitumor effects in several solid tumors (19-21). However, the anticancer effect and the mechanism of action of amentoflavone in CRC are unknown. In this study, we demonstrated the anti-CRC cell migration, invasion, and EMT effect of amentoflavone and the role of increased miR-16-5p expression on amentoflavone-induced inhibition of tumor progression in CRC. miR-16-5p is a member of the miR-15/-16/-195/-424/-497/-503 family. A remarkably inhibitory effect of miR-16-5p on the progression of several types of cancer has been reported previously (10-15). However, the function of miR-16-5p in the progression of CRC remains unknown. In this study, we found that amentoflavone reduced migration, invasion, and EMT in CRC cell lines dependent on an increased miR-16-5p expression, suggesting that miR-16-5p is a crucial tumor suppressor in CRC. In addition, our clinical investigations revealed a close correlation between miR-16-5p levels and CRC patient prognosis, thus making miR-16-5p an optimal target for CRC therapy.
MicroRNAs can function as tumor suppressors or oncogenes by targeting their respectively associated genes (23). Using TargetScan bioinformatics, the HMGA2 gene was potentially identified as a direct target for miR-16-5p. HMGA2 is a nonhistone and architectural transcription factor, which exerts an oncogenic effect on numerous cancers. Recently, overexpression of HMGA2 in CRC patients has been shown to play an important role in metastasis development and is a potential indicator of poor survivability (26-29). In this study, we demonstrated a negative regulation of HMGA2 by miR-16-5p using a luciferase reporter assay. Following amentoflavone treatment, HMGA2 RNA and protein levels decreased and were recovered by inhibiting miR-16-5p expression. In addition, we observed that miR-95-3p expression was negatively correlated with HMGA2 transcription in CRC. This finding implies that the miR-16-5p/HMGA2 axis might serve as a novel therapeutic target in patients with CRC.
There are multiple reports of Wnt/β-catenin pathway activation by HMGA2 to enhance migration, invasion, and EMT (30-32). In this study, we found that amentoflavone inhibited the Wnt/β-catenin pathway, and inhibiting miR-16-5p could reverse this inhibitory effect. Wnt/β-catenin signaling has been confirmed to promote tumor metastasis by inducing EMT (33). EMT is a process in which cancer cells lose their epithelial characteristics, obtain mesenchymal phenotypes, and develop motility and invasiveness (34). In the current study, we found that amentoflavone played an antitumor role in CRC by increasing miR-16-5p expression and suppressing HMGA2 and β-catenin levels, which suggests that the miR-16-5p/HMGA2/β-catenin axis determines the amentoflavone response.
There are some limitations associated with the current study. Future studies need to establish a metastatic model, such as a tail vein injection model, to validate the effects of amentoflavone on CRC cell metastasis in vivo. In this study, only the TargetScan database was explored to identify miR-16 target genes. Therefore, future studies should use additional online databases to define the potential targets of miR-16 in CRC cells. Our PDX model showed amentoflavone partially blocked the growth of PDXs derived from CRC tumors, but the in vitro data failed to show that amentoflavone inhibited the proliferation of CRC cells. Further research should use more PDX models to define the effect of amentoflavone in inhibiting CRC cell growth; to explore the novel lncRNA regulating the miR-16/HMGA2/β-catenin pathway in CRC; to explore the effect of amentoflavone on T cell infiltration and tumor microenvironment during EMT in CRC; supplemented results of morphological experiments in vivo experiments. Till now, the achievements from this study are still in preclinical stage, though it’s still need to accomplish comprehensive studies to evaluate its therapeutic effect on patients, it shed lights on discovery of new biomarkers for clinical purposes.
Taken together, our results demonstrated that amentoflavone has the potential to inhibit CRC progression through suppression of HMGA2/Wnt/β-catenin activation by increasing miR-16-5p levels.
Acknowledgments
The authors appreciate the academic support from the AME Colorectal Cancer Collaborative Group.
Funding: This research was funded by grants from the Guangxi Natural Science Foundation (No. 2020GXNSFAA297141) and the Self-Financing Project of the Guangxi Healthy Department (No. z20201201).
Footnote
Reporting Checklist: The authors have completed the ARRIVE reporting checklist. Available at https://atm.amegroups.com/article/view/10.21037/atm-22-3035/rc
Data Sharing Statement: Available at https://atm.amegroups.com/article/view/10.21037/atm-22-3035/dss
Conflicts of Interest: All authors have completed the ICMJE uniform disclosure form (available at https://atm.amegroups.com/article/view/10.21037/atm-22-3035/coif). The authors have no conflicts of interest to declare.
