The role of ferritin and iron dextran in exacerbating preeclampsia in an L-NAME-treated rat model
Introduction
Preeclampsia (PE) is a pregnancy-specific disease occurring in the second half of gestation and is characterized by the onset of high blood pressure, proteinuria, and edema (1). This syndrome causes pregnancy complications in over 5% of pregnant women and is a leading cause of maternal and fetal morbidity and mortality worldwide (2). Previous studies have revealed that many factors contribute to the onset of PE, including disordered neovascularization, impaired spiral artery remodeling, and oxygen dysregulation (3-5). Placental hypoxia initiates the release of inflammatory cytokines and overproduction of reactive oxygen species (ROS), resulting in a maternal inflammatory response and multi-organ injuries (6). Although oxidative stress is regarded as one of the leading causes of atherosclerosis in PE, and the levels of lipid peroxides in the placenta were found to be altered in PE, the mechanism by which oxidative stress works in PE development has not been completely defined (7,8).
Iron is an essential element for living cells and most organisms, including humans (9). Iron participates in a mass of intracellular vital enzymatic redox reactions due to its tendency to reversibly obtain or lose electrons by transitioning between different oxidation states (10). It is widely accepted that pregnant women need more iron to support fetal growth and development (11). However, excessive iron intake or a high iron status may be harmful in pregnancy and lead to adverse pregnancy outcomes (APOs), such as preeclampsia, hypertension, placental abruption, and preterm delivery (12,13). On the other hand, ferroptosis is a form of programmed cell death that is different from apoptosis, autophagy, and pyroptosis. In essence, ferroptosis is lipid peroxidation damage induced by oxidative stress, and it accelerates the generation of ROS (14,15). Thus, increased ferritin concentrations and ferroptosis may be crucial mechanisms involved in the pathogenesis of PE.
In the current study, we aimed to explore the effects of ferritin concentrations and ferroptosis levels on the different stages of nitro-L-arginine methyl ester (L-NAME)-treated normotensive pregnant rats. We present the following article in accordance with the ARRIVE reporting checklist (available at https://atm.amegroups.com/article/view/10.21037/atm-22-3675/rc).
Methods
Animal experiments
The animal studies were approved under a project license (No. 21432) by the Ethics Committee of Animal Experiments of the School of Medicine, Zhejiang University and conducted in accordance with national guidelines for the care and use of animals. A total of 60 three-month-old female Wistar rats were purchased from the Nanjing Qinglongshan Animal Breeding Center (Nanjing, China) and kept under standard conditions that included a room temperature of 25±2 ℃, 12 h light/dark cycles, and free access to food pellets and water. The rats were mated with their respective male strain, and the first day of pregnancy was recorded by observing spermatozoa after a vaginal smear (16). Thirty rats were randomly divided into five groups (n=6): control group (con), deferoxamine (DFO)-20 group, DFO-100 group, iron dextran (ID)-20 group, and ID-100 group. The female rats were injected intraperitoneally with 20/100 mg/kg DFO or 20/100 mg/kg ID on days 5–9 of pregnancy (17,18), and 0.9% NaCl was used for the control group during the same 5 days. On day 10 of pregnancy, the rats were anesthetized and sacrificed by cervical dislocation. The placenta, vascular tissues, and blood were collected for further experiments.
Another thirty rats were randomly divided into six groups (n=5): control group (con), model group, DFO-early group, DFO-late group, ID-early group, and ID-late group. To establish a PE rat model, 25 pregnant rats were treated with L-NAME (60 mg/kg/day, orally) from days 13–19 of pregnancy. Among the PE model rats, 10 rats (n=5) were treated with an injection of 100 mg/kg DFO-early or 100 mg/kg ID-early from days 5–9 of pregnancy, respectively. 10 rats (n=5) were treated with 100 mg/kg DFO-late or 100 mg/kg ID-late from days 15–19 of pregnancy. No signs of peritonitis, pain, or discomfort were observed after anesthesia.
Hematoxylin and eosin (H&E) staining
The placenta and vascular tissues were observed after DFO or ID treatments in the presence and absence of L-NAME treatment by H&E staining. Briefly, these tissues were fixed in 10% neutral formaldehyde for 72 h, embedded in paraffin, cut into 4-µm thickness slices, and then stained with H&E staining. The pathological changes were observed under a light microscope (Olympus, Tokyo, Japan).
The measurement of reactive oxygen species (ROS), malondialdehyde (MDA), and superoxide dismutase (SOD) activity
ROS, MDA, and SOD activities were assessed in the placental homogenates with or without L-NAME administration. The corresponding available kits were used to detect ROS, MDA, and SOD levels according to the manufacturer’s standard procedures. Each experiment was repeated at least three times.
Detection of nitric oxide (NO) and nitric oxide synthase (NOS) levels
The NO and NOS levels in the vascular tissues of each group were evaluated by commercially available kits (Jiancheng, Nanjing, China) in accordance with the manufacturer’s instructions. Each experiment was carried out at least three times.
Enzyme-linked immunosorbent assay (ELISA)
Cytokine concentrations of IL-6, IL-1β, and TNF-α in placental tissues and plasma with or without L-NAME treatment were detected by ELISA kits (Jiancheng, Nanjing, China). The levels of ferritin (FER) and transferrin (TF) were measured with rat ELISA kits (Boster, Wuhan, China) as per the manufacturer’s instructions.
Blood pressure measurement
Systolic blood pressure (SBP) and mean arterial blood pressure (MAP) were taken in conscious rats using a tail-cuff machine (Letica 5000 devices, Comella, Spain) on the 19th day of pregnancy. Systolic blood pressure was measured consecutively three times and averaged.
Urine protein measurement
On the 19th day of pregnancy, 24-h urine in each group was collected, and total urine protein was determined by a sulfosalicylic acid test.
Western blot assay
The lysis of placental homogenates from rats with different treatment was implemented utilizing cold RIPA buffer (Bingtai Biotechnology Co., Ltd.). The isolated proteins were quantified with the help of a BCA Protein Assay Kit (Shanghai Zeye Biotechnology Co., Ltd.). After the exposure with 10% SDS-PAGE, the transferring of cell lysates to PVDF membranes, which were impeded with 5% nonfat milk, was operated. Subsequently, the overnight subjection of membranes with primary antibodies targeting Bax (1:2,000; ab182733), Bcl-2 (1:1,000; ab194583), caspase 3 (1:500; ab13847), cleaved caspase 3 (1:500; ab2302), GPx4 (1:1,000; ab231174), SLC7A11 (1:500; ab175186), ACSL4 (1:10,000; ab155282), TFR-1 (1:1,000; 10084-2-AP, Proteintech Group), and GAPDH (1:3,000; ab125247) was implemented at 4 ℃, after which was the 1 h of exposure to HRP-labeled secondary antibody (1:1,000; ab6734) for 1 h. The bands got visualized employing an enhanced chemiluminescent (ECL) detection kit and quantified by Image J software (NIH, USA). GAPDH was chosen as the internal control.
Statistical analysis
SPSS 21.0 software (SPSS Inc., Armonk, NY, USA) was used to analyze the data. Two-tailed Student’s t-tests and one-way analysis of variance (ANOVA) followed by Scheffe’s post-hoc test were used for the comparisons between two groups and multiple groups, respectively. Data are expressed as means ± standard deviation (SD). P<0.05 was considered to be statistically significant.