Ethical Statement: The authors are accountable for all aspects of the work in ensuring that questions related to the accuracy or integrity of any part of the work are appropriately investigated and resolved. The study was conducted in accordance with the Declaration of Helsinki (as revised in 2013). The study was approved by the Medical Research Ethics Committee of the First Affiliated Hospital of Guangxi University of Chinese Medicine (No. UEXP00001325). Informed consent was taken from all the patients. Animal experiments were performed under a project license (No. ANIEXP00003106) granted by the Ethical Committee of the First Affiliated Hospital of Guangxi University of Chinese Medicine, in compliance with national guidelines for the care and use of animals. A protocol was prepared before the study without registration.
Open Access Statement: This is an Open Access article distributed in accordance with the Creative Commons Attribution-NonCommercial-NoDerivs 4.0 International License (CC BY-NC-ND 4.0), which permits the non-commercial replication and distribution of the article with the strict proviso that no changes or edits are made and the original work is properly cited (including links to both the formal publication through the relevant DOI and the license). See: https://creativecommons.org/licenses/by-nc-nd/4.0/.
References
- Siegel RL, Miller KD, Jemal A. Cancer statistics, 2020. CA Cancer J Clin 2020;70:7-30. [Crossref] [PubMed]
- Qi J, Yu Y, Akilli Öztürk Ö, et al. New Wnt/β-catenin target genes promote experimental metastasis and migration of colorectal cancer cells through different signals. Gut 2016;65:1690-701. [Crossref] [PubMed]
- Zheng K, Zhou X, Yu J, et al. Epigenetic silencing of miR-490-3p promotes development of an aggressive colorectal cancer phenotype through activation of the Wnt/β-catenin signaling pathway. Cancer Lett 2016;376:178-87. [Crossref] [PubMed]
- Liu N, Jiang F, Han XY, et al. MiRNA-155 promotes the invasion of colorectal cancer SW-480 cells through regulating the Wnt/β-catenin. Eur Rev Med Pharmacol Sci 2018;22:101-9. [PubMed]
- Li J, He M, Xu W, et al. LINC01354 interacting with hnRNP-D contributes to the proliferation and metastasis in colorectal cancer through activating Wnt/β-catenin signaling pathway. J Exp Clin Cancer Res 2019;38:161. [Crossref] [PubMed]
- Hu JL, Wang W, Lan XL, et al. CAFs secreted exosomes promote metastasis and chemotherapy resistance by enhancing cell stemness and epithelial-mesenchymal transition in colorectal cancer. Mol Cancer 2019;18:91. [Crossref] [PubMed]
- Yang S, Liu Y, Li MY, et al. FOXP3 promotes tumor growth and metastasis by activating Wnt/β-catenin signaling pathway and EMT in non-small cell lung cancer. Mol Cancer 2017;16:124. [Crossref] [PubMed]
- Gu Y, Wang Q, Guo K, et al. TUSC3 promotes colorectal cancer progression and epithelial-mesenchymal transition (EMT) through WNT/β-catenin and MAPK signalling. J Pathol 2016;239:60-71. [Crossref] [PubMed]
- Oh J, Rho HS, Yang Y, et al. Extracellular signal-regulated kinase is a direct target of the anti-inflammatory compound amentoflavone derived from Torreya nucifera. Mediators Inflamm 2013;2013:761506. [Crossref] [PubMed]
- Park HJ, Kim MM. Amentoflavone Induces Autophagy and Modulates p53. Cell J 2019;21:27-34. [PubMed]
- Chiang CH, Yeh CY, Chung JG, et al. Amentoflavone Induces Apoptosis and Reduces Expression of Anti-apoptotic and Metastasis-associated Proteins in Bladder Cancer. Anticancer Res 2019;39:3641-9. [Crossref] [PubMed]
- Liu H, Yue Q, He S. Amentoflavone suppresses tumor growth in ovarian cancer by modulating Skp2. Life Sci 2017;189:96-105. [Crossref] [PubMed]
- Shen F, Chen Y, Chen L, et al. Amentoflavone Promotes Apoptosis in Non-Small-Cell Lung Cancer by Modulating Cancerous Inhibitor of PP2A. Anat Rec (Hoboken) 2019;302:2201-10. [Crossref] [PubMed]