Results
Effects of different ferritin concentrations on histopathological changes and oxidative stress in the placental tissues of pregnant rats
The rats (n=6) were treated with either 20/100 mg/kg DFO or 20/100 mg/kg ID during days 5–9 of pregnancy. The placental tissues were harvested on the 10th day of pregnancy and stained with H&E reagent. As shown in Figure 1A, obvious inflammatory cell infiltration, increased vacuolated cells, cell hyperplasia, and edema were observed in the ID-20 and ID-100 groups compared with the two DFO groups and the control group. However, the density of vascular tissue in the placentas of pregnant rats decreased after treatment with DFO. Given that ferroptosis is implicated in the excessive lipid peroxidation mediated by Fe (II), the levels of ROS, MDA, and SOD activity were tested. According to Figure 1B, we found that ROS and MDA concentrations were markedly decreased in the DFO-treated groups but were increased in the ID-treated groups. However, SOD activity was elevated after treatment with DFO but reduced after ID administration.
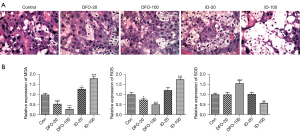
Ferritin content affected the ferroptosis-related process in pregnant rats
Next, we examined the effects of ferritin concentration on ferroptosis in pregnant rats. As depicted in Figure 2A,2B, the levels of inflammatory factors IL-6, IL-1β, and TNF-α were remarkably lower in the DFO-100 group, as were the levels of ferritin and transferrin (TF). However, ID treatment resulted in the opposite effect, with increased inflammatory, ferritin, and transferrin levels. Furthermore, western blot analysis showed that 100 mg/kg DFO significantly reduced Bax levels and increased Bcl-2 levels, while 100 mg/kg ID increased the levels of Bax, cleaved caspase 3, and inhibited Bcl-2 levels compared with the control group (Figure 2C). Additionally, ferroptosis-related proteins were assessed in the placental homogenate of pregnant rats. The results revealed that 100 mg/kg DFO notably increased the contents of GPX4 and SLC7A11, whereas 100 mg/kg ID inhibited the levels of the two proteins and elevated the production of ACSL4 and TFR-1 in pregnant rats (Figure 2D).
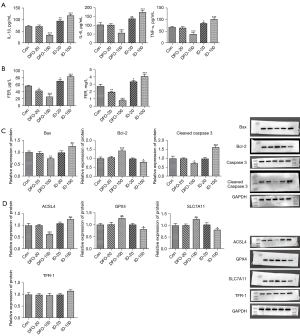
SBP, MAP, and proteinuria levels
From days 13 to 19 of gestation, pregnant rats were orally given 60 mg/kg/day of L-NAME. Blood pressure and urine protein levels were measured on day 19 of pregnancy. As shown in Figure 3A, SBP and MAP were significantly higher after L-NAME administration compared with the control group. Moreover, administration of 100 mg/kg of DFO in the later stage of pregnancy caused a decrease in both SBP and MAP, whereas 100 mg/kg of ID exacerbated high blood pressure in the same period. As shown in Figure 3B, the 24-h urinary protein of PE rats on the 19th day was dramatically higher than that of the control group. In the late period, the level of urine protein was reduced in the 100 mg/kg DFO group and increased in the ID group.
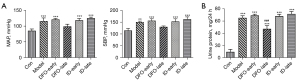
Effects of ferritin on blood vessels in pregnant rats with PE
To evaluate the influence of ferritin concentrations on the placental tissues of PE pregnant rats (n=5), their vascular tissue pathophysiology was assessed. As shown in Figure 4A, significant inflammatory cell infiltration and damage were observed in the placental blood vessels of the model rats. DFO treatment in the later stage of pregnancy ameliorated the pathological changes caused by L-NAME, whereas the ID-late group showed more serious histopathological lesions. Furthermore, in the model groups, the levels of NO and NOS were significantly suppressed compared with the control group. Treatment in the DFO-late group improved the lower levels of NO and NOS, but ID treatment in the later stage of pregnancy exacerbated the reduction of NO and NOS (Figure 4B). In addition, L-NAME treatment significantly increased the production of IL-6, IL-1β, and TNF-α in the peripheral blood of pregnant rats with PE. DFO treatment in the later stage of pregnancy ameliorated the excess secretions of IL-6, IL-1β, and TNF-α. In contrast, injection with ID in the second half of pregnancy aggravated the generation of these inflammatory cytokines compared with the model group (Figure 4C).
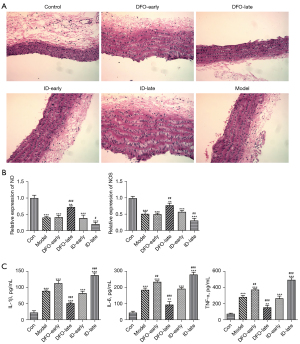
Low or high iron status altered the morphology and oxidation resistance of the placenta in PE rats
As depicted in Figure 5A, in the placenta of rats treated with L-NAME, there was an increase in vacuolated cells, thickening of the basal zone, increased inflammatory cell infiltration, and significant swelling and thickening of the meconium. The treatment with DFO in later pregnancy significantly protected placental tissues from inflammatory injuries. However, ID treatment in later pregnancy caused more inflammatory damage in the placenta of PE rats. Additionally, treatment with L-NAME for a lengthy period reduced the antioxidant ability of the placenta due to the increased levels of ROS and MDA. ID treatment in later pregnancy further diminished SOD activity and increased the levels of ROS and MDA, while DFO showed the opposite effects on antioxidant ability (Figure 5B-5D).
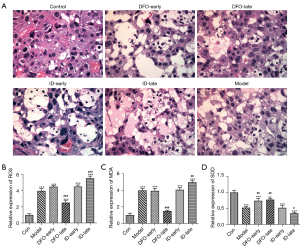
The effects of ferritin concentration on inflammation, apoptosis, and ferroptosis in the PE rat model
An ELISA assay and western blot analysis were performed to assess the effects of ferritin concentration on ferroptosis in the placentas of PE pregnant rats. As illustrated in Figure 6A, IL-6, IL-1β, and TNF-α were enhanced in the model group, showing increased ferritin and TF levels. However, DFO or ID administration during the later stage of pregnancy respectively inhibited or stimulated the secretions of IL-6, IL-1β, TNF-α, ferritin, and TF (Figure 6A,6B). Furthermore, the western blot assay results showed that DFO treatment in later pregnancy significantly reduced the protein levels of Bax and cleaved caspase 3 but increased Bcl-2 levels compared with the model group (Figure 6C). In addition, DFO treatment in later pregnancy noticeably promoted the levels of GPX4 and SLC7A11, whereas ID suppressed the levels of ACSL4 and TFR-1 compared with the model group (Figure 6D).
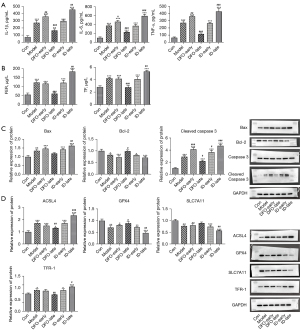
Discussion
Ferroptosis is caused by an accumulation of iron-dependent lipid peroxides and has achieved much attention due to its relationship with oxidative stress in many types of cells (19). In the course of pregnancy, iron was in great need to backing the growth as well as the advancement of the fetus (20). Nevertheless, it was evidenced that excessive iron intake and/or high iron status might imparted detrimental impacts on pregnancy, possibly contributing to reproductive disorders, like endometriosis and preeclampsia (21,22). However, dysregulated iron homeostasis, which was a contributor to excessive ferroptosis, may result in various clinical manifestations of reproductive disorders, together with pregnancy complications (11,23). Here, the functional impacts of ferritin concentrations and ferroptosis levels at different stages of pregnancy was appraised using a PE rat model. The data showed that different concentrations of ferritin in the earlier or later stages of pregnancy cause various biological changes in the placenta and vascular tissues in PE rats and, therefore, different degrees of ferroptosis, indicating that iron intervention at the early stage of pregnancy may exacerbate the degree of PE at late stage of pregnancy which provides a novel fundamental insight into iron intervention for the further treatment and prognosis of preeclampsia.