- Xu TL, Sun YW, Feng XY, et al. Development of miRNA-Based Approaches to Explore the Interruption of Mosquito-Borne Disease Transmission. Front Cell Infect Microbiol 2021;11:665444. [Crossref] [PubMed]
- Cimmino A, Calin GA, Fabbri M, et al. miR-15 and miR-16 induce apoptosis by targeting BCL2. Proc Natl Acad Sci U S A 2005;102:13944-9. [Crossref] [PubMed]
- Chava S, Reynolds CP, Pathania AS, et al. miR-15a-5p, miR-15b-5p, and miR-16-5p inhibit tumor progression by directly targeting MYCN in neuroblastoma. Mol Oncol 2020;14:180-96. [Crossref] [PubMed]
- Jia X, Li X, Shen Y, et al. MiR-16 regulates mouse peritoneal macrophage polarization and affects T-cell activation. J Cell Mol Med 2016;20:1898-907. [Crossref] [PubMed]
- Yu T, You X, Zhou H, et al. MiR-16-5p regulates postmenopausal osteoporosis by directly targeting VEGFA. Aging (Albany NY) 2020;12:9500-14. [Crossref] [PubMed]
- Liu GP, Wang WW, Lu WY, et al. The mechanism of miR-16-5p protection on LPS-induced A549 cell injury by targeting CXCR3. Artif Cells Nanomed Biotechnol 2019;47:1200-6. [Crossref] [PubMed]
- Zhang H, Yang K, Ren T, et al. miR-16-5p inhibits chordoma cell proliferation, invasion and metastasis by targeting Smad3. Cell Death Dis 2018;9:680. [Crossref] [PubMed]
- Xu Y, Shen L, Li F, et al. microRNA-16-5p-containing exosomes derived from bone marrow-derived mesenchymal stem cells inhibit proliferation, migration, and invasion, while promoting apoptosis of colorectal cancer cells by downregulating ITGA2. J Cell Physiol 2019;234:21380-94. [Crossref] [PubMed]
- Yan D, He Q, Chen Y, et al. Detection of α-fetoprotein and glypican-3 mRNAs in the peripheral blood of hepatocellular carcinoma patients by using multiple FQ-RT-PCR. J Clin Lab Anal 2011;25:113-7. [Crossref] [PubMed]
- Konishi H, Sato H, Takahashi K, et al. Tumor-Progressive Mechanisms Mediating miRNA-Protein Interaction. Int J Mol Sci 2021;22:12303. [Crossref] [PubMed]
- Fang DD, Zhang B, Gu Q, et al. HIP1-ALK, a novel ALK fusion variant that responds to crizotinib. J Thorac Oncol 2014;9:285-94. [Crossref] [PubMed]
- Mansoori B, Mohammadi A, Naghizadeh S, et al. miR-330 suppresses EMT and induces apoptosis by downregulating HMGA2 in human colorectal cancer. J Cell Physiol 2020;235:920-31. [Crossref] [PubMed]
- Gao X, Dai M, Li Q, et al. HMGA2 regulates lung cancer proliferation and metastasis. Thorac Cancer 2017;8:501-10. [Crossref] [PubMed]
- Chen RX, Chen X, Xia LP, et al. N6-methyladenosine modification of circNSUN2 facilitates cytoplasmic export and stabilizes HMGA2 to promote colorectal liver metastasis. Nat Commun 2019;10:4695. [Crossref] [PubMed]
- Dong J, Wang R, Ren G, et al. HMGA2-FOXL2 Axis Regulates Metastases and Epithelial-to-Mesenchymal Transition of Chemoresistant Gastric Cancer. Clin Cancer Res 2017;23:3461-73. [Crossref] [PubMed]
- Zhang S, Mo Q, Wang X. Oncological role of HMGA2 Int J Oncol 2019;55:775-88. (Review). [PubMed]
- Jiang H, Li Y, Li J, et al. Long noncoding RNA LSINCT5 promotes endometrial carcinoma cell proliferation, cycle, and invasion by promoting the Wnt/β-catenin signaling pathway via HMGA2. Ther Adv Med Oncol 2019;11:1758835919874649. [Crossref] [PubMed]
- Yang S, Gu Y, Wang G, et al. HMGA2 regulates acute myeloid leukemia progression and sensitivity to daunorubicin via Wnt/β-catenin signaling. Int J Mol Med 2019;44:427-36. [Crossref] [PubMed]
- Li W, Wang J, Zhang D, et al. MicroRNA-98 targets HMGA2 to inhibit the development of retinoblastoma through mediating Wnt/β-catenin pathway. Cancer Biomark 2019;25:79-88. [Crossref] [PubMed]
- Teeuwssen M, Fodde R. Wnt Signaling in Ovarian Cancer Stemness, EMT, and Therapy Resistance. J Clin Med 2019;8:1658. [Crossref] [PubMed]
- Basu S, Cheriyamundath S, Ben-Ze'ev A. Cell-cell adhesion: linking Wnt/β-catenin signaling with partial EMT and stemness traits in tumorigenesis. F1000Res 2018;7:eF1000 Faculty Rev-1488.
(English Language Editor: D. Fitzgerald)