DFO is an iron chelator that reduces iron levels in plasma or specific organ tissues in experimental animals and humans (24-26). In this study, we administered 20 or 100 mg/kg DFO to decrease the ferritin levels in pregnant rats. Additionally, we increased the iron levels in other pregnant rats by injecting them with 20 or 100 mg/kg of ID (27). On the 10th day of pregnancy, all rats were sacrificed, and placental tissues were collected from those with intact placentas. The H&E staining results showed little inflammatory damage in untreated or DFO-treated rats. However, 5 days of ID treatment in pregnant rats led to severe pathological changes in placental tissues, suggesting that iron overload may be harmful to placental tissues in pregnancy (11).
Previous study has showed that ferritin was moderately high in the early weeks of pregnancy, but ferritin and other iron parameters were higher in women who suffered from APOs (28). Moreover, in the middle and later stages of pregnancy, ferritin levels were also significantly higher than in the normal population (29,30). ROS, MDA, and SOD are detection indexes that evaluate the levels of oxidative stress and lipid peroxidation, and their levels help determine the degree of ferroptosis in the placenta of PE women. D’Souza et al. revealed that MDA concentrations were significantly higher in the maternal cord blood and peripheral blood of women who developed PE (31). In our study, L-NAME was used to successfully establish a preeclampsia rat model, as demonstrated by our observations of high blood pressure and high concentrations of urinary protein. We found that the anti-oxidative activity of SOD was significantly diminished after treatment with L-NAME, but ROS and MDA levels were increased. Administration of DFO or ID had no obvious effects on the levels of ROS, MDA, and SOD in early pregnancy, while DFO and ID had opposite effects on the three indices in the later stage of pregnancy. Similarly, Amaral et al. reported that SOD activity was lower and MDA was significantly higher in rats treated with L-NAME (32).
Pregnancy hypertension and preeclampsia have a significant positive correlation with oxidative stress in the placenta (33). However, to date, there has been little evidence for an association between ferroptosis and PE. Considering ferroptosis is a crucial part of lipid peroxidation, we speculated that an increase in ferritin concentration and ferroptosis-related mechanisms might be associated with PE, and the alteration of ferritin concentrations at different stages of pregnancy might cause different influences on PE placental tissues. Previous studies have revealed that iron, ferritin, and transferrin were markedly elevated in patients with PE (34,35). Furthermore, a recent study showed that MDA and total Fe2+ levels in placental PE tissues were higher than those in healthy controls (36). In the present study, we also detected correlations between ferritin, iron parameters, and PE. The ELISA and western blot assay results demonstrated that ferritin and transferrin concentrations were significantly elevated in the L-NAME-treated placentas of PE rats compared with the untreated control group. Interestingly, in later pregnancy, the levels of ferritin and transferrin in PE were reduced by DFO treatment but increased with ID treatment.
GPx4 is a crucial antioxidant enzyme that inhibits the production of ROS-induced lipid hydroperoxides (37). Glutathione (GSH) is a substrate involved in the protective effect of GPx4. The synthesis of GSH depends on the facilitation of SLC7A11. Inhibition of SLC7A11 suppresses the production of GSH and sequentially promotes the accumulation of lipid hydroperoxides (38,39). Therefore, GPx4 and SLC7A11 are usually used as important markers for ferroptosis. Additionally, ACSL4 and TFR-1 are considered indicators of ferroptosis and are used to regulate lipid composition and the import and storage functions of iron (40,41). Our study showed that ACSL4 and TFR-1 levels were increased in the placentas of rats treated with L-NAME while GPx4 and SLC7A11 levels were decreased. Furthermore, DFO-late treatment showed increased GPx4 and SLC7A11 levels but reduced protein levels of ACSL4 and TFR-1. However, ID-late treatment showed the opposite results, suggesting that intervention with DFO in later pregnancy reversed the PE placenta damage induced by L-NAME. Our data revealed that intervention with DFO in early pregnancy resulted in a decrease in ROS levels that led to insufficient uterine artery recasting, so PE features were more significant in the later stages of pregnancy. Early ID intervention also aggravated PE injury in later stages, but the degree was small and not significant. Using ID in later pregnancy significantly increased the level of ferroptosis in placental tissues. This preliminary study investigated the role of ferritin and ferroptosis on pregnant rats in the early and later stages of pregnancy, but whether these results are consistent with human research remains to be seen.
Conclusions
In this study, we demonstrated for the first time the relationship between ferritin concentrations, ferroptosis, and PE progression. Different concentrations of ferritin affected the histopathology status, oxidative stress, inflammatory response, apoptosis, and ferroptosis in pregnant rats with PE during different stages of pregnancy, indicating that high iron status and ferroptosis in later pregnancy may seriously affect the development and progression of PE.
Acknowledgments
Funding: This work was funded by the Zhejiang Traditional Chinese Medicine Foundation, China (grant No. 2016ZA133).
Footnote
Reporting Checklist: The authors have completed the ARRIVE reporting checklist. Available at https://atm.amegroups.com/article/view/10.21037/atm-22-3675/rc
Data Sharing Statement: Available at https://atm.amegroups.com/article/view/10.21037/atm-22-3675/dss
Conflicts of Interest: All authors have completed the ICMJE uniform disclosure form (available at https://atm.amegroups.com/article/view/10.21037/atm-22-3675/coif). The authors have no conflicts of interest to declare.
Ethics Statement: The authors are accountable for all aspects of the work in ensuring that questions related to the accuracy or integrity of any part of the work are appropriately investigated and resolved.
Open Access Statement: This is an Open Access article distributed in accordance with the Creative Commons Attribution-NonCommercial-NoDerivs 4.0 International License (CC BY-NC-ND 4.0), which permits the non-commercial replication and distribution of the article with the strict proviso that no changes or edits are made and the original work is properly cited (including links to both the formal publication through the relevant DOI and the license). See: https://creativecommons.org/licenses/by-nc-nd/4.0/.
References
- Nirupama R, Divyashree S, Janhavi P, et al. Preeclampsia: Pathophysiology and management. J Gynecol Obstet Hum Reprod 2021;50:101975. [Crossref] [PubMed]
- Mol BWJ, Roberts CT, Thangaratinam S, et al. Pre-eclampsia. Lancet 2016;387:999-1011. [Crossref] [PubMed]
- Matsubara K, Matsubara Y, Uchikura Y, et al. Pathophysiology of Preeclampsia: The Role of Exosomes. Int J Mol Sci 2021;22:2572. [Crossref] [PubMed]
- Opichka MA, Rappelt MW, Gutterman DD, et al. Vascular Dysfunction in Preeclampsia. Cells 2021;10:3055. [Crossref] [PubMed]
- Gong RQ, Nuh AM, Cao HS, et al. Roles of exosomes-derived lncRNAs in preeclampsia. Eur J Obstet Gynecol Reprod Biol 2021;263:132-8. [Crossref] [PubMed]
- Wedn AM, El-Bassossy HM, Eid AH, et al. Modulation of preeclampsia by the cholinergic anti-inflammatory pathway: Therapeutic perspectives. Biochem Pharmacol 2021;192:114703. [Crossref] [PubMed]
- Al-Kuraishy HM, Al-Gareeb AI, Al-Maiahy TJ. Concept and connotation of oxidative stress in preeclampsia. J Lab Physicians 2018;10:276-82. [Crossref] [PubMed]
- Wang Y, Walsh SW, Kay HH. Placental lipid peroxides and thromboxane are increased and prostacyclin is decreased in women with preeclampsia. Am J Obstet Gynecol 1992;167:946-9. [Crossref] [PubMed]
- Ni S, Yuan Y, Kuang Y, et al. Iron Metabolism and Immune Regulation. Front Immunol 2022;13:816282. [Crossref] [PubMed]
- Igbinosa I, Berube C, Lyell DJ. Iron deficiency anemia in pregnancy. Curr Opin Obstet Gynecol 2022;34:69-76. [PubMed]
- Ng SW, Norwitz SG, Norwitz ER. The Impact of Iron Overload and Ferroptosis on Reproductive Disorders in Humans: Implications for Preeclampsia. Int J Mol Sci 2019;20:3283. [Crossref] [PubMed]
- Fisher AL, Nemeth E. Iron homeostasis during pregnancy. Am J Clin Nutr 2017;106:1567S-74S. [Crossref] [PubMed]
- Yang N, Wang Q, Ding B, et al. Expression profiles and functions of ferroptosis-related genes in the placental tissue samples of early- and late-onset preeclampsia patients. BMC Pregnancy Childbirth 2022;22:87. [Crossref] [PubMed]
- Dixon SJ, Lemberg KM, Lamprecht MR, et al. Ferroptosis: an iron-dependent form of nonapoptotic cell death. Cell 2012;149:1060-72. [Crossref] [PubMed]
- Tang D, Kang R, Berghe TV, et al. The molecular machinery of regulated cell death. Cell Res 2019;29:347-64. [Crossref] [PubMed]
- Marcondes FK, Bianchi FJ, Tanno AP. Determination of the estrous cycle phases of rats: some helpful considerations. Braz J Biol 2002;62:609-14. [Crossref] [PubMed]
- Khudiar KK. Studying the effective dose of polyphenols extracted from green tea in ameliorating the deleterious effect of iron overload in female rats: Khalisa K. Khudiar1 and Nabeel M. Naji2. The Iraqi Journal of Veterinary Medicine 2012;36:142-52. [Crossref]
- Li Y, Yang H, Ni W, et al. Effects of deferoxamine on blood-brain barrier disruption after subarachnoid hemorrhage. PLoS One 2017;12:e0172784. [Crossref] [PubMed]
- Cao JY, Dixon SJ. Mechanisms of ferroptosis. Cell Mol Life Sci 2016;73:2195-209. [Crossref] [PubMed]
- Bothwell TH. Iron requirements in pregnancy and strategies to meet them. Am J Clin Nutr 2000;72:257S-64S. [Crossref] [PubMed]
- Altamura S, Kessler R, Gröne HJ, et al. Resistance of ferroportin to hepcidin binding causes exocrine pancreatic failure and fatal iron overload. Cell Metab 2014;20:359-67. [Crossref] [PubMed]
- Anderson ER, Taylor M, Xue X, et al. Intestinal HIF2α promotes tissue-iron accumulation in disorders of iron overload with anemia. Proc Natl Acad Sci U S A 2013;110:E4922-30. [Crossref] [PubMed]
- Means RT. Iron Deficiency and Iron Deficiency Anemia: Implications and Impact in Pregnancy, Fetal Development, and Early Childhood Parameters. Nutrients 2020;12:447. [Crossref] [PubMed]
- Najafzadeh H, Jalali MR, Morovvati H, et al. Comparison of the prophylactic effect of silymarin and deferoxamine on iron overload-induced hepatotoxicity in rat. J Med Toxicol 2010;6:22-6. [Crossref] [PubMed]
- Velasquez J, Wray AA. Deferoxamine, in StatPearls. 2022, StatPearls Publishing Copyright © 2022, StatPearls Publishing LLC.: Treasure Island (FL).
- Abdul Y, Li W, Ward R, et al. Deferoxamine Treatment Prevents Post-Stroke Vasoregression and Neurovascular Unit Remodeling Leading to Improved Functional Outcomes in Type 2 Male Diabetic Rats: Role of Endothelial Ferroptosis. Transl Stroke Res 2021;12:615-30. [Crossref] [PubMed]
- Nematbakhsh M, Pezeshki Z, Moaeidi BA, et al. Protective Role of Silymarin and Deferoxamine Against Iron Dextran-induced Renal Iron Deposition in Male Rats. Int J Prev Med 2013;4:286-92. [PubMed]
- Simavli S, Derbent AU, Keskin EA, et al. Do the first, second and third trimester maternal serum hepcidin concentrations clarify obstetric complications? J Matern Fetal Neonatal Med 2015;28:854-7. [Crossref] [PubMed]
- Sammallahti S, Tiemeier H, Reiss IKM, et al. Maternal early-pregnancy ferritin and offspring neurodevelopment: A prospective cohort study from gestation to school age. Paediatr Perinat Epidemiol 2022;36:425-34. [Crossref] [PubMed]
- Rogozińska E, Daru J, Nicolaides M, et al. Iron preparations for women of reproductive age with iron deficiency anaemia in pregnancy (FRIDA): a systematic review and network meta-analysis. Lancet Haematol 2021;8:e503-12. [Crossref] [PubMed]
- D'Souza V, Rani A, Patil V, et al. Increased oxidative stress from early pregnancy in women who develop preeclampsia. Clin Exp Hypertens 2016;38:225-32. [Crossref] [PubMed]
- Amaral TAS, Ognibene DT, Carvalho LCRM, et al. Differential responses of mesenteric arterial bed to vasoactive substances in L-NAME-induced preeclampsia: Role of oxidative stress and endothelial dysfunction. Clin Exp Hypertens 2018;40:126-35. [Crossref] [PubMed]
- Tayal D, Goswami B, Patra SK, et al. Association of inflammatory cytokines, lipid peroxidation end products and nitric oxide with the clinical severity and fetal outcome in preeclampsia in Indian women. Indian J Clin Biochem 2014;29:139-44. [Crossref] [PubMed]
- Talukdar RK, Das SK, Dokania I. A comparative study of cord blood haemoglobin and ferritin in normotensive versus preeclamptic patients. The New Indian Journal of OBGYN 2021;8:23-6. [Crossref]
- Singh L, Thakur A, Khan FM, et al. Prophylactic Iron Supplementation in Pregnancy and its Implications in Development of Preeclampsia: A Case Control Study. Journal of Clinical and Diagnostic Research 2021;15:BC22-B26.
- Zhang H, He Y, Wang JX, et al. miR-30-5p-mediated ferroptosis of trophoblasts is implicated in the pathogenesis of preeclampsia. Redox Biol 2020;29:101402. [Crossref] [PubMed]
- Imai H, Matsuoka M, Kumagai T, et al. Lipid Peroxidation-Dependent Cell Death Regulated by GPx4 and Ferroptosis. Curr Top Microbiol Immunol 2017;403:143-70. [Crossref] [PubMed]
- Chen D, Fan Z, Rauh M, et al. ATF4 promotes angiogenesis and neuronal cell death and confers ferroptosis in a xCT-dependent manner. Oncogene 2017;36:5593-608. [Crossref] [PubMed]
- Zhang Y, Zhuang L, Gan B. BAP1 suppresses tumor development by inducing ferroptosis upon SLC7A11 repression. Mol Cell Oncol 2018;6:1536845. [Crossref] [PubMed]
- Li Y, Feng D, Wang Z, et al. Ischemia-induced ACSL4 activation contributes to ferroptosis-mediated tissue injury in intestinal ischemia/reperfusion. Cell Death Differ 2019;26:2284-99. [Crossref] [PubMed]
- Dongiovanni P, Lanti C, Gatti S, et al. High fat diet subverts hepatocellular iron uptake determining dysmetabolic iron overload. PLoS One 2015;10:e0116855. [Crossref] [PubMed]
(English Language Editor: D. Fitzgerald)